RNA

Ribonucleic acid or RNA is a nucleic acid, consisting of many nucleotides that form a polymer. Each nucleotide consists of a nitrogenous base, a ribose sugar, and a phosphate. RNA plays several important biological roles, including many processes involving translation of genetic information from deoxyribonucleic acid (DNA) into proteins. One type of RNA, messenger RNA, carries information from DNA to the protein synthesis complexes known as ribosomes, whereas ribosomal RNAs form vital portions of the structure of ribosomes and transfer RNAs act as essential carrier molecules for amino acids to be used in protein synthesis. It has also been known since the 1990s that several types of RNA regulate which genes are active.
RNA is very similar to DNA, but differs in a few important structural details: in the cell RNA is usually single stranded, while DNA is usually double stranded. RNA nucleotides contain ribose while DNA contains deoxyribose (a type of ribose that lacks one oxygen atom), and RNA uses the nucleotide uracil in its composition, instead of thymine which is present in DNA. RNA is transcribed from DNA by enzymes called RNA polymerases and is generally further processed by other enzymes. Some of these RNA processing enzymes contain their own RNAs.
Structure
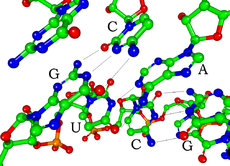
Each nucleotide in RNA contains a ribose sugar, with carbons numbered 1' through 5'. A base is attached to the 1' position, generally adenine, cytosine, guanine or uracil. A phosphate group is attached to the 3' position of one ribose and the 5' position of the next. The phosphate groups have a negative charge each at physiological pH, making RNA a charged molecule (polyanion). The bases may form hydrogen bonds between cytosine and guanine, between adenine and uracil and between guanine and uracil.[1] However other interactions are possible, such as a group of adenine bases binding to each other in a bulge,[2] or the GNRA tetraloop that has a guanine–adenine base-pair.[1]
An important structural feature of RNA that distinguishes it from DNA is the presence of a hydroxyl group at the 2' position of the ribose sugar. The presence of this functional group enforces the C3'-endo sugar conformation (as opposed to the C2'-endo conformation of the deoxyribose sugar in DNA) that causes the helix to adopt the A-form geometry rather than the B-form most commonly observed in DNA.[3] This results in a very deep and narrow major groove and a shallow and wide minor groove.[4] A second consequence of the presence of the 2'-hydroxyl group is that in conformationally flexible regions of an RNA molecule (that is, not involved in formation of a double helix), it can chemically attack the adjacent phosphodiester bond to cleave the backbone.[5]
RNA is transcribed with only four bases (adenine, cytosine, guanine and uracil),[6] but there are numerous modified bases and sugars in mature RNAs. Pseudouridine (Ψ), in which the linkage between uracil and ribose is changed from a C–N bond to a C–C bond, and ribothymidine (T), are found in various places (most notably in the TΨC loop of tRNA).[7] Another notable modified base is hypoxanthine, a deaminated guanine base whose nucleoside is called inosine. Inosine plays a key role in the wobble hypothesis of the genetic code.[8] There are nearly 100 other naturally occurring modified nucleosides,[9] of which pseudouridine and nucleosides with 2'-O-methylribose are the most common.[10] The specific roles of many of these modifications in RNA are not fully understood. However, it is notable that in ribosomal RNA, many of the post-transcriptional modifications occur in highly functional regions, such as the peptidyl transferase center and the subunit interface, implying that they are important for normal function.[11]
The functional form of single stranded RNA molecules, just like proteins, frequently requires a specific tertiary structure. The scaffold for this structure is provided by secondary structural elements which are hydrogen bonds within the molecule. This leads to several recognizable "domains" of secondary structure like hairpin loops, bulges and internal loops.[12] There has been a significant amount of research directed at the RNA structure prediction problem.
