Differentiation of quantities in animals
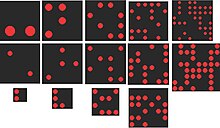
The differentiation of quantities in animals ( numerosity ) and the generalization of numbers ( number estimation ) has been proven in numerous, mutually independent behavioral experiments. In particular, some in Japan and in the USA with chimpanzees - the closest relatives of humans - conducted studies suggest that simple mathematical skills are not limited to humans. Evidence that animals of different species are capable of numbers (and some of them even numbers ) to distinguish could if one day should adequately be many studies that give an indication of how the ability to Expect in the course of evolutionary history of the species has developed.
Mental arithmetic and the use of complex mathematical formulas are cultural achievements and probably cannot do without the ability to use a language. A feeling for more or less and the ability to estimate numbers, however, are not linked to language (“ number sense ”). The differentiation of quantities - in addition to the perception of space and time - is likely to be one of the most elementary prerequisites for animals to be able to react appropriately to their environment when foraging, for example.
Experiments with infants show that babies as early as three months old can distinguish between different amounts. However, "these findings about the first abilities of children to distinguish between sets [...] hardly allow any conclusions to be drawn as to the extent to which one can speak of an understanding of sets in the mathematical sense."
First studies
Investigations into the "recording of relations" or the "understanding of numbers" of animals were already carried out at the beginning of the 20th century - before the establishment of the academic subject of animal psychology - and at that time formed the bridge to human psychology . The result was "an extensive literature on this phenomenon": In 1913 alone, more than 500 reports were published, reported the Munich physiologist Otto Frank in 1914 in the German Medical Weekly . At the same time, Frank published precise suggestions on how one could expose the alleged mathematical abilities of animals as self-deception by their owners with the help of carefully carried out tests. Frank attributed the lack of such tests to the fact that "psychology is still in its first development and one would like to see a certain guideline available for assessing the intellectual performance of animals."
"Smart" dogs and horses
In his review published in 1914, Otto Frank also stated, using the example of the dog Rolf from Mannheim: “It is not the scholar, but an experienced circus director or a skilled detective who seems to be primarily called to enlightenment. The scientifically interesting thing lies more in the psychology of the acting persons. ”A very extensive behavior analysis of the“ thinking dog Rolf von Mannheim ”was published in August 1916 in the Munich Medical Weekly . That Rolf was previously - also in the opinion of "a larger number of important psychologists" - as capable of communicating with people with the help of a "knock alphabet" (a kind of Morse code ). Allegedly the dog could do arithmetic and read, dictate letters and poems, and even write his autobiography . In fact, according to the behavioral analysis, it was the facial expressions of the dog's owner that controlled the dog's tapping.
Because of such supposed miracle animals, the newly emerging animal psychology had to struggle for the rank of a serious science in the 1920s and 1930s, since in the eyes of the academics its animal experiments and training were to a certain extent in competition with pseudoscientific carnival presentations . Bernhard Hassenstein wrote in 1974 in his obituary for Otto Koehler :
- “The so-called clever animals caused a special sensation: the Elberfeld horses, as well as Rolf, Lumpi, Fips, Kurwenal, Isolde and - until 1938 - around 80 other dogs, which apparently understood, calculated, took root and spelled every human word. When asked by a professor of theology: What is your worldview? replied the dachshund Kurwenal: Mine is yours! It has been proven several times that these miracle animals only knocked or barked until their owners, mostly unconsciously, gave them a sign to stop, i.e. dictated their own answers. All the more resolutely the injured stood up for their darlings, and even a professor of zoology discussed with conviction 'The number-speaking dogs as a domestication phenomenon ...' "

At that time the clever Hans , a horse that was said to be able to count, made it particularly well known . However, it turned out that the animal was only highly sensitive to facial expressions and postures of people.
In 2008, however, researchers at the University of Essex demonstrated that horses can actually differentiate between quantities: In a choice experiment between, for example, 6 and 4 apples (as well as 2 versus 1, 3 versus 2), the horses each decided on the larger number. According to a study published in 2013, dogs, on the other hand, generally do not prefer the larger portion of the food presented in such selective trials; however, according to an older study, at least individual test animals were able to do this. Furthermore, feral domestic dogs seem to be able to estimate the size of an 'enemy' pack of dogs. For wolves it was again proven by Austrian researchers that they differentiate between the size of the amount of food they eat (for example 1: 3) and that they prefer the larger amount of food in the selective experiment; from this it was concluded that dogs lost this ability in the course of domestication.
The observations on horses and other "clever" animals ultimately led to "a revolution in behavioral research: in studies of the cognitive abilities of animals, all eye or face contact must be strictly avoided."
"Counting attempts" with birds
Werner Fischel , from 1941 the first lecturer in animal psychology in Germany, published his doctoral thesis, which he wrote under Otto Koehler at the University of Munich , in 1926 under the title: “Do birds have a 'memory of numbers'?” Otto Koehler and his colleagues were the first to study numerous Publications specifically documented “counting attempts” of birds, which Koehler repeatedly examined using precise scientific methods. For example, pigeons and budgies learned to pick up either 2 or 4 baits, depending on the different colored instructions. Koehler taught a tamed raven from several vessels to always choose the one with five points on the lid, with the shape, size and position of the points being changed from experiment to experiment. The African gray parrot Jako reacted to acoustic and visual stimuli by, for example, removing 3 baits from the bowls after 3 flashes of light, and he also distinguished one from two sounds.
According to Otto Koehler, the differentiation of numbers always reached up to certain upper limits: in pigeons 5, in budgerigars and jackdaws 6, in Amazon parrots , magpies and common ravens 7, in African gray parrots 8. These data have been largely confirmed by other researchers. Since humans can also keep about 7 information units in their short-term memory at the same time (the so-called Miller's number ), Bernhard Hassenstein suspected in 1974 that the ability to differentiate numbers in humans and animals "comes from a common root", after all, under similar test conditions, " Humans do about the same as these animals ”.
