MicroTCA
MicroTCA (also: µTCA ) stands for Micro Telecommunications Computing Architecture and is a modular standard adopted by the PICMG that regulates the structure of subracks and complete basic systems . The MicroTCA specification defines the requirements for a system that operates PICMG AdvancedMCs directly on a backplane . The specification describes general mechanical, electrical, thermal and management-related properties of a MicroTCA system that are necessary to support modules that are compatible with the AdvancedMC standard.
MicroTCA is complementary to PICMG3.0 Advanced Telecommunications Computing Architecture ( AdvancedTCA ). While AdvancedTCA was designed for high capacity and high performance applications, MicroTCA is focused on cost sensitive, physically smaller applications with lower capacity, performance and possibly less strict availability requirements. MicroTCA retains many of AdvancedTCA's important philosophies, including the basic interconnection topologies and management structures.
overview
history
MicroTCA was developed out of the need to have a high-speed system platform available that would meet both the high demands of the telecommunications sector and the less demanding needs of industry. For this purpose, the Advanced Mezzanine Cards (AdvancedMCs), which were developed for the AdvancedTCA telecommunications platform, were plugged directly onto a backplane. As a result, high cost savings can be achieved in the area of mechanics, electronics and management components, while still using the advantages of high data rates, redundancy and modularity.
Market positioning
In the telecommunications sector, MicroTCA positions itself in the middle of the bandwidth range due to some restrictions both in the number of modules and in the topology . MicroTCA systems with low bandwidth can be used as workgroup routers or WLAN base stations, while systems with high bandwidth can be used, for example, as DSL access points ( DSLAMs ).
Modularity
MicroTCA allows a multitude of possibilities in terms of modularity. The systems can be designed in the most varied of complexity levels. The advantage of a modular structure of the systems lies in the simple interchangeability of components, which thanks to the extensive management is even possible during ongoing operation. For example, a defective fan can be replaced by replacing the corresponding fan cassette without having to shut down the system. This contributes to the smooth operation and high reliability of the systems. This is essential for telecom applications in particular, as these systems must be constantly available.
Components
A MicroTCA system has several different components that can be plugged into the system. On the one hand, up to twelve AdvancedMCs can be integrated in the system. In addition, up to four Power Modules designed for redundant operation can be integrated. In addition, one or two cooling units (fan cassettes) are integrated into a system. All interchangeable components are called " Field Replaceable Units " (FRUs).
System concepts
MicroTCA Pico
The MicroTCA specification allows several concepts to implement a system. The smallest unit that complies with the MicroTCA standard is the MicroTCA Pico. The pico is usually a sub-assembly of a larger application. The external dimensions are not stipulated and are usually no larger than the subrack itself. The applications that are based on a Pico usually only require low power, which is why there are usually only a few slots in the system.
MicroTCA Cube
Another design that is defined by the MicroTCA specification is the MicroTCA Cube. This has approximately the shape of a cube (English "cube"). Due to the specified depth of the card basket, the cube has an edge length of approx. 200 mm. A MicroTCA Cube, like a Pico, is usually only part of a larger assembly. In contrast to the Pico, the cube is often larger than the card basket itself. The cube has additional space for MCHs and PMs, which may be pluggable and therefore modular. Often the CUs are already integrated into the cube, which means that it can be used as a completely independent system. These cubes are particularly useful as test systems for card developers or system integrators.
MicroTCA shelf
The largest and most common system is the so-called MicroTCA Shelf. This is a basic system with a width of 19 inches, which is common in this market segment. This means that cards with a total width of 84 HP can be integrated. The shelf usually contains two MCHs , two Power Modules and two Cooling Units. The cooling units are attached below or above the card basket, MCHs and power modules are in the card basket. The most common sizes of the plug-in modules (AdvancedMCs, MCHs and Power Modules) are "Single Module, Full Size". This means a height of approx. 75 mm and a width of 6 TE. The integration of two MCHs and two Power Modules leaves 60 TE that can be used for the AdvancedMCs. This means that eight "full size" modules (6 TE) and four "compact size" modules (3 TE) can be integrated, whereby two "compact size" slots located next to each other can also be used for a "full size" AdvancedMC.
cooling
The cooling in a MicroTCA system is very important because the power density in the system is very high. A module (AdvancedMC, MCH or Power Module) with the size "Double Module, Full Size" can generate up to 80 watts of waste heat. This means that a shelf can produce up to 14 times 80 watts = 1120 watts of waste heat.
management
The management in a MicroTCA system is very extensive. There is a central management instance in the system, the MicroTCA Carrier Hub (MCH). This is connected to all AdvancedMCs via a star-shaped IPMI bus and to the remaining components via a redundant IPMI bus. The MCH activates and deactivates all components and their ports .
