Photovoltaics
Photovoltaics, or PV for short, is a solar power technology that uses solar cells or solar photovoltaic arrays to convert energy from the sun into electricity. Photovoltaics is also the field of study relating to this technology.
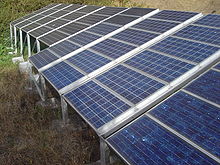
Solar cells produce direct current electricity from the sun’s rays, which can be used to power equipment or to recharge a battery. Many pocket calculators incorporate a solar cell.
When more power is required than a single cell can deliver, cells are generally grouped together to form “PV modules”, or solar panels, that may in turn be arranged in arrays. Such solar arrays have been used to power orbiting satellites and other spacecraft and in remote areas as a source of power for applications such as roadside emergency telephones, remote sensing, and cathodic protection of pipelines. The continual decline of manufacturing costs (dropping at 3 to 5% a year in recent years) is expanding the range of cost-effective uses including roadsigns, home power generation and even grid-connected electricity generation.
Large-scale incentive programs, offering financial incentives like the ability to sell excess electricity back to the public grid ("feed-in"), have greatly accelerated the pace of solar PV installations in Spain, Germany, Japan, the United States, Australia, South Korea, Italy, Greece, France, China and other countries.
Current development

Many corporations and institutions are currently developing ways to increase the practicality of solar power. While private companies conduct much of the research and development on solar energy, colleges and universities also work on solar-powered devices.
The most important issue with solar panels is cost. Because of much increased demand, the price of silicon used for most panels is now experiencing upward pressure. This has caused developers to start using other materials and thinner silicon to keep cost down. Due to economies of scale solar panels get less costly as people use and buy more — as manufacturers increase production, the cost is expected to continue to drop in the years to come. As of early 2006, the average cost per installed watt for a residential sized system was about USD 6.50 to USD 7.50, including panels, inverters, mounts, and electrical items.
Grid-tied systems represented the largest growth area. In the USA, with incentives from state governments, power companies and (in 2006 and 2007) from the federal government, growth is expected to climb. Net metering programs are one type of incentive driving growth in solar panel use. Net metering allows electricity customers to get credit for any extra power they send back into the grid. This causes an interesting role reversal, as the utility company becomes the buyer, and the solar panel owner becomes the seller of electricity. To spur growth of their renewable energy market, Germany has adopted an extreme form of net metering, whereby customers get paid 8 times what the power company charges them for any surplus they supply back to the grid. That large premium has created huge demand for solar panels in that country.
PV in buildings
Solar arrays are increasingly incorporated into new domestic and industrial buildings as a principal or ancillary source of electrical power. Typically, an array is incorporated into the roof or walls of a building, roof tiles can now even be purchased with an integrated PV cell (B.I.P.V.- Building Integrated PhotoVoltaics) . Arrays can also be retrofitted into existing buildings; in this case they are usually fitted on top of the existing roof structure. Alternatively, an array can be located separately from the building but connected by cable to supply power for the building.
Where a building is at a considerable distance from the public electricity supply (or grid) - in remote or mountainous areas – PV may be the only possibility for generating electricity, or PV may be used together with wind and/or hydroelectric power. In such off-grid circumstances batteries are usually used to store the electric power. However, the largest installations are grid-connected systems (see table below). These systems are connected to the utility grid through a direct current to alternating current (DC-AC) inverter. When the load required in the building is more than that supplied by the PV array then electricity will be drawn from the grid; conversely when the PV array is generating more power than is needed in the building then electricity will be exported to the grid. Batteries are not required and standard AC electrical equipment may be used. The average lowest retail cost of a large PV module declined from USD 7.50 to USD 4 per watt between 1990 and 2004. However, prices have gone up 15-20% in 2005-2006 due to increased demand (mainly due to increased incentives and subsidies) and silicon shortages. The silicon shortage is expected to persist until at least 2008. With many jurisdictions now giving tax and rebate incentives, and/or net metering solar electric power can now pay for itself in ten to twenty years in a few places.