Early experiments with rats
Otto Koehler's experiments with birds were neither reproduced nor continued by other research groups in the 1930s and 1940s. Above all in the USA, behavioral research was also characterized by behavioral research approaches that initially did not give rise to any interest in questions about innate, apparently cognitive performance of animals. Still, it was but then the derived just one of the pioneers of behaviorism, called programmed learning , the American psychologist Francis Mechner from Columbia University , the beginning of the 1960s developed a convincing proof method for distinguishing quantities, namely in rats .
Mechner ruled out any possible influence of the test director on the behavior of the test animals by using a so-called Skinner box . Hungry rats found two levers in this closed experimental apparatus that they could press with their snouts or paws. If lever 2 was pressed, an automat released some feed - but only if lever 1 had also been pressed beforehand. The number of required lever pressures on lever 1 was varied in different experimental approaches: Some animals only received their food reward if, for example, they pressed lever 1 four times and then lever 2, other animals had to press lever 1 eight times and then only lever 2 to get some food to obtain.
After some training, the test animals actually pressed lever 1 four or eight times on average and only then lever 2; They could also be taught to press the lever 12 and 16 times, although not every test animal always pressed the exact number specified by the test leader. Around 75 percent of the group of 4 pressed the lever three to six times, in the group of eight around 75 percent of the test animals pressed the lever seven to eleven times. From this one can deduce that rats can only learn a certain required number of actions relatively roughly. In order to rule out that the test animals learned to press the lever for a certain period of time instead of the number of lever presses, rats with different degrees of hunger were tested: the hungrier the animals were, the more hectically they pressed the lever, but this did not affect the number of lever presses.
Other rats were trained in a tunnel system to take the fourth turn to the left, regardless of the distance between the turns.
Two Brown University researchers , Russell Church and Warren Meck, published a study in 1984 that suggests that rats cannot just learn to do a certain number of lever pushes in a given situation. Rather, they can transfer what they have learned to a new situation; In human terms, one could say: The animals generalize the behavior they have learned. The researchers first taught the animals to press the left lever after two beeps and the right after four beeps. Then the animals also learned to press the left lever after two flashes of light and the right one after four flashes of light. Finally, during some tests, rats were presented with both sounds and flashes of light, and the rats also depressed the left lever when presented with a flash of light plus one tone and the right when presented with two flashes of light plus two tones.
Invertebrates
Honey bees
Honey bees ( Apis mellifera ) are known to orient themselves to landmarks when they repeatedly approach high-yielding forage plants. Researchers at the Australian National University trained bees to fly into a tunnel in which yellow lines or dots were used to mark a certain number of feeding places. The test bees learned to fly to a specific feeding point (at the first, second or third etc. marking), whereby the distances between the markings and the shape of the markings were variable. The actual test consisted in the trained bees flying into the tunnel without food being available at the usual feeding place. The tests showed that bees can remember the number of landmarks to fly over - regardless of their shape and their distance from one another - but not more than four landmarks.
A joint experiment by researchers from the Australian National University and Jürgen Tautz's working group in Würzburg showed that honeybees can distinguish numbers of up to four symbols, but not larger numbers such as four against five or four against six symbols. The bees first learned that a reward (sugar water) was hidden behind a board with two blue dots on it. The test animals conditioned in this way were then simultaneously given a board with two symbols and another board with, for example, four symbols to choose from: The trained animals each flew to the board with the two symbols. In further experiments the bees could be conditioned to quantities of up to four symbols. In addition, test animals that were conditioned to a certain quantity of blue dots, for example panels with yellow stars or green leaves, were given a choice: even under such changed conditions, the ability to distinguish the previously learned quantity was retained. The authors explained that their study was the first to demonstrate the ability to distinguish quantities in insects .
In 2018, Science reported that bees can also master the “concept zero ”. The test animals were first presented with two images with black objects on a white background, and they were rewarded with food if they approached the image with the smaller number of black objects. After training, they were presented with a pure white picture and one with two or three black objects for the first time: In 64 percent of all test flights - that is, overly often - the "empty" picture was flown to.
be crazy
The preying of young jumping spiders of the species Portia africana , a behavior that is directed against nests of the species Oecobius amboseli (Oecobiidae), was analyzed in an extensive laboratory study. It was already known that two Portia africana juveniles often eat together in the net of the prey. The experimental set-ups showed that a test animal of Portia africana is most likely to penetrate a nest of the prey species when exactly one individual of its own species is sitting there. Portia africana visits the nest statistically significantly less often if there are already two or three individuals of its own species or if no individual of its own species is present in the nest.
fishes
Gambus (mosquito fish) of the species Gambusia holbrooki form swarms whenever possible , with individual animals swimming to the largest of several swarms. Psychologists from the University of Padua used this behavior to test how big the difference between two swarms has to be in order to be distinguished from a single animal. They demonstrated that fish kept in the laboratory can differentiate between schools of 3 individuals and schools of 4 individuals, but not a ratio of 4: 5. A ratio of 2: 4, 4: 8 and 8:16 also proved to be distinguishable. Another study found that these animals can also distinguish small numbers of abstract symbols (2: 3).
Amphibians
Also Salamander the kind Plethodon cinereus , so amphibians , different size numbers may differ. This is the result of a study that a research group led by Claudia Uller from the University of Louisiana at Lafayette published in 2003 in the journal Animal Cognition . The test animals were presented with a different number of fruit flies as food in two glass tubes at the same time, for example one fly in one tube and two flies in the other tube. Without prior training, the test animals were able to differentiate between these different amounts of food and to control the tube with the larger number of flies. They were able to distinguish both the ratio of 1: 2 and 2: 3, but not the ratio of 3: 4 and 4: 6.
The researchers interpreted the results of their work as an expression of a widespread tendency in the animal kingdom to go for the larger amount of food. This tendency is innate because it occurs without practice and at least presupposes that a larger amount of food can be distinguished from a smaller one. In the case of small quantities, however, this ability to distinguish is not based on mere appraisal, but on precise differentiation between the differences. Since the ratio 2: 3 is differentiated, but not the ratio 4: 6, the researchers assume that the exact "number" of objects (2 or 3) actually influenced the behavior of the animals and not just the quantitative ratio of the feed animals in the two glass tubes. In the case of salamanders, the reliably distinguishable number of objects seems to be a maximum of 3.
