Acousto-optic modulator
An acousto-optical modulator (AOM, also Bragg cell ) is an optical component that modulates incident light in terms of frequency and direction of propagation or intensity . For this purpose, an optical grating is created in a transparent solid body with sound waves . The light beam is bent at this grating and its frequency is shifted at the same time.
Structure and functionality

An acousto-optical modulator traditionally consists of a transparent cuboid (e.g. quartz glass or a crystal) in which structure-borne sound ( ultrasound ) is generated by means of a piezo oscillator. There is a sound absorber opposite the piezoelectric exciter to avoid reflections and standing waves.
There are also fiber optic acousto-optic modulators. They offer lower insertion loss, better beam quality and easier integration into fiber optic systems. Such all-fiber AOMs use transverse or longitudinal acoustic waves running along the fiber . The latter can e.g. B. in turn modulate fiber Bragg gratings located in the fiber core .
The deflection of light in a traditional acousto-optic modulator works on the principle of diffraction of light on an optical grating. The optical grating consists of the density fluctuations of the sound wave passing through the crystal.
The sound wave with frequencies of typically 10 to 2000 MHz causes a periodic change in density in the crystal and thus a periodic modulation of the refractive index . The distance between these "grid lines" is equal to the wavelength of the ultrasonic wave and can be calculated from the speed of sound and the frequency of sound
- .
Mostly LiNbO 3 or PbMoO 4 for visible light and near infrared and Ge for medium infrared are used for the crystal . Typical speeds of sound in such crystals are between 3700 and 4300 m / s. A frequency of 195 MHz gives a lattice constant of 19 to 22 µm. These are typical values. The exact value depends on the ultrasonic frequency used and the speed of sound of the medium used.
Typically, the cross section of the incident light beam is significantly larger than the spatial period of the refractive index modulation, and since the speed of light is much greater than the speed of sound, one can approximately assume that the light looks and a static refractive index modulation constructive interference of light to the Bragg angle with
experiences, where the wavelength of the light in the crystal and the period of the refractive index modulation are.
The scattered light experiences a Doppler frequency shift with the frequency of the ultrasound. The process is similar to the reflection on a moving mirror.
Another approach, equivalent to this, considers the sound wave in the solid body as phonons that interact with the photons of light. The deflection of the light is caused by the fact that the momentum of the phonons is vectorially added to the momentum of the photons:
Here is the divided by Planck's quantum of action and the wave vector of the photons or phonons. From this perspective, it follows from the conservation of energy that the interaction changes the frequency of the light by the frequency of the sound wave:
Here is Planck's quantum of action and the frequency of the light, i.e. the photons. The term denotes the frequency of the sound wave. The frequency of the light is shifted exactly by the frequency of the sound wave.
The relative frequency shift of the light is very small, as the ultrasound frequency (≈ 10 7 … 10 9 Hz) is much smaller than the frequency of the light (≈ 10 14 … 10 15 Hz). However, it is essential for some applications.
Applications
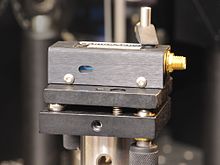
AOM are used to manipulate laser radiation. The applications can be broken down as follows:
-
Fast switching
- Q-switching in pulsed lasers .
- Blanking for scanning processes such as certain photolithography processes or laser shows .
-
Distraction and modulation
- Electrically controllable deflection of a laser beam, e.g. B. for automatic adjustment of the beam position. In the best case, the first order maximum ( ) used for this has over 90% of the beam intensity; the rest is lost (not distracted or higher orders)
- Amplitude modulation of the laser beam by deflecting it into an absorber. This is based on the fact that when the intensity of the sound wave is low, the deflected portion of the beam is proportional to the sound intensity.
- The introduction of periodic losses into a laser resonator for active mode coupling of the laser ensures pulsed operation.
-
Frequency shift
- Heterodyne interferometer ,
- Generation of ongoing interference patterns between the original laser beam and the laser beam frequency-shifted by the AOM,
- Applications in high-resolution spectroscopy and for manipulation of atoms in ion traps ,
- Laser Doppler anemometry to determine the direction of movement.
-
Frequency selection
- Selection of the color for multi-colored laser shows .
See also
literature
- Naumann, Schröder: Components of Optics. Paperback of technical optics. Fachbuchverlag Leipzig
- Frank L. Pedrotti, Leno S. Pedrotti, Werner Bausch: Optics for engineers. Basics. Springer, Berlin
- Helmbrecht Bauer: Laser technology: Basics and applications. Vogel publishing house, Würzburg
Individual evidence
- ↑ RE Silva, T. Tiess, M. Becker, T. Esch Rich, M. Rothhardt, M. Hunter, AAP Pohl, H. Bartelt: All-fiber acousto-optic modulator 10 MHz of a fiber Bragg grating at 1060 nm wavelength ' ( Page no longer available , search in web archives ) Info: The link was automatically marked as defective. Please check the link according to the instructions and then remove this notice. in Optical Society of America Vol. 23 (2015), No. 20, accessed Feb. 11, 2018
Web links
- Acousto-optic Modulators in the Encyclopedia of Laser Physics and Technology (engl.)
- Introduction to acousto-optics (PDF, English; 299 kB)