Comparison with DNA
RNA and DNA differ in three main ways. First, unlike DNA which is double-stranded, RNA is a single-stranded molecule in most of its biological roles and has a much shorter chain of nucleotides. Secondly, while DNA contains deoxyribose, RNA contains ribose, (there is no hydroxyl group attached to the pentose ring in the 2' position in DNA, whereas RNA has two hydroxyl groups). These hydroxyl groups make RNA less stable than DNA because it is more prone to hydrolysis. Thirdly, the complementary nucleotide to adenine is not thymine, as it is in DNA, but rather uracil, which is an unmethylated form of thymine.[13]
Like DNA, most biologically active RNAs including tRNA, rRNA, snRNAs and other non-coding RNAs are extensively base paired to form double stranded helices. Structural analysis of these RNAs have revealed that they are highly structured. Unlike DNA, this structure is not long double-stranded helices but rather collections of short helices packed together into structures akin to proteins. In this fashion, RNAs can achieve chemical catalysis, like enzymes.[14] For instance, determination of the structure of the ribosome – an enzyme that catalyzes peptide bond formation – revealed that its active site is composed entirely of RNA.[15]
Synthesis
Synthesis of RNA is usually catalyzed by an enzyme – RNA polymerase – using DNA as a template. Initiation of synthesis begins with the binding of the enzyme to a promoter sequence in the DNA (usually found "upstream" of a gene). The DNA double helix is unwound by the helicase activity of the enzyme. The enzyme then progresses along the template strand in the 3’ to 5’ direction, synthesizing a complementary RNA molecule with elongation occurring in the 5’ to 3’ direction. The DNA sequence also dictates where termination of RNA synthesis will occur.[16]
There are also a number of RNA-dependent RNA polymerases as well that use RNA as their template for synthesis of a new strand of RNA. For instance, a number of RNA viruses (such as poliovirus) use this type of enzyme to replicate their genetic material.[17] Also, it is known that RNA-dependent RNA polymerases are required for the RNA interference pathway in many organisms.[18]
Types of RNA
Messenger RNA
Messenger RNA (mRNA) is RNA that carries information from DNA to the ribosome sites of protein synthesis in the cell. In eukaryotic cells, once mRNA has been transcribed from DNA, it is processed before being exported from the nucleus into the cytoplasm, where it is bound to ribosomes and translated into its corresponding protein form with the help of tRNA. In prokaryotic cells, which do not have nucleus and cytoplasm compartments, mRNA can bind to ribosomes while it is being transcribed from DNA. After a certain amount of time the message degrades into its component nucleotides with the assistance of ribonucleases.[19]
Non-coding RNA

RNA genes are genes that encode RNA which is not translated into a protein, known as non-coding RNA or small RNA. Non-coding RNAs can also derive from introns.[20] The most prominent examples of non-coding RNAs are transfer RNA (tRNA) and ribosomal RNA (rRNA), both of which are involved in the process of translation.[13] There are also non-coding RNAs involved in gene regulation, RNA processing and other roles.
Certain RNAs are able to catalyse chemical reactions such as cutting and ligating other RNA molecules,[21] and the catalysis of peptide bond formation in the ribosome;[15] these are known as ribozymes.
Double-stranded RNA
Double-stranded RNA (dsRNA) is RNA with two complementary strands, similar to the DNA found in all cells. dsRNA forms the genetic material of some viruses called double-stranded RNA viruses. In eukaryotes, long double-stranded RNA such as viral RNA can trigger RNA interference, where short dsRNA molecules called siRNAs (short interfering RNAs) can cause enzymes to break down specific mRNAs or silence the expression of genes.[22] siRNA can also increase the transcription of a gene, a process called RNA activation.[23]
List of RNAs
Type | Abbr. | Function | Distribution | Ref. |
---|---|---|---|---|
Messenger RNA | mRNA | Codes for protein | All organisms | |
Ribosomal RNA | rRNA | Translation | All organisms | |
Transfer RNA | tRNA | Translation | All organisms | |
Transfer-messenger RNA | tmRNA | Rescuing stalled ribosomes | Bacteria | [24] |
Antisense RNA | aRNA | Gene regulation | All organisms | [25] |
Small interfering RNA | siRNA | Gene regulation | Most eukaryotes | [26] |
MicroRNA | miRNA | Gene regulation | Most eukaryotes | [27] |
Piwi-interacting RNA | piRNA | Gene regulation | Animals | [28] |
Small nuclear RNA | snRNA | Various | Eukaryotes and archaea | [29] |
Small nucleolar RNA | snoRNA | Nucleotide modification of RNAs | Eukaryotes and archaea | [30] |
Guide RNA | gRNA | mRNA modification | Kinetoplastid mitochondria | [31] |
Ribonuclease P | RNase P | tRNA maturation | All organisms | [32] |
Ribonuclease MRP | RNase MRP | rRNA maturation, DNA replication | Eukaryotes | [33] |
Y RNA | RNA processing, DNA replication | Animals | [34] | |
Telomerase RNA | Telomere synthesis | Most eukaryotes | [35] | |
Signal recognition particle RNA | SRP RNA | Protein export | All organisms | [36] |
Retrotransposon | Self-propagating | Eukaryotes and some bacteria | [37] | |
Viroid | Self-propagating | Infected plants | [38] |
[39] In addition, the genomes of many types of viruses consist of RNA, namely double-stranded RNA viruses, positive-sense RNA viruses, negative-sense RNA viruses and most satellite viruses and reverse transcribing viruses.