Birds
chick
Chicks of domestic hens do not have an innate image of their conspecifics when they hatch from the egg; rather , they only learn to recognize them immediately after hatching - through imprinting . In the experiment, chicks can therefore also be imprinted on people or on inanimate objects. In addition, whenever possible, chicks join the largest of several groups of their conspecifics. Researchers at the University of Padua used both facts to test the counting ability of newly hatched, largely inexperienced chicks. They first stamped the chicks on small balls and then placed the animals on a pedestal , from which they could see two groups of these balls. The balls were then placed behind a screen so that the chicks could no longer see them. Then - visible to the chicks - individual balls were placed from one hiding place to the other. This experimental set-up was intended to clarify whether the chicks count where the greatest number of their “conspecifics” hid after the relocation. In fact, after such repositioning, the chicks sought the larger group of balls. Without any prior learning, the chicks could decide that was smaller than , that was larger than, and that was larger than . Accordingly, the ability to add and subtract seems to be an innate quality in them.
Pigeons
It is also known from experiments on pigeons that they differentiate between small quantities more precisely than large ones. The Canadian researcher William Roberts therefore analyzed an analogous form of stimulus processing: behavior depending on the duration of a stimulus. He trained pigeons to peck against a red lever when a light source shines briefly (for example for a second). But if the light source shone for a long time (for example 16 seconds), they had to pick against a green lever. One could have expected that with an average light duration of 8 or 9 seconds, the switch would go from short to long (i.e. from the red to the green lever) or that the test animals would be confused and only peck at red and green at random. In fact, the change happened at 4 seconds. It was also observed that the animals could distinguish a light duration of 1 to 4 seconds better than a light duration of 13 to 16 seconds, while they could distinguish 9 to 10 seconds better than 7 to 8 seconds. The researcher interpreted these findings to the effect that a period of time in the pigeon's brain is not processed uniformly (linearly), but rather logarithmically, so to speak . If the pigeons were to process time intervals linearly, they would have to be able to differentiate between 1 and 4 second intervals with equal accuracy. With a logarithm-like stimulus processing, however, a 13:16 interval would appear smaller than a 1: 4 interval, which would explain the inaccuracy observed when differentiating the 13:16 interval compared to the 1: 4 interval.
In another study, pigeons were shown a different number of differently shaped symbols on a screen, for example 4 yellow ovals, 8 green squares, 5 blue dots. Two different numbers and different symbols were projected at the same time. With an average accuracy of 80 percent, the pigeons learned to first indicate the smaller number - regardless of their shape - by pecking and then the larger number.
Long-leg flycatcher
According to a study by researchers at Victoria University , free-living New Zealand long-legged flycatchers ( Petroica australis ) can distinguish numbers such as 1 versus 2, 2 versus 3, and 4 versus 6. Researchers from Simon Hunt's group had tested 14 long-legged flycatchers in the wild. In each test, two quantities of worms of different sizes were placed in two vessels and then presented to the birds to eat. The birds could always observe the number of worms placed in the respective test vessel. The birds then went to the container with the larger amount of food with a high degree of accuracy: in the alternative 1 worm versus 2 worms, the 2 worms were first eaten in almost 90 percent of the tests. With the alternatives 2 against 3, 3 against 4 and 4 against 8, the hit rate was 80 percent. Only with higher combinations (such as 6 against 8) did the hit rate approach the random value of 50 percent.
In a second test, certain different numbers of worms were placed in the test vessels, but some of them disappeared from the vessel through a trap door. It was then shown again that the birds initially approached the vessel with the initially larger number of worms. They stayed at this vessel, for example, four times as long if initially 2 worms had been in it, but the birds only found one, than if only 1 worm had been placed in it from the start. The researchers concluded that the animals actually counted and expected a certain number of worms.
African gray parrots
The American scientist Irene Pepperberg has been studying the ability of African gray parrots to distinguish between different quantities for more than 25 years . Her African gray parrot Alex (1976-2007) learned, among other things, to correctly designate 50 objects presented to him using a special utterance, plus seven colors and five shapes.
According to her, Alex could also do simple additions and count to six. In an experiment in which two, three and six different colored objects were in front of him and he was asked what color five (same colored) objects were, Alex replied: None . From this, the researcher concluded that Alex had a zero-like concept and also emphasized that zero and nothing are by no means identical.
Fox hummingbirds
The fox hummingbird native to North America is diurnal, it feeds on the nectar of the flowers and - coming as a migratory bird from its winter quarters in Mexico - it also colonizes the valleys of the Rocky Mountains during the warm season . In a field experiment in 2017, nine birds were tagged shortly after their arrival in the Westcastle Valley ( Alberta , Canada ) after they had visited a bright yellow “artificial flower” and consumed the sugar solution it offered. The test arrangement consisted of ten similarly lined up, 60 centimeter high wooden sticks, on each of which a “blossom” was attached. Initially, only the first flower in the row was prepared with sugar solution at night, which - unsurprisingly - was immediately approached on the following days. After this training phase, a different, randomly selected “flower” was prepared with a sugar solution: the marked birds initially flew to the first flower in the row. So they had learned the position of the food source and not just based on the smell of the sugar solution. In further test arrangements, the second, third or fourth “flower” in the row of ten was initially prepared several times with sugar solution and then left without this food supply: In this case, too, the birds first flew to the previously prepared “flower”; they are consequently able to identify the position of an object in a series of similar objects using numerical criteria.
Mammals
Raccoons
Stanislas Dehaene reports in his book The Sense of Numbers about an experiment in which raccoons learned to take raisins from a transparent box - always from the box that contained three raisins and not from one of the neighboring boxes that held two or four raisins lay.
