Pyroclastic density flow
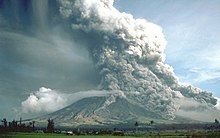
The term pyroclastic density current (engl. Pyroclastic density current ) is in the Vulkanologie or geology used as a generic term to refer to a gas (liquid) particles mixture, which arise in the explosive eruption of a volcano and flow through its density at the bottom can. It covers the entire spectrum from low-particle to high-particle mixtures, hot and relatively cool, e.g. Sometimes also water-containing streams that move at relatively low or high speeds, turbulent or through grain-grain contact. The term is more comprehensive than the older and more well-known, but also ambiguous term pyroclastic flow , which describes only part of the phenomenon and is now usually only used in a very narrow sense (pyroclastic flow in the past). Both terms describe a transport and deposition process in volcanology, not the deposition itself. Pyroclastic density flows can reach considerable speeds (up to more than 1000 km / h) and be very hot (up to around 800 ° C). The so-called glowing clouds and glowing avalanches , which are responsible for some devastating catastrophes during volcanic eruptions in human history , are special forms of pyroclastic density currents.
requirements
Pyroclastic density flows contain more than 75% pyroclasts, i.e. juvenile material from the magma, but also fine rock material of volcanic origin (lithic material or lithic tuff), which was pulverized during the eruption or when a lava dome breaks off due to the internal friction in a density flow . Further pyroclastic components are fine crystals (crystal tuff), pumice and glass fragments (vitric material or vitric tuff). In the transition area to the surges , the density currents generated by phreatic explosions are often included, although according to the definition of a phreatic explosion these only contain shattered "old rock". Pure phreatic explosions without pyroclasts are rare or are usually quickly followed by phreatomagmatic explosions , which then also contain pyroclastic material.
history
The terms for this transport and deposition process, the definitions of these terms and the further subdivision differ in the literature e.g. T. considerably; so far there is hardly any consensus. In the older literature, the term pyroclastic flow is used very often for this transport and deposition process. This is defined very narrowly by some authors (corresponds here to the pyroclastic flow, see str.), While other authors have interpreted it relatively broadly (then almost corresponds to the term pyroclastic density flow). However, in its broadest version it does not include the "wet" and low-temperature pyroclastic surges. In any case, this term describes only partial aspects and not the entire spectrum of pyroclastic density flows.
The main reasons for the very different opinions are that pyroclastic density currents are still relatively little investigated. The processes inside a stream (e.g. density, temperature, flow regime) cannot be observed directly. The flow rate can be calculated, for example, with the help of photo series, provided that there were observers of a volcanic eruption. This is rarely the case with remote volcanoes.
definition
During the explosive eruption of a volcano, mixtures of gas (liquid) solid particles can form, which are heavier than air. They therefore mostly flow along the ground until their kinetic energy is used up and the particles have gradually settled. The speed of the flows, the water vapor content, the grain size, the flow regime, the density, the chemical composition of the gases and the temperature do not initially play a role; The decisive factor is the gas (liquid) solid particle mixture, the solid particles consisting of more than 75% pyroclasts.
In the English-language literature, the umbrella term pyroclastic density current has become established for this transport and deposition process, which encompasses the entire spectrum of this transport and deposition process (see for example). It also explicitly includes the "diluted" end, ie a gas (liquid) particle mixture with a relatively low particle density (and possibly a low temperature), but often at high speed. In this area, the definitions of pyroclastic density flow and surges overlap . At the other "dense" end of the pyroclastic density flows there is also a transition area to the volcanic, but "cold" debris avalanches ( debris avalanches ).