Biological roles
Translation
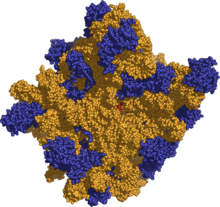
Messenger RNA (mRNA) carries information about protein sequence to the ribosomes, the protein synthesis factories in the cell. It is coded so that each three nucleotides (a codon) correspond to one amino acid. In addition to mRNA, two types of RNA are central to translation: transfer RNA (tRNA) and ribosomal RNA (rRNA).[19]
Transfer RNA is a small RNA chain of about 80 nucleotides that transfers a specific amino acid to a growing polypeptide chain at the ribosomal site of protein synthesis, during translation. It has sites for amino acid attachment and an anticodon region for codon recognition that binds to a specific sequence on the messenger RNA chain through hydrogen bonding.[20]
Ribosomal RNA is the catalytic component of the ribosomes. Eukaryotic ribosomes contain four different rRNA molecules: 18S, 5.8S, 28S, and 5S rRNA. Three of the rRNA molecules are synthesized in the nucleolus, and one is synthesized elsewhere. In the cytoplasm, ribosomal RNA and protein combine to form a nucleoprotein called a ribosome. The ribosome binds mRNA and carries out protein synthesis. Several ribosomes may be attached to a single mRNA at any time.[19] rRNA is extremely abundant and makes up 80% of the 10 mg/ml RNA found in a typical eukaryotic cytoplasm.[40]
Gene regulation
Several types of RNA can downregulate gene expression by being complementary to a part of a gene. MicroRNAs (miRNA; 21-22 nt) are found in eukaryotes and act through RNA interference (RNAi), where an effector complex of miRNA and enzymes can break down mRNA which the miRNA is complementary to, block the mRNA from being translated, or cause the promoter to be methylated which generally downregulates the gene.[41] Some miRNAs upregulate genes instead (RNA activation).[42] While small interfering RNAs (siRNA; 20-25 nt) are often produced by breakdown of viral RNA, there are also endogenous sources of siRNAs.[43] siRNAs act through RNA interference in a fashion similar to miRNAs. Animals have Piwi-interacting RNAs (piRNA; 29-30 nt) which are active in germline cells and are thought to be a defense against transposons and play a role in gametogenesis.[28][44] X chromosome inactivation in female mammals is caused by Xist, an RNA which coats one X chromosome, inactivating it.[45] Antisense RNAs are widespread among bacteria; most downregulate a gene, but a few are activators of transcription.[46]
RNA processing
Many RNAs are involved in modifying other RNAs. Spliceosomes splice introns out of pre-mRNA; these contain several small nuclear RNAs (snRNA).[13]
RNA can also be altered by having its nucleotides modified to other nucleotides than A, C, G and U. In eukaryotes, modifications of RNA nucleotides are generally directed by small nucleolar RNAs (snoRNA; 60-300 nt),[20] found in the nucleolus and cajal bodies. snoRNAs associate with enzymes and guide them to a spot on an RNA by basepairing to that RNA. These enzymes then perform the nucleotide modification. rRNAs and tRNAs are extensively modified, but snRNAs and mRNAs can also be the target of base modification.[47][48]
Discovery
