Ion implantation
The ion implantation is a method for introducing foreign atoms (in the form of ions ) in a base material, doping mentioned. In this way, the material properties (mostly the electrical properties) of the base material can be changed. The process is used, among other things, in semiconductor technology . Corresponding systems for ion implantation are referred to as ion implanters .
principle
The basic procedure is the bombardment of solids in a high vacuum with accelerated ions. It can be divided into the following steps:
- Generation of ions in an ion source
- Extraction of the ions by an electrostatic field
- Separation of the ions according to mass in a mass separator
- Acceleration of ions
- Distraction by means of electric fields
- Implantation in the sample
The most important parameters for characterizing ion implantation are the acceleration energy, which can range from 500 eV to 6 MeV, and the implantation dose, which is in the range of 10 11 −10 18 cm −2 . Since ions are slowed down after entering the target material through a random collision with the electrons and atomic nuclei, the energy determines the range of the ions in the solid and the dose determines the doping concentration. Furthermore, the angle of incidence depending on the target material (possibly also its crystal orientation ) and the ion current density are important parameters for the concentration depth profile to be achieved.
During implantation, radiation damage occurs in the crystal lattice of the semiconductor, depending on the mass of the implanted ions and the implantation dose . Therefore, the substrate has to be annealed after an implantation step. This happens through a high temperature process in which the foreign atoms are built into the lattice and thus electrically activated and the lattice structure is restored. The healing process can be realized by an oven process or Rapid Thermal Annealing (RTA). The RTA process offers the advantage of separating and controlling the crystal healing process and the diffusion process that is always connected.
Range of ions
The range of the ions plays a decisive role in ion implantation. An important theory describing the range of ions in amorphous solids was proposed in 1963 by Jens Lindhard , Morten Scharff and Hans E. Schiøtt , commonly known as the LSS theory . It describes the braking of the ions by the electrons of the braking medium, the electron gas being a kind of viscous medium ( electronic braking ).
The LSS theory describes the dopant concentration in amorphous solids with good accuracy. It can also be used for mono- or polycrystalline solids. Under certain circumstances, however, there can be large deviations. For example, there can be a greater dopant concentration at a greater depth. The reason for this lies in the so-called lattice guide effect, which can occur with crystalline solids.
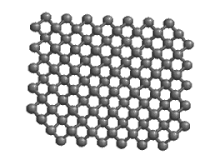
The channeling effect is an undesirable effect when doping monocrystalline silicon wafers. Depending on the crystal arrangement in the disk, there is the possibility that ions penetrate almost unchecked and therefore undesirably deep into the substrate due to the uniform crystal structure through the spaces between the atoms. The effect interferes with the exact process control, since it is very difficult to describe it using statistical relationships, but this works very well with scattered ions. The grid guidance can be prevented by inclining the substrate surface by approx. 7 ° and rotating it by 22 ° with respect to the <100> directions and / or coating it with a thin scattering oxide before implantation.
application
With ion implantation, a wide variety of material properties can be changed depending on the area of application. In semiconductor technology , ion implantation is used, among other things, to introduce foreign atoms to dop the semiconductor crystal, with the main goal being to change the electrical conductivity and charge carrier mobility . In this area, ion implantation has become the most important process and has largely replaced diffusion processes . Typical dopants are: aluminum , antimony , arsenic , boron , fluorine , gallium , germanium , indium , carbon , phosphorus , nitrogen or even oxygen , which is used, for example, in SIMOX technology ( separation by IMplanted OXygen ).
However, there are also numerous possible applications outside of microelectronics. Above all, they aim to change the color , hardness , optical properties , etchability , adhesion , gas diffusion and composition of a material.
Significance in semiconductor technology
In the 1970s and 1980s, ion implantation became the preferred method for doping silicon and other semiconductor materials. Previously, the doping was realized by diffusion processes. However, it is difficult to manufacture typical semiconductor structures (diodes, transistors, thyristors) with one or more pn junctions or different variants of these on a substrate (wafer) because the necessary process temperatures and times as well as the sequence of doping types are cleverly combined with one another Need to become. In addition, areas of opposite doping, as is necessary in CMOS technology, must be protected in a complex manner against the diffusion of the dopants. This was usually done using silicon oxide layers that were initially generated thermally (additional high-temperature step) and then locally removed by photolithographic structuring and etching, before the unprotected area is then exposed to the dopant source. Changes in the sequence of different doping (even in areas that are separate from one another) inevitably led to significantly changed doping profiles and electrical properties.