Subdivision
Pyroclastic density flows are classified according to their terminal members, relatively particle-rich, highly concentrated flows and relatively low-particle, low-concentration flows in
- pyroclastic currents i. e. S., relatively rich in particles, highly concentrated
- pyroclastic surges , relatively low in particles, low concentration, "wet" and "dry"
divided. The boundaries between the two types of mass transport are not defined, however. While pyroclastic surges usually contain less than 1% solid material, pyroclastic currents can contain tens of% solid material. The speed can be the same for both types of density flow (between a few tens of meters per second up to about 300 m / s). Pyroclastic surges can, at least theoretically, reach higher speeds than pyroclastic currents i. e. S.
The literature emphasizes that there is no continuum between the end links; this is (also) put forward as an argument for the elimination of the two classes. In addition to the density, there are two further clear differences between the highly concentrated (or dense) and the low-concentration ("diluted") density flows:
- the velocity fluctuates strongly in the low-concentration streams; it is much more constant and steady in the highly concentrated currents
- in low-concentration currents, turbulence is the main transport mechanism; in the case of the highly concentrated, the grain-grain collision.
Therefore, one of the authors emphasized the differences mentioned; the other authors point out that pyroclastic currents can change into pyroclastic surges and very often alternate in flow deposits.
Emergence
Pyroclastic density currents arise mainly on volcanoes that promote acidic, silicic acid-containing and gas-rich lavas . The acidic lava is viscous and can clog the volcanic vent. This can greatly increase the pressure inside the volcano and cause a powerful explosion. However, they are not limited to the volcanoes with acidic lava, but also occur in volcanoes that produce basic magma. The actual causes for pyroclastic density currents are named in the literature:
- Collapse of an eruption cloud
- direct eruption from the crater
- Part of the lava dome is broken off, components explode
- When a debris avalanche breaks off on the flanks of a volcano, a magma reservoir is exposed
- Lateral eruption with a blocked chimney, rapid decompression of an initially highly compressed mixture of gas and pyroclastic material
- Explosion at the front of a lava flow
- Interaction of rising magma with hydrothermally modified rocks
- Interaction of a pyroclastic flow with water can trigger a secondary density flow, which can have a completely different direction than the triggering flow.
- phreatomagmatic explosions
As a rule, these events initially result in pyroclastic currents i. e. S., which often change rapidly. Pyroclastic surges are mainly caused by lateral, detonation-like outbreaks and by phreatomagmatic explosions .
The pyroclastic surges can reach speeds of over 1000 km / h. The density flows observed on Mt. St. Helens, for example, initially had speeds of around 350 km / h, but later they quickly increased to around 1080 km / h. At least in theory, significantly higher speeds are possible.
Change during transport
Pyroclastic density currents often change during transport, often shortly after their formation. They can be separated into a dense "lower cloud" (= pyroclastic current see str.) And a less dense "upper cloud" (pyroclastic surge). The transport direction of both streams can separate if there are obstacles. When Mont Pelée erupted, the pyroclastic current s followed. st. a creek while the pyroclastic surge left the valley and devastated the city of St. Pierre.
By absorbing air, a secondary convection cloud can rise from a density flow (so-called "Phoenix cloud" ). This cloud can be displaced by the wind and in turn produce pyroclastic fall deposits (mostly only ash) ("co-ignimbrite ash-fall deposits").
Pyroclastic currents s. st. can also move submarine, as a cushion of air forms around the stream. However, it should also be remembered that at least in shallow water, the water is displaced. Smaller bodies of water such as lakes can almost completely evaporate and trigger further water vapor explosions, as in the eruption of Mount St. Helens in 1980. Due to their relatively low density, pyroclastic surges often move tens of kilometers on the surface of water.
Deposits from pyroclastic density flows
The deposits from pyroclastic density flows are generally called pyroclastic flow deposits . Analogous to the pyroclastic case deposits, they can be further subdivided according to grain size and components. However, in the case of pyroclastic flow deposits there is also the temperature factor. They can be very hot, e.g. In some cases, the currents can heat up further during transport (friction, combustion of flammable gases). The clasts can already completely or partially melt during transport, or else only melt, partially melt or become plastic after they have been deposited.