Nucleic acids were discovered in 1868 by Friedrich Miescher, who called the material 'nuclein' since it was found in the nucleus.[49] It was later discovered that prokaryotic cells, which do not have a nucleus, also contain nucleic acids. The role of RNA in protein synthesis had been suspected since 1939, based on experiments carried out by Torbjörn Caspersson, Jean Brachet and Jack Schultz.[50] Gerard Marbaix isolated the first messenger RNA, for rabbit hemoglobin, and found it induced the synthesis of hemoglobin after injection into oocytes.[51] Finally, Severo Ochoa discovered how RNA is synthesized, winning Ochoa the 1959 Nobel Prize for Medicine.[52] The sequence of the 77 nucleotides of a yeast RNA was found by Robert W. Holley in 1965,[53] winning Holley the 1968 Nobel Prize for Medicine. Carl Woese and others realised RNA can be catalytic in 1967[54] and proposed the earliest forms of life relied on RNA both to carry genetic information and to catalyze biochemical reactions – an RNA world.[55] In 1976, Walter Fiers and his team at the University of Ghent determined the first complete nucleotide sequence of an RNA virus genome, that of bacteriophage MS2.[56] In the early 1990s it was found that introduced genes can silence homologous endogenous genes in plants.[57] At about the same time, 22 nt long RNAs, now known as microRNAs, were found to have a role in the development of C. elegans.[58] The discovery of gene regulatory RNAs led to attempts to develop drugs made of RNA, particularly to silence oncogenes and viral genes.[59] As of 2006 there is only one such drug on the market, Vitravene (an antisense RNA that inhibits a gene from cytomegalovirus), but there is promising research on using siRNAs to downregulate genes through RNA interference.[60]
See also
- Genetics
- Molecular biology
- Phosphoramidite
- Quantification of nucleic acids
- RNA Ontology Consortium
- Sequence profiling tool
References
- ^ a b Lee JC, Gutell RR (2004). "Diversity of base-pair conformations and their occurrence in rRNA structure and RNA structural motifs". J. Mol. Biol. 344 (5): 1225–49. doi:10.1016/j.jmb.2004.09.072.
- ^ Barciszewski J, Frederic B, Clark C (1999). RNA biochemistry and biotechnology. Springer. pp. 73–87. ISBN 0792358627.
{{cite book}}
: CS1 maint: multiple names: authors list (link) - ^ Salazar M, Fedoroff OY, Miller JM, Ribeiro NS, Reid BR (1992). "The DNA strand in DNAoRNA hybrid duplexes is neither B-form nor A-form in solution". Biochemistry. 1993 (32): 4207–15. PMID 7682844.
{{cite journal}}
: Cite has empty unknown parameter:|1=
(help)CS1 maint: multiple names: authors list (link) - ^ Hermann T, Patel DJ (2000). "RNA bulges as architectural and recognition motifs". Structure. 8 (3): R47–R54. doi:10.1016/S0969-2126(00)00110-6.
- ^ Mikkola S, Nurmi K, Yousefi-Salakdeh E, Strömberg R, Lönnberg H (1999). "The mechanism of the metal ion promoted cleavage of RNA phosphodiester bonds involves a general acid catalysis by the metal aquo ion on the departure of the leaving group". Perkin transactions 2: 1619–26. doi:10.1039/a903691a.
{{cite journal}}
: CS1 maint: multiple names: authors list (link) - ^ Jankowski JAZ, Polak JM (1996). Clinical gene analysis and manipulation: tools, techniques and troubleshooting. Cambridge University Press. p. 14. ISBN 0521478960.
- ^ Yu Q, Morrow CD (2001). "Identification of critical elements in the tRNA acceptor stem and TΨC loop necessary for human immunodeficiency virus type 1 infectivity". J Virol. 75 (10): 4902–6. doi:10.1128/JVI.75.10.4902-4906.2001.
- ^ Elliott MS, Trewyn RW (1983). "Inosine biosynthesis in transfer RNA by an enzymatic insertion of hypoxanthine". J. Biol. Chem. 259 (4): 2407–10. PMID 6365911.
- ^ Söll D, RajBhandary U (1995). tRNA: Structure, biosynthesis, and function. ASM Press. p. 165. ISBN 155581073X.
- ^ Kiss T (2001). "Small nucleolar RNA-guided post-transcriptional modification of cellular RNAs". The EMBO Journal. 20: 3617–22. doi:10.1093/emboj/20.14.3617.
- ^ King TH, Liu B, McCully RR, Fournier MJ (2002). "Ribosome structure and activity are altered in cells lacking snoRNPs that form pseudouridines in the peptidyl transferase center". Molecular Cell. 11 (2): 425–35. doi:10.1016/S1097-2765(03)00040-6.