American black bear
Three black bears were trained in front of a touchscreen to differentiate between different numbers of moving or immovable points. All three animals succeeded in doing this.
Asian elephants
The fourteen-year-old Asian elephant Authai from Ueno Zoo was shown two different numbers of bananas, apples or watermelons on a touchscreen that he could operate with the tip of his trunk, and he was rewarded with treats for each larger one Number indicated. These fruits (0 to 10) were never shown the same size to ensure that the choice could not be made based on the area covered. In 181 of 271 elections (= 66.8%) the larger number was correctly displayed. It was irrelevant how large the distance between the numbers shown was, but the time interval between the start of a test and the answer was longer, the smaller the distance between the numbers shown. This study, published in 2018, confirmed an earlier research from 2009, in which it had been shown that Asian elephants can distinguish between different amounts of up to six objects.
Asian elephants can also differentiate between a larger and a smaller amount of food based on smell alone.
Rhesus monkeys
In 1998, Elizabeth M. Brannon and Herbert S. Terrace demonstrated in a much-cited study of rhesus monkeys that they can differentiate between larger and smaller quantities. Comparable findings on the behavior of the Anubis baboons were published in 2013.
Andreas Nieder's Primate Neurocognition Working Group (Hertie Institute for Clinical Brain Research at the University of Tübingen ) investigated non-linguistic precursors of numerical competence in rhesus monkeys. In a test, his team trained two rhesus monkeys to distinguish certain numbers of points that were shown to them on a computer screen. For example, the animals were shown a circle with four points and, after a break, another circle with either four or three or five points. If the second number shown was the same as the first, the monkey released a lever and received a reward. If the number of points was different, the test animal continued to hold the lever down until it was presented with the same number of points.
At the same time, with the help of implanted microelectrodes, the researchers registered the activity of individual nerve cells in certain brain areas of the test animals in which numerical information is processed: In the intraparietal sulcus - a parietal lobe of the cerebral cortex - and around the prefrontal cortex , an area of the frontal lobe . Nieder's team found out that numerical information is initially processed in the intraparietal sulcus and "presumably passed on to the prefrontal cortex", where it is amplified and retained in short-term memory and is thus available for behavioral control. In this way, it was also possible to prove that individual nerve cells are 'calibrated' to process certain quantities: they fire particularly intensely when the animal is presented with 'their' quantity. Certain neurons therefore have a certain 'favorite set'.
In another study it was shown that 20 percent of the neurons located in the prefrontal cortex of rhesus monkeys are active when the test animals are stimulated to differentiate between quantities. The researchers had trained two monkeys to move a lever when the number of points on an image was larger than one previously shown or when it was smaller than one previously shown. In the prefrontal cortex, different groups of neurons were active, depending on whether a number shown was smaller or larger than a previously shown number.
In December 2007, two researchers from Duke University reported that female rhesus monkeys and students can solve simple addition problems with comparable reliability. The test subjects were shown groups of points on a touchscreen , for example five points for half a second, three points after a short break and two boxes with eight or four points after a short break. If the correct box was tapped, the two test animals were given fruit juice as a reward, the twelve students were rewarded as a lump sum. A total of 40 such addition tasks had to be solved by each participant. The humans solved 95 percent of the tasks, the monkeys 75 percent. Errors were most likely to arise when the two solutions offered were very close to each other, i.e. consisted of eleven or twelve points, respectively.
Chimpanzees
David Premack and Guy Woodruff published a study in Nature in 1981 that suggested that chimpanzees can operate on fractions of quantities. For example, the test animals were shown a half-full glass and then had to point to another half-full glass rather than one three-quarters full. After the animals had learned this, they were shown a half-full glass, followed by half an apple and a three-quarter apple. Although apples and glasses are completely different looking objects, the test animals pointed to half the apple; In human terms, one could say: the chimpanzees knew that half a cake is to a whole cake like a half-filled glass is to a whole glass. They were able to distinguish a quarter and three quarters with similar success. If the animals were shown a half-full glass and a quarter apple at the same time in a further experiment, a three-quarter circle was then indicated more often than a whole circle.
In 1988, the chimpanzee Sheba was trained in dealing with quantities and numbers by Sally Boysen at the Ohio State University Chimpanzee Center . She was the first animal that could be shown to understand the meaning of zero . She masters the numbers up to 8 and has spontaneously carried out additions in this number range. After Sheba, other chimpanzees at Ohio State University were made familiar with counting and naming numbers in a similar way. This was done by teaching the animals, for example, to first collect a certain number of oranges and then to point to the number that corresponded to the number of oranges - for example, to point to the number 4 after collecting four oranges. Sheba is also the only animal known to date that was able to add numbers purely symbolically: If she was shown the number 2 on one picture and the number 4 on another, she was able to move towards the number 6 from the first attempt interpret. In early 2006, the Ohio State University Chimpanzee Center , founded in 1983 by Sally Boysen, was closed due to lack of funds and the animals were housed in a primate center in Texas .
At the Primate Research Institute of the University of Kyoto , tests were also carried out with several chimpanzees, which produced comparable results: The chimpanzee "Ayumu" and five other animals can number the numbers from 1 to 9 in ascending order and in the correct order with their fingers and one of the animals named "Ai" can do this from 0 to 9.
However, this success was only achieved after years of training. Ai had first learned the meaning of the Arabic numeral 1. When the number 2 was also introduced, it turned out that 2 was initially used by her in the sense of more than 1 . After she was able to safely use the Arabic number 2, the number 3 was included in the training program: The number 3 was also initially used by the animal in the sense of more than 2 . Every single number up to 9 had to be learned in this way in long training phases.
This learning behavior is comparable to that of human children around 30 months old. Five-year-old children, on the other hand, already have a sufficiently large capacity for abstraction that enables them to creatively use even very large numbers that lie outside their normal world of experience.