In contrast to high-temperature diffusion processes, ion implantation takes place at low temperatures (even when the substrate is heated to less than 100 ° C by ion bombardment) and is therefore compatible with photolithographic structuring. For local doping, a structured photoresist layer on the wafer and subsequent healing of the crystal damage (after removal of the foro-lacquer layer) is basically sufficient. This basic property enables a much more flexible process integration and was decisive for numerous improvements in the coming decades and was part of the cost reduction in microelectronics.
The ion implantation improved the production of near-surface doping for (local) setting of the threshold voltage of MOS field effect transistors and for the first time enabled threshold voltages below 1.5 V. The more flexible sequence in production (even if various high-temperature steps are still necessary in production) made it easier to manufacture various electronic components on one substrate, which has brought significant advantages in improving CMOS products, etc. In addition, the combination of ion implantation and local doping protection through a photoresist mask enabled numerous other improvements to the transistor properties, including sharper doping profiles (also important for bipolar transistors), reduced tolerance requirements, e.g. B. in the manufacture of the source-drain areas by self-aligning masking of the channel area with the help of the polysilicon gate electrode, the introduction of LDD field effect transistors with a low doped area near the gate electrode, the "halo" areas, well Isolation, channel stop and anti-punch-through implantations (mostly deep doping areas). These have brought significant improvements in terms of electrical properties and manufacturing tolerances and thereby enabled the constant miniaturization (scaling) of semiconductor components and integrated circuits or even new types of components or manufacturing variants.
Advantages and disadvantages
Compared to other methods, such as diffusion, ion implantation offers numerous advantages, but also some disadvantages, which are briefly summarized below.
Advantages:
- Short process times.
- High homogeneity and reproducibility .
- Possibility of implantation through already deposited thin layers.
- So-called “buried layers” can be created below the surface (e.g. SIMOX technology).
- The main process usually takes place at room temperature (heating at high current densities not considered), associated with this is a low thermal load and compatibility with the photolithographic structuring of a photoresist for local doping. Even higher temperatures are only necessary when the crystal damage heals, but by using short RTA processes they still allow significantly lower diffusion proportions (therefore sharper implantation profiles) and lower process scatter.
Disadvantage:
- Generation of radiation damage in the crystal lattice .
- Implantation is limited to layers close to the surface (up to approx. 5 µm).
- Profiles with sharp implantation boundaries cannot be generated (the transitions are, however, sharper than with diffusion doping).
- Due to additional effects, there may be deviations between the actual and the theoretical profile (see LSS theory and LBZ theory ).
- Use of problematic, toxic and polluting chemicals.
See also
literature
- JF Ziegler: Ion Implantation Science and Technology. Edgewater, USA 1996, ISBN 0-12-780620-2 .
- H. Ryssel, I. Ruge: Ion implantation. J. Wiley & Sons, Chichester 1986, ISBN 0-471-10311-X .
- Ulrich Hilleringmann: Silicon semiconductor technology. Teubner, 2004, ISBN 3-519-30149-0 .
Web links
Individual evidence
- ^ J. Lindhard, M. Scharff, HE Schiøtt: Range concepts and heavy ion ranges (Notes on atomic collisions, II) . In: Kgl. Danske Videnskab. Selskab. Mat. Fys. Medd . tape 33 , no. 14 , 1963, pp. 1-49 .
- ↑ Klaus Bethge , Gertrud Walter, Bernhard Wiedemann: Kernphysik . Springer, 2007, ISBN 978-3-540-74566-2 , pp. 121 ff .
- ↑ Dietrich Widmann, Hermann Mader, Hans Friedrich: Technology of highly integrated circuits . Springer, 1996, ISBN 3-540-59357-8 , pp. 228 f .
- ^ Andrew Marshall, Sreedhar Natarajan: SOI Design: Analog, Memory and Digital Techniques . Springer, 2002, ISBN 0-7923-7640-4 , pp. 6 ( limited preview in Google Book search).
- ↑ a b c Lis K. Nanver, Egbert JG Goudena: Ion implantation . In: Wiley Encyclopedia of Electrical and Electronics Engineering . American Cancer Society, 1999, ISBN 978-0-471-34608-1 , doi : 10.1002 / 047134608X.W7021 .
- ↑ Jörg Schulze: Concepts of silicon-based MOS components . Springer-Verlag, 2006, ISBN 978-3-540-27547-3 .