{{cite journal}}
: CS1 maint: multiple names: authors list (link) - ^ Mathews DH, Disney MD, Childs JL, Schroeder SJ, Zuker M, Turner DH (2004). "Incorporating chemical modification constraints into a dynamic programming algorithm for prediction of RNA secondary structure". Proc. Natl. Acad. Sci. USA. 101 (19): 7287–92. doi:10.1073/pnas.0401799101.
{{cite journal}}
: CS1 maint: multiple names: authors list (link) - ^ a b c Berg JM, Tymoczko JL, Stryer L (2002). Biochemistry (5th edition ed.). WH Freeman and Company. pp. 118–19, 781–808. ISBN 0-7167-4684-0.
{{cite book}}
:|edition=
has extra text (help)CS1 maint: multiple names: authors list (link) - ^ Higgs PG (2000). "RNA secondary structure: physical and computational aspects". Quarterly Reviews of Biophysics. 33: 199–253. doi:10.1017/S0033583500003620.
- ^ a b Nissen P, Hansen J, Ban N, Moore PB, Steitz TA (2000). "The structural basis of ribosome activity in peptide bond synthesis". Science. 289 (5481): 920–30. doi:10.1126/science.289.5481.920.
{{cite journal}}
: CS1 maint: multiple names: authors list (link) - ^ Nudler E, Gottesman ME (2002). "Transcription termination and anti-termination in E. coli". Genes to Cells. 7: 755–68. doi:10.1046/j.1365-2443.2002.00563.x.
- ^ Jeffrey L Hansen, Alexander M Long, Steve C Schultz (1997). "Structure of the RNA-dependent RNA polymerase of poliovirus". Structure. 5 (8): 1109–22. doi:doi:10.1016/S0969-2126(97)00261-X.
{{cite journal}}
: Check|doi=
value (help)CS1 maint: multiple names: authors list (link) - ^ Ahlquist P (2002). "RNA-Dependent RNA Polymerases, Viruses, and RNA Silencing". Science. 296 (5571): 1270–73. doi:10.1126/science.1069132.
- ^ a b c Cooper GC, Hausman RE (2004). The Cell: A Molecular Approach (3rd edition ed.). Sinauer. pp. 261–76, 297, 339–44. ISBN 0-87893-214-3.
{{cite book}}
:|edition=
has extra text (help) - ^ a b c Wirta W (2006). Mining the transcriptome – methods and applications (PDF). ISBN 91-7178-436-5.
- ^ Rossi JJ (2004). "Ribozyme diagnostics comes of age". Chemistry & Biology. 11 (7): 894–95. doi:10.1016/j.chembiol.2004.07.002.
- ^ Blevins T; et al. (2006). "Four plant Dicers mediate viral small RNA biogenesis and DNA virus induced silencing". Nucleic Acids Res. 34 (21): 6233–46. PMID 17090584.
{{cite journal}}
: Explicit use of et al. in:|author=
(help) - ^ Doran G (2007). "RNAi – Is one suffix sufficient?". Journal of RNAi and Gene Silencing. 3 (1): 217–19.
{{cite journal}}
: Cite has empty unknown parameter:|1=
(help) - ^ Gillet R, Felden B (2001). "Emerging views on tmRNA-mediated protein tagging and ribosome rescue". Molecular Microbiology. 42 (4): 879–85. doi:10.1046/j.1365-2958.2001.02701.x.
- ^ Brantl S (2002). "Antisense-RNA regulation and RNA interference". Biochimica et Biophysica Acta. 1575 (1–3): 15–25. PMID 12020814.
- ^ Ahmad K, Henikoff S (2002). "Epigenetic consequences of nucleosome dynamics". Cell. 111 (3): 281–84. doi:10.1016/S0092-8674(02)01081-4.
- ^ Lin S-L, Miller JD, Ying S-Y (2006). "Intronic microRNA (miRNA)". Journal of Biomedicine and Biotechnology: 1–13. PMID 17057362.
{{cite journal}}
: CS1 maint: multiple names: authors list (link) - ^ a b Horwich MD, Li C Matranga C, Vagin V, Farley G, Wang P, Zamore PD (2007). "The Drosophila RNA methyltransferase, DmHen1, modifies germline piRNAs and single-stranded siRNAs in RISC". Current Biology. 17: 1265–72. doi:10.1016/j.cub.2007.06.030.