Features
The management of the MicroTCA system is very extensive thanks to the IPMI protocol and supports numerous features. Temperatures in the system and on the modules are queried via this bus, and fan speeds are checked and readjusted. Above all, however, the hot swap is an important function in order to enable a smooth exchange of the components during operation. This minimizes the idle times of the system, which means that applications and services are always available. The management also supports electronic keying (e-keying). This is a function that makes it possible to query the compatibility of the modules before commissioning and, depending on the module, then to activate it completely, partially or not at all. For example, modules whose operating voltages or power are not compatible with the system are not activated. Modules whose protocols are not compatible with the switching functions of the MCH on certain ports can activate, but are not allowed to activate the affected ports.
Management controller
Every module that is integrated into a MicroTCA system and that is replaceable must have a management controller. The AdvancedMCs must have a "Module Management Controller" (MMC), the Power Modules, Cooling Units and application-specific modules must have an "Enhanced Module Management Controller" (EMMC). The task of these management controllers is to communicate with the MCH's management controller, which is called the "MicroTCA Carrier Management Controller" (MCMC). This communication is necessary to support hot-swap and e-keying.
links
The backplane is one of the most important components in the MicroTCA system. It contains all connections between the components. This includes the serial high-speed ports, the clock networks, the management connections and the power supply.
Ports
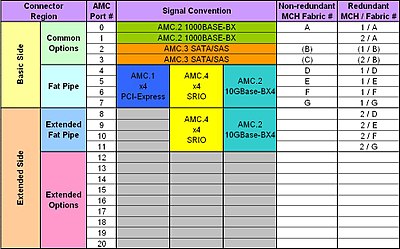
In order to be able to realize the required high bandwidths, the AdvancedMCs are connected via the high-speed ports. These consist of two differential pairs that enable fast transmission in the range of several GBit / s. The signaling takes place via low voltage differential signaling . The PCI Express , Serial Rapid IO , (10) Gigabit Ethernet or Serial Attached SCSI protocols are transmitted via the ports . Each AdvancedMC can use up to 21 ports (port [0] to port [20]). The MicroTCA standard only specifies the first twelve ports. The rest can be used for customer-specific transmissions or for outputting signals to the rear of the system.
Clock networks
MicroTCA defines three clock networks (Clock 1, Clock 2 and Clock 3). The connections differ depending on whether the system is equipped with a redundant MCH or not. The frequency of Clock 1 and Clock 2 is 8 kHz, 1.544 MHz, 2.048 MHz or 19.44 MHz, depending on the requirements. Clock 3 has a frequency of 100 MHz and can be implemented as a spread spectrum clock to save costs.
Non-redundant clock network
If only one MCH is integrated in the system, the clock network is designed to be non-redundant. Individual point-to-point connections are established between all clock connections of the AdvancedMCs and the MCH. The MCH has 36 clock connections for this purpose, three clock connections for each AdvancedMC.
Redundant clock network
In the redundant clock network, the first clock of each AdvancedMC is connected to the first clock of the first MCH. The third cycle of the AdvancedMCs is connected to the first cycle of the second MCH (redundancy if the first MCH fails). The second cycle of the AdvancedMCs is connected to the second cycle of the two MCHs. This is made possible by the fact that the termination network is adapted in such a way that each participant sees a termination of 100 ohms despite branching. By connecting clock 1 of an MCH to clock 3 of the AdvancedMCs, no PCI Express can be transmitted here because the corresponding clock network is not available.
Change of specification
On November 15, 2006 revision 2.0 of the AdvancedMC.0 specification (Base Specification) was published. The clock connections and their designations have been revised in this specification. Two more clock networks have been added that are used in place of port [16]. The names have been changed so that the clock networks are now called TCLKA (Clock 1), TCLKB (Clock 2), FCLKA (Clock 3), TCLKC (new addition) and TCLKD (new addition). The letter 'T' in front of CLK (= Clock) stands for "Telecom", 'F' stands for "Fabric". It is expected that the MicroTCA specification will adapt to the changes.