Biological and social foundations in humans

It is unknown whether the ability to differentiate between quantities developed several times over the course of the tribal history independently of one another (i.e. convergent ) or whether the common ancestors of bees, birds and humans were already able to do this. Nothing is known about the numerical understanding or even the mathematical abilities of the pre-humans and the early, non-written cultures. The earliest evidence of human evidence is the Ishango bone, as well as records from the Sumerians and ancient Egyptians . Among other things, they developed systems for dealing with large numbers, for example for stock management.
However, it is certain that the ability to deal with numbers and numbers in humans is based on certain innate properties of the brain, and a. specifically of the visual cortex . If the areas of the brain that are responsible for this are disturbed, for example by an injury, this can lead to the clinical picture of dyscalculia . US researchers demonstrated a connection between estimating numbers and solving math problems in children aged 5 to 14.
Studies in infants and young children
A study by French psychologists on 80 to three-day-old newborns who were able to distinguish small from large amounts speaks in favor of an innate recognition of quantities in humans. American psychologists reported comparable findings after experiments with 48 children who had been tested at the age of six months and again at the age of 3½ years. Even the infants focused their attention more on a screen on which the number of points appeared in a different arrangement and also constantly changing between 10 and 20 than on a second screen on which exactly 10 points always appeared in a changing arrangement. In addition, those children who looked particularly conspicuously at the first screen at the age of six months also showed a particularly good ability to differentiate between different numbers at the age of 3½ years. For six-month-old infants it has been proven that they can differentiate 1: 2, but not 2: 3, elements; 10-month-old infants manage 8:12 (i.e. 2: 3), but not 8:10 (i.e. 4: 5 ) To distinguish elements.
French researchers reported comparable findings in a study. They registered the brain waves of 36 three-month-old babies while the babies were shown images on a screen. In the pictures, different objects were shown alternately, but in each individual picture the same objects and usually a certain number of them, for example four ducks; occasionally, however, a different number was projected. In this way, it was possible to prove that a deviation from the usual number of projected objects resulted in a change in the activities in a certain brain region, and in a different region than was the case when the objects depicted were changed while maintaining their number .
Another study on infants suggests that the perception of quantities of different sizes and the ability to calculate in the brain are closely linked. The researchers at Ben Gurion University in the Negev initially showed the same number of dolls (either one or two) several times on a screen for babies between six and nine months . Then they were shown one doll too many or too little. This deviation caused the infants to fixate the screen about a second longer than before. For the researchers, this was an indication that the infants had perceived the different numbers. Michael Posner had already carried out such experiments 15 years earlier with the same result, but his interpretations had been questioned again and again. Therefore, this time, in addition to observing the babies' eyes, his team had created a special measuring system with 128 electrodes to record the brain waves. As the researchers reported, the brain wave measurements showed clear parallels to measurements of the adult brain during arithmetic.
A study with preschool children who had not yet had a math class also showed evidence of a positive correlation between correctly estimating numbers and advanced math skills. In addition, the clinical picture of Gerstmann's syndrome indicates that there is a close neuropsychological connection between understanding numbers and difficulties in naming and identifying one's own fingers; It is possible that counting began with the help of the fingers - from a phylogenetic point of view, which in turn would explain the widespread system of 10 .
Adult studies
If adults - without counting - should name the number of objects, numbers greater than 4 are increasingly incorrectly recognized; This was first reported in the journal Nature in 1871 . These observations are consistent with early written records from the ancient Greek city of Karystos as well as the Cretans , Hittites , Phoenicians and from the China of the Yin dynasty , in which only the numbers 1 to 4 are represented by vertical (Mediterranean) or horizontal lines (China). shown, for the numbers 5 and larger, however, different characters were used.
In 2008, Stanislas Dehaene reported on investigations into the Munduruku , an indigenous people in the Brazilian Amazon region . The Munduruku do not attend schools and only know words for the numbers one through five; larger quantities are generally referred to as “some” or “many”. Dehaene asked his test subjects to assign different numbers of points - between 1 and 10 points each, in a second test between 10 and 100 points - to a position on a straight line. While European test persons placed 5 or 50 points very precisely in the middle of the straight line, the 5 and 50 points were always placed closer to 10 and 100 respectively by the indigenous test persons. Since a comparable “compression” of larger numbers was also demonstrated in European children, Dehaene concluded from his findings that the original intuitive allocation of the quantities was logarithmic . He described the concept of linear arrangement as a cultural achievement that does not develop in the absence of formal training.
A study of users of the Nicaraguan sign language also pointed to cultural influences when recognizing and naming large numbers . For quantities greater than three, these people became imprecise and, for example, showed nine fingers for the number “10”; In contrast, users of American Sign Language generally did not show such inaccuracies. Both groups live in a social environment in which dealing with large numbers and numbers is common.
A special case: the Venus flytrap
The Venus flytrap can register how often an insect touches its sensory hair. A single touch does not trigger the trap mechanism, but only a second touch. With five or more touches, the plant also activates the genes for digestive enzymes in its glands.
See also
literature
- Stanislas Dehaene: The sense of numbers or why we can calculate. Birkhäuser Verlag, Basel 1999, ISBN 3-7643-5960-9 .
(Original title: The Number Sense. Oxford University Press, 1997.) - Hans Joachim Gross: Can animals count? The magic number four and the innate understanding of numbers in humans and animals. In: Biology in Our Time. Vol. 42, No. 4, 2012, pp. 232-237, doi: 10.1002 / biuz.201210483 .
- Andreas Nieder: A Brain for Numbers: The Biology of the Number Instinct. The MIT Press, 2019, ISBN 978-0-262-04278-9 .
- Ute Seibt: Number concept and number behavior in animals. In: Zeitschrift für Tierpsychologie. Vol. 60, 1982, pp. 325-341.
Web links
- "Counting" animals. (Parrots, magpies, squirrels) Historical film documentation from Otto Koehler's laboratory
- Differentiation of quantities in the dog video of the BBC
Individual evidence
- ↑ Jamie D. Roitman, Elizabeth M. Brannon, and Michael L. Platt: Monotonic Coding of Numerosity in Macaque Lateral Intraparietal Area. In: PLoS Bio. Volume 5, No. 8, e208, 2017, doi: 10.1371 / journal.pbio.0050208
- ↑ "The ability to represent time and space and number is a precondition for having any experience whatsoever." According to the co-director of the Rutgers Center for Cognitive Science, Randy Gallistel , quoted in: Ewen Callaway: Animals that count. In: New Scientist, June 20, 2009, p. 37.