Each density flow produces a flow unit , with outbreaks there are usually several density flows that produce several flow units. Since the time between the individual density flows is very different, the cooling of the individual flows is also very different.
Dangers from pyroclastic density currents
A pyroclastic density flow destroys almost everything that lies on its way. The transported components, from block to ash size, typically move at speeds of over 80 km / h. They knock down, smash, bury or drag almost all objects with them that are on their way down the valley. The mostly high temperatures between around 200 ° C and 700 ° C in the current ignite all combustible material. People and animals at the edges of the density streams can suffocate or be badly burned by the hot gases.
Pyroclastic density currents mostly follow valleys or flood low-lying areas; the flow range naturally depends on the volume and the composition or density of the flow. You can fill up valleys with up to 200 m thick deposits or cover low-lying areas over a large area with thin deposits in the meter range. Large areas of agricultural land can be destroyed in the process. If the deposits are cooler than around 500 ° C (and therefore do not merge), these loose deposits can lead to lahars in heavy rain . For example, they can block rivers and lead to the damming of a lake behind the dam. If this dam breaks, a lahar i. w. S. be triggered. Hot pyroclastic currents can also trigger lahars directly or transform them into lahars. This happens through the melting of ice and snow on the flanks of a volcano and the subsequent mixing and erosion of other volcanic loose masses (for example, 1985 on Nevado del Ruiz , Colombia).
Some notable pyroclastic density flows in the recent past
6350 years ago, the eruption of the Kikai volcano in Japan created a pyroclastic density flow, the "upper cloud" of which crossed a sea surface of 40 km and then continued to flow 60 km on a neighboring island.
The eruption of a volcano in the Long Valley in the US state of California 760,000 years ago created a pyroclastic density flow that crossed a 1000 m high mountain range of the Sierra Nevada.
swell
literature
- Alain Burgisser, George W. Bargantz: Reconciling Pyroclastic Flow and Surge: the Multiphase Physics of Pyroclastic Density Currents. In: Earth and Planetary Science Letters. 202, 2, 2002, pp. 405-418, doi : 10.1016 / S0012-821X (02) 00789-6 .
- Haraldur Sigurdsson (Ed.): Encyclopedia of Volcanoes. Academic Press, San Diego CA et al. 2000, ISBN 0-12-643140-X .
- Elisabeth A. Parfitt, Lionel Wilson: Fundamentals of Physical Volcanology. Blackwell Publishing, Malden MA et al. 2008, ISBN 978-0-632-05443-5 .
- Hans Pichler, Thomas Pichler: volcanic areas of the earth. Spectrum Akademischer Verlag, Heidelberg 2007, ISBN 978-3-8274-1475-5 .
- Gerd Simper: Understanding and experiencing volcanism. Feuerland Verlag, Stuttgart 2005, ISBN 3-00-015117-6 .
Individual evidence
- ↑ a b B. Behncke, S. Calvari, S. Giammanco, M. Neri, H. Pinkerton: Pyroclastic density currents resulting from the interaction of basaltic magma with hydrothermally altered rock: an example from the 2006 summit eruptions of Mount Etna, Italy . In: Bulletin of Volcanology. 70, pp. 1249-1268, Berlin / Heidelberg 2008 doi : 10.1007 / s00445-008-0200-7 .
- ↑ M. Edmonds, RA Herd: Inland-directed base surge generated by the explosive interaction of pyroclastic flows and seawater at Soufrière Hills volcano, Montserrat. In: Geology. 33, pp. 245-248, Boulder, Col. doi : 10.1130 / G21166.1 .
annotation
- ↑ The temperatures of over 1000 ° C given in the literature seem to be too high. The example of Mt. Pelée is cited very often. But at that time the investigation of density currents was at the very beginning and an exact temperature determination was and is still not possible today. Today temperatures up to about 800 ° C are mentioned; for example Pichler and Pichler (2007). However, this does not rule out that higher temperatures are theoretically possible.