In August 2006 there was widespread news coverage in the United Kingdom of the major high street electrical retailers (Currys) decision to stock PV modules, manufactured by Sharp, at a cost of one thousand pounds sterling per module. The retailer also provides an installation service. The agency that administers UK government grants for domestic solar power systems estimates that an installation for an average-sized house would cost between £8,000 and £18,000, and yield annual savings between £75 and £125. [1]
Example of PV in building
In the United Kingdom, the second tallest building in Manchester, the CIS Tower, was clad in PV panels at a cost of £5.5 million and started feeding electricity to the national grid in November 2005. [2]
Solar-powered vehicles
There is intensive research interest in solar-powered vehicles and the technology is developing rapidly. Solar-powered cars have commonly appeared at solar races such as the World Solar Challenge and at car and technology shows. Solar boats are a new application of the technology. Solar Boats from colleges and universities compete in the Solar Splash[1] competition in North America, and the Frisian Nuon Solar Challenge[2] in Europe.
PV power stations
Deployment of solar power depends largely upon local conditions and requirements. But as all industrialised nations share a need for electricity, it is clear that solar power will increasingly be used to supply a cheap, reliable electricity supply. In 2004 the worldwide production of solar cells increased by 60% but silicon shortages reduced growth afterwards.
The list below shows the largest photovoltaic plants in the world. For comparison, the largest non-photovoltaic solar plant, the solar trough-based SEGS in California produces 350 MW and the largest nuclear reactors generate more than 1,000 MW. A plant in Australia, which will not come into service until 2008, is expected to be 154 MW when it is completed by 2013.[3]
DC Peak Power | Location | Description | MW·h/year | Coordinates |
---|---|---|---|---|
154 MW*** | Mildura/Swan Hill, Australia [4] | Heliostat Concentrator Photovoltaic technology | n.a. | n.a. |
40 MW* | Muldentalkreis, Germany[5] | n.a. | n.a. | 51°19′43″N 12°39′20″E / 51.32861°N 12.65556°E |
20 MW*** | Beneixama, Spain[6][7] | Tenesol, Aleo and Solon solar modules with Q-Cells cells | 30,000 MW·h | 38°43′26″N 0°43′48″W / 38.72389°N 0.73000°W |
12 MW | Gut Erlasee, Germany[8] | 1408 SOLON mover | 14,000 MW·h | n.a. |
11 MW** | Serpa, Portugal | 52,000 solar modules | n.a. | n.a. |
10 MW | Pocking, Germany | 57,912 solar modules | 11,500 MW·h | n.a. |
6.3 MW | Mühlhausen, Germany[9] | 57,600 solar modules | 6,750 MW·h | 49°09′29″N 11°25′59″E / 49.15806°N 11.43306°E |
5.2 MW | Kameyama, Japan | 47,000 square meters on Sharp LCD factory roof | n.a. | 34°52′15″N 136°24′19″E / 34.87083°N 136.40528°E |
5 MW | Bürstadt, Germany | 30,000 BP solar modules | 4,200 MW·h | n.a. |
5 MW | Espenhain, Germany | 33,500 Shell solar modules | 5,000 MW·h | n.a. |
4.59 MW | Springerville, AZ, USA | 34,980 BP solar modules | 7,750 MW·h | 34°17′48″N 109°16′2″W / 34.29667°N 109.26722°W |
4 MW | Geiseltalsee, Merseburg, Germany | 25,000 BP solar modules | 3,400 MW·h | n.a. |
4 MW | Gottelborn, Germany | 50,000 solar modules (when completed) | 8,200 MW·h (when completed) | n.a. |
4 MW | Hemau, Germany | 32,740 solar modules | 3,900 MW·h | n.a. |
3.9 MW | Rancho Seco, CA, USA | n.a. | n.a. | 38°20′31″N 121°07′1″W / 38.34194°N 121.11694°W |
3.3 MW | Dingolfing, Germany | Solara, Sharp and Kyocera solar modules | 3,050 MW·h | n.a. |
3.3 MW | Serre, Italy | 60,000 solar modules | n.a. | n.a. |
* Project finish date: 2009
** Under construction, as of July 2006.Press Release
*** Under construction, as of summer 2007
Worldwide installed photovoltaic totals
Total peak power of installed solar panels is around 5,300 MW as of the end of 2005. (IEA statistics appear to be under-reported: they report 2,600 MW as of 2004, which with 1,700 installed in 2005 would be a cumulative total of 4,300 for 2005). The three leading countries (Japan, Germany and the USA) represent 90% of the total worldwide PV installations. A view of the deployments of solar power of all types is given at Deployment of solar power to energy grids.
