Grain growth
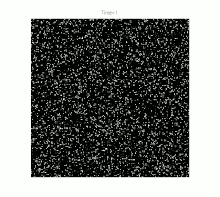
Grain growth occurs in polycrystalline solids . At sufficiently high temperatures and as soon as crystal recovery and recrystallization have taken place, a further reduction in internal energy can only be achieved by reducing the size of the grain boundaries. This happens through the growth of the largest and most favorably oriented crystallites ("grains") at the expense of smaller representatives. Ideally, but rarely in practice, this process can continue to the point where a single crystal is finally formed.
The term is commonly used in metallurgy , but can also refer to ceramic and mineral substances.
meaning
The majority of the materials show the Hall-Petch effect at room temperature and therefore achieve a higher yield point with smaller grain sizes . At high temperatures, the opposite is the case because the open and disordered structure of the grain boundaries means that voids can migrate more easily and quickly along the boundaries, which leads to higher coble creep (a type of creep ). Since grain boundaries are regions of high energy, they form excellent nucleation sites for precipitations and other secondary phases : e.g. B. Mg-Si-Cu phases in some aluminum alloys or martensite flakes in steel . Depending on the second phase involved, this can have positive or negative effects on the macroscopic properties.
regulate
For a long time, grain growth was studied primarily by examining cut, polished and etched samples under an optical microscope . Although this resulted in a wide range of empirical illustrative material, especially with regard to temperature curves and material compositions , the lack of knowledge of the crystal structure limited the understanding of the fundamental physical processes. Nevertheless, one could define the following basic properties of grain growth:
- Grain growth occurs through migration of the interfaces, not through coalescence (as is the case with water droplets).
- The migration of the interfaces is discontinuous, the direction of movement can suddenly change.
- One grain can grow into the other while it is consumed by another from the other.
- The consumption rate often increases when a grain is almost used up.
- An arcuate border usually migrates radially.
- As soon as the grain boundaries of a single phase meet at an angle ≠ 120 °, the grain that is hit by the more acute angle is used up, so that all angles strive for 120 °.
Normal and abnormal growth
Together with crystal recovery and recrystallization , growth phenomena can be divided into continuous and discontinuous processes. In the former, the structure from state A to state B is uniform, which means that the grain sizes become larger. In the second case, the changes take place heterogeneously, so that particular transformed and untransformed areas can be identified.
Discontinuous grain growth , also known as secondary recrystallization , is characterized by a proportion of rapidly growing grains that quickly use up their neighbors; it tends to result in a microstructure in which a few very large grains dominate. In order for this to take place, a subset of grains must have an advantage over the competition, e.g. high interfacial energy , high local mobility of the interface (i.e. high temperature), a favorable structure or lower particle density of a secondary phase that "pins" the grain boundaries.
Driving force
The boundary between a grain and its neighbor forms a defect in the crystal structure and is therefore associated with a certain amount of energy. The result is a thermodynamic effort to reduce the total area of the grain boundaries. This happens during grain growth with a simultaneous reduction in the total number of individual grains.
Compared to phase transitions , the internal energy that can be achieved in grain growth is low, so it takes place much more slowly and can easily be weakened by particles or loose atoms.
Ideal growth
Ideal growth is a special case in which only the reduction of the general interfacial energy is effective. Other environmental conditions such as elastic stresses or temperature gradients are neglected. If it can be said that the growth rate is proportional to the acting force and the acting force is proportional to the total interfacial energy, it can be shown that the time t required to reach a certain grain diameter can be approximated by the following equation :
where d 0 is the original grain size, d is the finite grain size and k is a temperature-dependent constant that is formed exponentially:
where k 0 is a constant, T the absolute temperature and Q the activation energy for interfacial displacements. In theory, the activation energy for interfacial shifts is as much as that for self-diffusion , but this is often not the case.
In general, these equations apparently apply to high-purity materials, but fail when tiny amounts of dissolved substances are introduced.
Growth inhibiting factors
If there are other factors that hinder the boundary movements, such as Zener pinning by small particles, the grain size can be limited to a value much smaller than one would normally expect. This is an important manufacturing mechanism to prevent the softening of materials at high temperatures. In fine-grain structural steel , the carbonitrides of the micro-alloying elements titanium , niobium and vanadium take on this role.