- ↑ Klaus Hasemann, Hedwig Gasteiger: Initial lessons in mathematics. 3. Edition. Springer Spectrum, Berlin and Heidelberg 2014, p. 2, ISBN 978-3-642-39312-9
- ↑ Dimitri Usnadze : On the problem of the recording of relations in animals. In: Archives for the whole of psychology. Volume 60, 1927, pp. 361-390.
- ↑ Otto Frank : The so-called thinking animals. In: Deutsche Medizinische Wochenschrift Volume 40, No. 24, 1914, pp. 1224-1226.
- ↑ Dr. Wilhelm Neumann: About the thinking dog Rolf von Mannheim. In: Münchner Medizinische Wochenschrift , Volume 31, 1916, p. 1226 f. (a detailed, critical analysis of Rolf's alleged intellectual abilities )
- ^ Journal of Animal Psychology , Volume 35, pp. 449 ff.
- ↑ Theodor Pintner : Some remarks about the so-called thinking animals. Lecture from March 17, 1915, full text (PDF) .
- ↑ A sound recording from 1912 of the "speaking dog Don" is preserved in the phonogram archive of the Ethnological Museum of the State Museums in Berlin.
- ^ Karl Krall: Thinking animals. Contributions to animal soul science based on own experiments. The clever Hans and my horses Muhamed and Zarif . Friedrich Engelmann, Leipzig 1912. Online at archive.org
- ↑ Claudia Uller, Jennifer Lewis: Horses (Equus caballus) select the greater of two quantities in small numerical contrasts. In: Animal Cognition , Volume 12, No. 5, 2009, pp. 733-738. doi: 10.1007 / s10071-009-0225-0
- ^ Krista Macpherson and William A. Roberts: Can dogs count? In: Learning and Motivation , Volume 44, No. 4, 2013, pp. 241-251. doi: 10.1016 / j.lmot.2013.04.002
- ^ Camille Ward and Barbara B. Smuts : Quantity-based judgments in the domestic dog (Canis lupus familiaris). In: Animal Cognition , Volume 10, No. 1, 2007, pp. 71-80. doi: 10.1007 / s10071-006-0042-7
- ^ Roberto Bonanni et al .: Free-ranging dogs assess the quantity of opponents in intergroup conflicts. In: Animal Cognition , Volume 14, No. 1, 2011, pp. 103-115. doi: 10.1007 / s10071-010-0348-3
- ↑ Ewelina Utrata et al .: Quantity discrimination in wolves (Canis lupus). In: Frontiers in Psychology , online publication November 16, 2012. doi: 10.3389 / fpsyg.2012.00505
-
^ Friederike Range et al .: Difference in quantity discrimination in dogs and wolves. In: Frontiers in Psychology , online publication of November 18, 2014. doi: 10.3389 / fpsyg.2014.01299
Much or little. Wolves are better at distinguishing between sets than dogs. On: idw-online from December 16, 2014. - ↑ Hans Joachim Gross: A forgotten revolution. The story of the clever horse Hans. In: Biology in our time , Volume 44, No. 4, 2014, pp. 268–272. doi: 10.1002 / biuz.201410544
- ↑ Werner Fischel: Do birds have a “number memory”? In: Journal of Comparative Physiology. Volume 4, No. 8, 1926, pp. 345-369
- ↑ Otto Koehler: “Counting” experiments on a common raven and comparative experiments on humans. In: Zeitschrift für Tierpsychologie. Volume 5, No. 3, 1943, pp. 575-712, doi: 10.1111 / j.1439-0310.1943.tb00665.x ; cf. also Stanislas Dehaene: The sense of numbers or why we can calculate. Birkhäuser Verlag, Basel 1999, p. 28. - Squirrels also learned to choose the only other one from several lids with the same number of points .
- ↑ Journal for Animal Psychology. Volume 35, p. 229 ff.
- ^ F. Mechner: Effects of deprivation upon counting and timing in rats. In: Journal of the Experimental Analysis of Behavior. Volume 5, 1962, pp. 463-466
- ↑ Dehaene, numbers sense, p. 29
- ↑ Russell M. Church and W. H. Meck: The numerical attribute of stimuli. In: H. L. Roitblat, T. G. Bever and H. S. Terrace (Eds.): Animal cognition. Erlbaum, Hillsdale, NJ, 1984, pp. 445-464
- ↑ Marie Dacke, Mandyam V. Srinivasan: Evidence for counting in insects. In: Animal Cognition. Volume 11, Number 4, 2008, pp. 1435-9448. doi: 10.1007 / s10071-008-0159-y
- ^ Hans J. Gross, Mario Pahl, Aung Si, Hong Zhu, Jürgen Tautz and Shaowu Zhang (2009): Number-Based Visual Generalization in the Honeybee. In: PLoS ONE . Volume 4, No. 1: e4263. doi: 10.1371 / journal.pone.0004263
-
^ Scarlett R. Howard: Numerical ordering of zero in honey bees. In: Science. Volume 360, No. 6393, 2018, pp. 1124–1126, doi: 10.1126 / science.aar4975
Bees understand the concept of zero. On: sciencemag.org June 7, 2018 - ↑ Ximena J. Nelson and Robert R. Jackson: The role of numerical competence in a specialized predatory strategy of an araneophagic spider. In: Animal Cognition. Volume 15, 2012, pp. 699-710, doi: 10.1007 / s10071-012-0498-6 , full text
- ↑ Christian Agrillo et al .: Do fish count? Spontaneous discrimination of quantity in female mosquitofish. In: Animal Cognition. Volume 11, Issue 3, July 2008, pp. 495–503, online at Springer.com, doi: 10.1007 / s10071-008-0140-9 , accessed on December 19, 2016.