Country | PV Capacity | ||||
---|---|---|---|---|---|
Cumulative | Installed in 2005 | ||||
Off-grid PV [kW] | Grid-connected [kW] | Total [kW] | Total [kW] | Grid-tied [kW] | |
Japan | 87,057 | 1,334,851 | 1,421,908 | 289,917 | 287,105 |
Germany | 29,000 | 1,400,000 | 1,429,000 | 635,000 | 632,000 |
United States | 233,000 | 246,000 | 479,000 | 103,000 | 70,000 |
Australia | 41,841 | 8,740 | 60,581 | 8,280 | 1,980 |
Spain | 15,800 | 41,600 | 57,400 | 20,400 | 18,600 |
Netherlands | 4,919 | 45,857 | 50,776 | 1,697 | 1,547 |
Italy | 12,300 | 15,200 | 37,500* | 6,800 | 6,500 |
* Original source gives these individual numbers and totals them to 37,500 KW. The 2004 reported total was 30,700 KW.[11] With new installations of 6,800 KW, this would give the reported 37,500 KW.
PV power costs
The table below shows the total cost in US cents per kWh of electricity generated by a photovoltaic system. The row headings on the left show the total cost, per peak kilowatt (kWp), of a photovoltaic installation. The column headings across the top refer to the annual energy output in kWh expected from each installed kWp. This varies by geographic region because the average insolation depends on the average cloudiness and the thickness of atmosphere traversed by the sunlight. It also depends on the path of the sun relative to the panel and the horizon.
Panels are usually mounted at an angle based on latitude, and often they are adjusted seasonally to meet the changing solar declination. Solar tracking can also be utilized to access even more perpendicular sunlight, thereby raising the total energy output. The calculated values in the table reflect the total cost in cents per kWh produced. They assume a 10% total capital cost (for instance 4% interest rate, 1% operating and maintenance cost, and depreciation of the capital outlay over 20 years). (Normally, photovoltaic modules have a 25 year warranty, but they should be fully functional even after 30-40 years.)
20 years | 2400 kWh/kWp y |
2200 kWh/kWp y |
2000 kWh/kWp y |
1800 kWh/kWp y |
1600 kWh/kWp y |
1400 kWh/kWp y |
1200 kWh/kWp y |
1000 kWh/kWp y |
800 kWh/kWp y |
---|---|---|---|---|---|---|---|---|---|
200 $/kWp | 0.8 | 0.9 | 1.0 | 1.1 | 1.3 | 1.4 | 1.7 | 2.0 | 2.5 |
600 $/kWp | 2.5 | 2.7 | 3.0 | 3.3 | 3.8 | 4.3 | 5.0 | 6.0 | 7.5 |
1000 $/kWp | 4.2 | 4.5 | 5.0 | 5.6 | 6.3 | 7.1 | 8.3 | 10.0 | 12.5 |
1400 $/kWp | 5.8 | 6.4 | 7.0 | 7.8 | 8.8 | 10.0 | 11.7 | 14.0 | 17.5 |
1800 $/kWp | 7.5 | 8.2 | 9.0 | 10.0 | 11.3 | 12.9 | 15.0 | 18.0 | 22.5 |
2200 $/kWp | 9.2 | 10.0 | 11.0 | 12.2 | 13.8 | 15.7 | 18.3 | 22.0 | 27.5 |
2600 $/kWp | 10.8 | 11.8 | 13.0 | 14.4 | 16.3 | 18.6 | 21.7 | 26.0 | 32.5 |
3000 $/kWp | 12.5 | 13.6 | 15.0 | 16.7 | 18.8 | 21.4 | 25.0 | 30.0 | 37.5 |
3400 $/kWp | 14.2 | 15.5 | 17.0 | 18.9 | 21.3 | 24.3 | 28.3 | 34.0 | 42.5 |
3800 $/kWp | 15.8 | 17.3 | 19.0 | 21.1 | 23.8 | 27.1 | 31.7 | 38.0 | 47.5 |
4200 $/kWp | 17.5 | 19.1 | 21.0 | 23.3 | 26.3 | 30.0 | 35.0 | 42.0 | 52.5 |
4600 $/kWp | 19.2 | 20.9 | 23.0 | 25.6 | 28.8 | 32.9 | 38.3 | 46.0 | 57.5 |
5000 $/kWp | 20.8 | 22.7 | 25.0 | 27.8 | 31.3 | 35.7 | 41.7 | 50.0 | 62.5 |
Kilowatt-hours per peak kilowatts per year at various locations[12]:
- Southern Germany: ~900-1,130 kWh per kWp per year
- Italy, Sicily: ~1,800 kWh per kWp per year
- South Spain: ~1,800 kWh per kWp per year
- China, Takla Makan: ~1,840 kWh per kWp per year
- U.S.A., Great Basin: ~1,930 kWh per kWp per year