Supply voltages
In classic bus systems, “power planes”, i.e. complete copper layers that are connected to the supply voltages, are integrated into the backplane. These copper layers are used to provide a simple way of connecting all slots and components to the supply voltages by means of vias. Furthermore, the use of complete copper layers has the advantage that these copper layers represent electrical shields, so that the impedance of the conductor tracks between these layers can also be adjusted uniformly and easily. The “Powerplanes” cannot be used with a MicroTCA system, as e-keying is used to monitor the compatibility of the inserted components. To support e-keying, it must be possible to switch all power supplies for the inserted modules on and off separately. Two different voltage levels are available in the system. On the one hand, this is “management power”, which supplies the management controller of the components with +3.3 V, which is responsible for controlling the e-keying. "Payload Power" is also used to supply the actual payload of the module. This +12 V must be converted locally to the required voltage level. With these separate power supplies, each component has two supply networks that must be separated from the other components.
Management connections
Numerous connections must be available for extensive management in a MicroTCA system. These are primarily the IPMI buses, the hardware and data transmission of which corresponds to the I²C bus. This means that every IPMI bus consists of a data line ("Serial Data", SDA) and a clock line ("Serial Clock", SCL). Each AdvancedMC is connected radially to the two MCHs via a separate IPMI connection. This means that twelve local IPMI connections (IPMI-L) are required. Furthermore, the Power Modules and Cooling Units and any application-specific modules that may be present are connected via two redundant IPMI connections. These two connections are called IPMI-A and IPMI-B and together make up IPMI-0. As the application-specific modules can be connected to the IPMI-0, the number of components available here is not limited. This is why IPMI-0 cannot be routed radially like the IPMI-L connections, but is arranged in a serial bus topology. In addition, there are contacts on the modules that are necessary for detection and activation. On the one hand, there are the present pins PS0 # and PS1 # and an ENABLE # pin. The PS0 # pin indicates to the module that it is completely plugged in, while PS1 # signals the presence of the module to the PMs. The PM then activates the ENABLE # pin and management power for this component. ENABLE # is also used to reset the management controller. The Power Module itself has no PS0 #, PS1 # and ENABLE # pins, but only has one PS_PM pin. This pin has the same function as the PS0 # pin and indicates to the Power Module that it is fully inserted and can therefore be activated.
Information modules
FRU information device
The FRU information device is used to store system-specific data. These are absolutely necessary to support functions such as e-keying. Information such as the performance of the power modules, consumption of the other modules, slot arrangement, port connections and activation cycles are saved. The communication partners can be identified through the port connections and thus the activation of the drivers can be controlled. A FRU information device is available for each MCH, which is connected to the MCH via a local I²C bus.
Carrier Locator
The carrier locator is used to localize the system in a larger arrangement. For this an I²C IO expander is connected to a DIP switch. This makes it possible to query the number of the system via the I²C bus. The carrier locator is connected to the MCH via the same I²C bus as the FRU information device.
Connectors
AdvancedMC and MCH connectors
The MicroTCA connector that connects the backplane with the modules was determined by the already existing AdvancedMCs. These were integrated into the system in AdvancedTCA systems via a "carrier card". The AdvancedMCs have "Card Edge" contacts, ie gold contacts that are located directly on the circuit board. Thus, the edge of the circuit board is plugged directly into the mating connector. Since the AdvancedMC is plugged in parallel to the carrier card but vertically on the backplane, a new, compatible connector has to be developed for MicroTCA. The connector has 170 contacts, 85 on either side of the circuit board. There are three types of MicroTCA connectors available on the market. There is an SMT connector that is soldered to the surface of the circuit board. There is also a compression mount connector that is simply screwed on. A connection to the circuit board is established via the spring contacts of the plug. The third connector is a press-fit connector .
To ensure uniformity, the MCH is plugged into the backplane using the same connector. However, the MCH requires a large number of contacts that cannot be routed via one of these connectors. For this reason, up to four of these connectors are attached directly next to each other on the backplane in order to route all connections of an MCH to the backplane.
Power connectors
The connector for the power modules requires, on the one hand, high-current contacts to supply the modules in the system and, on the other hand, signal contacts for the management functions. For this purpose, a separate connector was developed that has 24 high-current contacts and 72 signal contacts.
Web links
- MicroTCA page of the PICMG with a MicroTCA short form (English; PDF; 821 kB)
- Examples of some MicroTCA systems and accessories
- Example for a MicroTCA Carrier Hub (English)