- ↑ Christian Agrillo et al .: Quantity discrimination in female mosquito fish. In: Animal Cognition. Volume 10, Issue 1, January 2007, pp. 63–70, online at Springer.com, doi: 10.1007 / s10071-006-0036-5 , accessed on December 19, 2016.
- ↑ Christian Agrillo et al .: Use of Number by Fish. In: PLoS ONE. Volume 4, Issue 3: e4786, March 10, 2009, online at Plos.org, doi: 10.1371 / journal.pone.0004786 , accessed on December 19, 2016.
- ↑ Claudia Uller et al .: Salamanders (Plethodon cinereus) go for more: rudiments of number in an amphibian. In: Animal Cognition. Volume 6, Issue 2, June 2003, pp. 105-112, doi: 10.1007 / s10071-003-0167-x ; Full text ( memento of September 29, 2007 in the Internet Archive ), (PDF; 165 kB).
- ^ Rosa Rugani et al .: Arithmetic in newborn chicks. In: Proceedings of the Royal Society B. Volume 276, 2009, pp. 2451-2460, doi: 10.1098 / rspb.2009.0044 .
-
^ William A. Roberts: How do pigeons represent numbers? Studies of number scale bisection. In: Behavioral Processes. Volume 69, No. 1, 2005, pp. 33-43.
William A. Roberts: Evidence that pigeons represent both time and number on a logarithmic scale. In: Behavioral Processes. Volume 72, No. 3, 2006, pp. 207-214, doi: 10.1016 / j.beproc.2006.03.002 . - ^ Damian Scarf, Harlene Hayne, Michael Colombo: Pigeons on Par with Primates in Numerical Competence. In: Science . Volume 334, No. 6063, 2011, p. 1664, doi: 10.1126 / science.1213357 .
- ↑ Simon Hunt, Jason Low, K.C. Burns: Adaptive numerical competency in a food-hoarding songbird. In: Proceedings of the Royal Society B. Volume 275, No. 1649, 2008, pp. 2373-2379, doi: 10.1098 / rspb.2008.0702 .
- ↑ Süddeutsche Zeitung. No. 210 of September 12, 2007, p. 18.
- ↑ Marcus Anhäuser: Honor rescue for the sparrow brain. In: Süddeutsche Zeitung. Wissen, February 8, 2005, p. 9, online at Redaktion-Wissen.de, accessed on January 17, 2017.
- ↑ Alex: 1976-2007. From: The Alex Foundation, online at AlexFoundation.org, accessed January 17, 2017.
- ↑ Gardner: No average bird. ( Memento of September 3, 2006 in the Internet Archive ) In: Brandeis News. July 5, 2005, Brandeis University , accessed January 17, 2017.
-
^ Tas IF Vámos, Maria C. Tello-Ramos, T. Andrew Hurly, and Susan D. Healy: Numerical ordinality in a wild nectarivore. In: Proceedings of the Royal Society B. Volume 287, No. 1930, 2020, doi: 10.1098 / rspb.2020.1269 .
Hummingbirds can count their way to food. On: sciencemag.org July 7, 2020. - ↑ Stanislas Dehaene: The Sense of Numbers or Why We Can Calculate. Birkhäuser Verlag, Basel 1999, ISBN 978-3-0348-7826-5 .
- ↑ Jennifer Vonk, Michael J. Beran: Bears 'count' too: quantity estimation and comparison in black bears, Ursus americanus. PMC 3398692 (free full text), In: Animal Behavior. Volume 84, No. 1, Elsevier, July 1, 2012, pp. 231-238, online at NCBI, accessed on February 1, 2017, doi: 10.1016 / j.anbehav.2012.05.001 .
-
^ Naoko Irie, Mariko Hiraiwa-Hasegawa and Nobuyuki Kutsukake: Unique numerical competence of Asian elephants on the relative numerosity judgment task. In: Journal of Ethology. Online publication from October 22, 2018, doi: 10.1007 / s10164-018-0563-y
Asian elephants could be the maths kings of the jungle. On: eurekalert.org from October 22, 2018 - ^ Naoko Irie-Sugimoto, Tessei Kobayashi, Takao Sato and Toshikazu Hasegawa: Relative quantity judgment by Asian elephants (Elephas maximus). In: Animal Cognition: Volume 12, No. 1, 2009, pp. 193-199, doi: 10.1007 / s10071-008-0185-9
-
↑ Joshua M. Plotnik et al .: Elephants have a nose for quantity. In: PNAS. Online publication of June 3, 2019, doi: 10.1073 / pnas.1818284116
Watch an elephant 'count' simply by using its sense of smell. On: sciencemag.org from June 3, 2019 - ↑ Elizabeth M. Brannon, Herbert S. Terrace: Ordering of the Numerosities 1 to 9 by Monkeys. In: Science. Volume 282, No. 5389, October 23, 1998, pp. 746-749, doi: 10.1126 / science.282.5389.746 .
- ↑ Allison M. Barnard et al .: Inherently analog quantity representations in olive baboons (Papio anubis). In: Frontiers in Psychology. Volume 4, No. 253, May 2, 2013, doi: 10.3389 / fpsyg.2013.00253 .
- ^ Andreas Nieder, David J. Freedman and Earl K. Miller: Representation of the quantity of visual items in the primate prefrontal cortex. In: Science. Volume 297, No. 5587, September 6, 2002, pp. 1708-1711, doi: 10.1126 / science.1072493 .
- ↑ Press release of the Working Group of Scientific Medical Societies from July 10, 2006 on the occasion of a lecture by Andreas Nieder at the 2006 Forum of the Federation of European Neuroscience Societies (FENS) in Vienna.
- ^ Sylvia Bongard, Andreas Nieder: Basic mathematical rules are encoded by primate prefrontal cortex neurons. In: PNAS . Volume 107, No. 5, February 2, 2010, pp. 2277-2282, doi: 10.1073 / pnas.0909180107 .