- Spain, Canary Islands: ~2,000 kWh per kWp per year
- U.S.A., Hawaii: ~2,100 kWh per kWp per year
- Africa, Sahara: ~2,270 kWh per kWp per year
- Australia, Great Sandy: ~2,320 kWh per kWp per year
- Middle-East, Arabian: ~2,360 kWh per kWp per year
- South America, Atacama: ~2,410 kWh per kWp per year
- Equipment prices
- Polycrystalline modules (manufacturing costs): ~$2,000 / kWp
- Polycrystalline modules (commercial prices): from $3,490 up to $5,100 / kWp (8 m²/kWp)[13]
- Installation: from $600 up to $2,000 / kWp (self-construction: from $100 up to $400 / kWp)
- Inverter for grid feed-in: ~$400 /kWp[14]
Environmental impacts
Unlike fossil fuel based technologies, solar power does not lead to any harmful emissions during operation, but the production of the panels leads to some amount of pollution.
Greenhouse gases
Life cycle greenhouse gas emissions are now in the range of 25-32 g/kWh and this could decrease to 15 g/kWh in the future; though, this is speculative. [15] For comparison, a combined cycle gas-fired power plant emits some 400 g/kWh and a coal-fired power plant with Carbon capture and storage some 200 g/kWh. Nuclear power emits 25 g/kWh on average; only wind power is better with a mere 11 g/kWh.
Cadmium
One issue that has often raised concerns is the use of cadmium in Cadmium telluride (CdTe) modules (not all PV technologies use CdTe). Cadmium in its metallic form is a toxic substance that has the tendency to accumulate in ecological food chains. The amount of cadmium used in PV modules is relatively small (5-10 g/m2) and with proper emission control techniques in place the cadmium emissions from module production can be almost zero. Current PV technologies lead to cadmium emissions of 0.3-0.9 microgram/kWh over the whole life-cycle.[15] Most of these emissions actually arise through the use of coal power for the manufacturing of the modules, and coal and lignite combustion leads to much higher emissions of cadmium. Life-cycle cadmium emissions from coal is 3.1 microgram/kWh, lignite 6.2, and natural gas 0.2 microgram/kWh.
Energy return on investment
A key indicator of environmental performance is the ratio of electricity generated divided by the energy required to build and maintain the equipment. Of course, little is gained if it takes as much energy to produce the modules as they produce in their lifetimes. This ratio is called the energy return on investment (EROI) This should not be confused with the economic return on investment, which varies according to local energy prices, subsidies available and metering techniques. A related concept is the energy pay-back time, i.e. the time required to produce an amount of energy as great as what was consumed during production.
Crystalline silicon PV systems presently have energy pay-back times of 1.5-2 years for South-European locations and 2.7-3.5 years for Middle-European locations. For silicon technology clear prospects for a reduction of energy input exist, and an energy pay-back of 1 year may be possible within a few years. Thin film technologies now have energy pay-back times in the range of 1-1.5 years (S.Europe).[15] With lifetimes of such systems of at least 30 years, the EROI is in the range of 10 to 30.
Alternative theories of energy accounting provide a more pessimistic calculation for EROI of photovoltaics. Such theories, such as Howard Odum's "Emergy" concept, or Price-Estimated EROI calculations [4], arrive at EROI closer to 1:1 for photovoltaics.