{{cite journal}}
: CS1 maint: multiple names: authors list (link) - ^ Thore S, Mayer C, Sauter C, Weeks S, Suck D (2003). "Crystal Structures of the Pyrococcus abyssi Sm Core and Its Complex with RNA". J. Biol. Chem. 278 (2): 1239–47. doi:10.1074/jbc.M207685200.
{{cite journal}}
: CS1 maint: multiple names: authors list (link) CS1 maint: unflagged free DOI (link) - ^ Kiss T (2001). "Small nucleolar RNA-guided post-transcriptional modification of cellular RNAs". The EMBO Journal. 20: 3617–22. doi:10.1093/emboj/20.14.3617.
- ^ Alfonzo JD, Thiemann O, Simpson L (1997). "The mechanism of U insertion/deletion RNA editing in kinetoplastid mitochondria". Nucleic Acids Research. 25 (19): 3751–59. PMID 9380494.
{{cite journal}}
: CS1 maint: multiple names: authors list (link) - ^ Pannucci JA, Haas ES, Hall TA, Harris JK, Brown JW (1999). "RNase P RNAs from some Archaea are catalytically active". Proc Natl Acad Sci USA. 96 (14): 7803–08. PMID 10393902.
{{cite journal}}
: CS1 maint: multiple names: authors list (link) - ^ Woodhams MD, Stadler PF, Penny D, Collins LJ (2007). "RNase MRP and the RNA processing cascade in the eukaryotic ancestor". BMC Evolutionary Biology. 7: S13. doi:10.1186/1471-2148-7-S1-S13.
{{cite journal}}
: CS1 maint: multiple names: authors list (link) CS1 maint: unflagged free DOI (link) - ^ Perreault J, Perreault J-P, Boire G (2007). "Ro-associated Y RNAs in metazoans: evolution and diversification". Molecular Biology and Evolution. 24 (8): 1678–89. doi:10.1093/molbev/msm084.
{{cite journal}}
: CS1 maint: multiple names: authors list (link) - ^ Lustig AJ (1999). "Crisis intervention: The role of telomerase". Proc Natl Acad Sci USA. 96 (7): 3339–41. PMID 10097039.
- ^ Gribaldo1 S, Brochier-Armanet C (2006). "The origin and evolution of Archaea: a state of the art". Philos Trans R Soc Lond B Biol Sci. 361 (1470): 1007–22. PMID 16754611.
{{cite journal}}
: CS1 maint: numeric names: authors list (link) - ^ Boeke JD (2003). "The unusual phylogenetic distribution of retrotransposons: a hypothesis". Genome Research. 13: 1975–83. PMID 12952870.
- ^ Flores R, Hernández C, Martínez de Alba AE, Daròs JA, Di Serio F (2005). "Viroids and viroid-host interactions". Annual Review of Phytopathology. 43: 117–39. PMID 16078879.
{{cite journal}}
: CS1 maint: multiple names: authors list (link) - ^ The housekeeping RNAs are from the list in Szymanski J, Barciszewska MZ, Zywicki M, Barciszewski J (2003). "Noncoding RNA transcripts". J. Appl. Genet. 44 (1): 9. PMID 12590177.
{{cite journal}}
: CS1 maint: multiple names: authors list (link) - ^ Kampers T, Friedhoff P, Biernat J, Mandelkow E-M, Mandelkow E (1996). "RNA stimulates aggregation of microtubule-associated protein tau into Alzheimer-like paired helical filaments". FEBS Letters. 399: 98–100, 344–49. PMID 8985176.
{{cite journal}}
: CS1 maint: multiple names: authors list (link) - ^ Matzke MA, Matzke AJM (2004). "Planting the seeds of a new paradigm". PLoS Biology. 2 (5): e133. doi:10.1371/journal.pbio.0020133.
{{cite journal}}
: CS1 maint: unflagged free DOI (link) - ^ Check E (2007). "RNA interference: hitting the on switch". Nature. 448 (7156): 855–58. doi:10.1038/448855a.