- ↑ Jessica F. Cantlon, Elizabeth M. Brannon: Basic Math in Monkeys and College Students. In: PLoS Biol . Volume 5, No. 12: e328, December 18, 2007, doi: 10.1371 / journal.pbio.0050328 .
- ↑ Guy Woodruff, David Premack: Primative mathematical concepts in the chimpanzee: proportionality and numerosity. In: Nature. Volume 293, October 15, 1981, pp. 568-570, doi: 10.1038 / 293568a0 .
- ↑ Dehaene: number sense. P. 50.
- ^ Ohio State to close its Primate Center, retire its Chimpanzees. ( Memento of December 31, 2006 in the Internet Archive ) In: Research News. Ohio State University, online at ResearchNews.osu.edu, accessed February 1, 2017.
- ^ Website of the chimpanzee "Ai" in Kyoto, accessed on February 1, 2017.
- ↑ Ewen Callaway: Animals that count. In: New Scientist . June 20, 2009, p. 39.
- ^ Ewen Callaway: Animals that count: How numeracy evolved. Video, June 17, 2009, on NewsScientist.com, accessed February 1, 2017.
- ^ Elliot Collins et al .: Numerosity representation is encoded in human subcortex. In: PNAS. Volume 114, No. 14, 2017, E2806-E2815, doi: 10.1073 / pnas.1613982114 .
- ↑ Amandine Van Rinsveld et al .: The neural signature of numerosity by separating numerical and continuous magnitude extraction in visual cortex with frequency-tagged EEG. In: PNAS. Online advance publication of March 2, 2020, doi: 10.1073 / pnas.1917849117 .
- ↑ Justin Halberda, Michele MM Mazzocco, Lisa Feigenson: Individual differences in non-verbal number acuity correlate with maths achievement. In: Nature. Volume 455, October 2, 2008, pp. 665–668, doi: 10.1038 / nature07246 .
-
^ Maria Dolores de Hevia, Ludovica Veggiotti, Arlette Streri and Cory D. Bonn: At Birth, Humans Associate "Few" with Left and "Many" with Right. In: Current Biology. Volume 27, No. 24, 2017, pp. 3879-3884.e2, doi: 10.1016 / j.cub.2017.11.024 .
Newborn babies know their numbers. On: sciencemag.org December 7, 2017 - ↑ Ariel Starr et al .: Number sense in infancy predicts mathematical abilities in childhood. In: PNAS. Online pre-publication of October 21, 2013, doi: 10.1073 / pnas.1302751110 .
- ^ Fei Xu and Rosa I. Arriaga: Number discrimination in 10-month-old infants. In: The British Journal of Developmental Psychology. Volume 25, No. 1, 2007, pp. 103-108, doi: 10.1348 / 026151005X90704 .
- ^ Véronique Izard, Ghislaine Dehaene-Lambertz, Stanislas Dehaene: Distinct Cerebral Pathways for Object Identity and Number in Human Infants. In: PLoS Biology . Vol. 6, No. 2, e11, doi: 10.1371 / journal.pbio.0060011 .
- ↑ a b Andrea Berger, Gabriel Tzur, Michael I. Posner: Infant brains detect arithmetic errors. In: PNAS. Vol. 103, 2006, pp. 12649-12653, doi: 10.1073 / pnas.0605350103 .
-
↑ similar: Jinjing (Jenny) Wang and Lisa Feigenson: Infants recognize counting as numerically relevant. In: Developmental Science. Volume 22, No. 6, 2019, e12805, doi: 10.1111 / desc.12805 .
Babies understand counting years earlier than believed. On: eurekalert.org of October 24, 2019. - ↑ Melissa E. Libertus et al .: Preschool acuity of the approximate number system correlates with school math ability. In: Developmental Science. Vol. 14, No. 6, 2011, pp. 1292-1300, doi: 10.1111 / j.1467-7687.2011.01080.x .
- ^ A b Alfredo Ardila: On the evolution of calculation abilities. In: Frontiers in Evolutionary Neuroscience. June 23, 2010, doi: 10.3389 / fnevo.2010.00007 .
- ^ Hans J. Gross: Give me 5… The invention of number five in ancient civilizations. A consequence of our limited inborn numerical competence. In: Communicative & Integrative Biology. Vol. 4, No. 1, 2011, pp. 62–63, doi: 10.4161 / cib.4.1.13762 , full text (PDF; 289 kB) ( Memento from February 27, 2013 in the Internet Archive ).
- ^ W. Stanley Jevons: The Power of Numerical Discrimination. In: Nature. Vol. 3, No. 67, 1871, pp. 281-282, doi: 10.1038 / 003281a0 , full text .
- ↑ Georges Ifrah: Universal History of Numbers. Campus Verlag, Frankfurt am Main 1986, pp. 391–392, ISBN 3-88059-956-4 .
- ^ Stanislas Dehaene et al .: Log or Linear? Distinct Intuitions of the Number Scale in Western and Amazonian Indigenous Cultures. In: Science. Vol. 320, No. 5880, 2008, pp. 1217-1220, doi: 10.1126 / science.1156540 .
- ↑ literally: “This indicates that the mapping of numbers onto space is a universal intuition and that this initial intuition of number is logarithmic. The concept of a linear number line appears to be a cultural invention that fails to develop in the absence of formal education. "
- ↑ Elizabet Spaepen et al .: Number without a language model. In: PNAS. Vol. 108, No. 8, 2011, pp. 3163-3168, doi: 10.1073 / pnas.1015975108 .
- ↑ Jennifer Böhm et al .: The Venus Flytrap Dionaea muscipula Counts Prey-Induced Action Potentials to Induce Sodium Uptake. In: Current Biology. Vol. 26, No. 3, 2016, pp. 286-295, doi: 10.1016 / j.cub.2015.11.057 .
- ↑ Marco Bosch: Plants can count. In: Press and Public Relations. Informationsdienst Wissenschaft (IDW), January 22, 2016, from IDW-online.de, accessed on February 13, 2017.