- ^ Vazquez F, Vaucheret H, Rajagopalan R, Lepers C, Gasciolli V, Mallory AC, Hilbert J, Bartel DP, Crété P (2004). "Endogenous trans-acting siRNAs regulate the accumulation of Arabidopsis mRNAs". Molecular Cell. 16 (1): 69–79. doi:10.1016/j.molcel.2004.09.028.
{{cite journal}}
: CS1 maint: multiple names: authors list (link) - ^ Girard A, Sachidanandam R, Hannon GJ, Carmell MA (2006). "A germline-specific class of small RNAs binds mammalian Piwi proteins". Nature. 442: 199–202. doi:10.1038/nature04917.
{{cite journal}}
: CS1 maint: multiple names: authors list (link) - ^ Heard E, Mongelard F, Arnaud D, Chureau C, Vourc'h C, Avner P (1999). "Human XIST yeast artificial chromosome transgenes show partial X inactivation center function in mouse embryonic stem cells". Proc. Natl. Acad. Sci. USA. 96 (12): 6841–46. PMID 10359800.
{{cite journal}}
: CS1 maint: multiple names: authors list (link) - ^ Wagner EG, Altuvia S, Romby P (2002). "Antisense RNAs in bacteria and their genetic elements". Adv Genet. 46: 361–98. PMID 11931231.
{{cite journal}}
: CS1 maint: multiple names: authors list (link) - ^ Covello PS, Gray MW (1989). "RNA editing in plant mitochondria". Nature. 341: 662–66. doi:10.1038/341662a0.
- ^ Omer AD, Ziesche S, Decatur WA, Fournier MJ, Dennis PP (2003). "RNA-modifying machines in archaea". Molecular Microbiology. 48 (3): 617–29. doi:10.1046/j.1365-2958.2003.03483.x.
{{cite journal}}
: CS1 maint: multiple names: authors list (link) - ^ Dahm R (2005). "Friedrich Miescher and the discovery of DNA". Developmental Biology. 278 (2): 274–88. PMID 15680349.
- ^ Nierhaus KH, Wilson DN (2004). Protein Synthesis and Ribosome Structure. Wiley-VCH. p. 3. ISBN 3527306382.
- ^ Carlier M (2003). "L'ADN, cette "simple" molécule". Esprit libre.
{{cite web}}
: Unknown parameter|month=
ignored (help) - ^ Ochoa S (1959). "Enzymatic synthesis of ribonucleic acid" (PDF). Nobel Lecture.
- ^ Holley RW; et al. (1965). "Structure of a ribonucleic acid". Science. 147 (1664): 1462–65. doi:10.1126/science.147.3664.1462.
{{cite journal}}
: Explicit use of et al. in:|author=
(help) - ^ Siebert S (2006). "Common sequence structure properties and stable regions in RNA secondary structures" (PDF). Dissertation, Albert-Ludwigs-Universität, Freiburg im Breisgau. p. 1.
- ^ Szathmáry E (1999). "The origin of the genetic code: amino acids as cofactors in an RNA world". Trends Genet. 15 (6): 223–9. doi:10.1016/S0168-9525(99)01730-8.
- ^ Fiers W; et al. (1976). "Complete nucleotide-sequence of bacteriophage MS2-RNA: primary and secondary structure of replicase gene". Nature. 260: 500–7. PMID 1264203.
{{cite journal}}
: Explicit use of et al. in:|author=
(help) - ^ Napoli C, Lemieux C, Jorgensen R (1990). "Introduction of a chimeric chalcone synthase gene into petunia results in reversible co-suppression of homologous genes in trans". Plant Cell. 2 (4): 279–89. PMID 12354959.
{{cite journal}}
: CS1 maint: multiple names: authors list (link) - ^ Ruvkun G (2001). "Glimpses of a tiny RNA world". Science. 294 (5543): 797–99. doi:10.1126/science.1066315.
- ^ Trent RJ (2005). Molecular Medicine (3rd edition ed.). Elsevier. pp. 160–63. ISBN 0-12-699057-3.
{{cite book}}
:|edition=
has extra text (help) - ^ Fichou Y, Férec C (2006). "The potential of oligonucleotides for therapeutic applications". Trends in Biotechnology. 24 (12): 563–70. doi:10.1016/j.tibtech.2006.10.003.
External links
- RNA World website
- Nucleic Acid Database Images of DNA, RNA and complexes.
- RNAJunction Database: Extracted atomic models of RNA junction and kissing loop structures.