Grid parity
Grid parity is already reached in some regions. This means photovoltaic power is equal to or cheaper than grid power. Grid parity has been reached in Hawaii and many other islands that use diesel fuel to produce electricity.
In Italy PV power is cheaper than retail grid electricity since 2006. One kWh costs 21.08 -€cent/kWh. Italy has an average of 1,600 kWh/m2 (Sicily even 1,800 kWh/m2) sun power/year. At 4 % costs of capital, 25 years of depreciation and costs (including installation) of 4,600 €/kWp PV current costs are 20.91 €-cent/kWh. At large scale plants with 3,900 €/kWp the costs reduces to 17.75 €-cent/kWh and is 15 % cheaper. To reach a 19% PV power coverage in Italy, 34,000 MWp power must be installed. This means 0.09 % of the size of Italy. 9 % of the size of Sicily could produce 25 % of the power of the complete European Union (ca. 2,100 TWh/year).
Financial incentives
The political purpose of incentive policies for PV is to grow the industry even while the cost of PV is significantly above grid parity, to allow it to achieve the economies of scale necessary to reach grid parity. The policies are implemented to promote national energy independence, high tech job creation and reduction of CO2 emissions
Two incentive mechanisms are used:
- investment subsidies:the authorities refund part of the cost of installation of the system,
- feed in tariffs/net metering: the electricity utility buys PV electricity from the producer under a multiyear contract at a guaranteed rate.
With investment subsidies, the financial burden falls upon the taxpayer, while with feed-in tariffs the extra cost is distributed across the utilities' customer base. While the investment subsidy may be simpler to administer, the main argument in favour of feed in tariffs is the encouragement of quality. Investment subsidies are paid out as a function of the nameplate capacity of the installed system and are independent of its actual power yield over time, so reward overstatement of power, and tolerate poor durability and maintenance. Feed in tariffs reward the number of kWh produced over a long period of time.
The price paid per kWh under a feed in tariff exceeds the price of grid electricity. "Net metering" refers to the case where the price paid by the utility is the same as the price charged.
The Japanese government through its Ministry of International Trade and Industry ran a successful programme of subsidies from 1994 to 2003. By the end of 2004, Japan led the world in installed PV capacity with over 1.1 GW.[5]
In 2004, the German government introduced the first large scale feed in tariff system, under a law known as the 'EEG' (see below) which resulted in explosive growth of PV installations in Germany. The principle behind the German system is a 20 year flat rate contract. The value of new contracts is programmed to decrease each year, in order to encourage the industry to pass on lower costs to the end users.
Subsequently Spain, Italy, Greece and France introduced feed in tariffs. None have replicated the programmed decrease of FIT in new contracts though, making the German incentive relatively less and less attractive compared to other countries. The French FIT offers a uniquely high premium for building integrated systems.
In 2006 California approved the 'California Solar Initiative', offering a choice of investment subsidies or FIT for small and medium systems and a FIT for large systems. Incentives are scheduled to decrease in future depending as a function of the amount of PV capacity installed.
The price/kWh or kWp of the FIT or investment subsidies in stimulating the installation of PV is only one of three factors. The other two are insolation (the more sunshine, the less money is needed) and administrative ease of obtaining permits and contracts (Southern European countries are reputedly relatively complex)
The most significant incentives programs are listed here.
Germany
Situation as of 2006. [6]
The legal framework is the German Renewable Energy Sources Act (Erneuerbare-Energien-Gesetz – EEG), amended version in force since 1 August 2004.
Feed in Tariffs:
- Roof mounted <= 30 kWp : EUR 0.5180/kWh
- Roof mounted 30 kWp to 100kWp: EUR 0.4928/kWh
- Roof mounted over 100kWp: EUR 0.4874/kWh
- Facade integrated as above + EUR 0.0500/kWh
- Field installation EUR 0.4060/kWh
Contract duration 20 years, constant remuneration. New contracts will be 5% lower in value in 2007 (6.5% for field installations).
Spain
Situation as of 2006.
The legal framework is the Real Decreto (royal decree) 436/2004.
Feed in Tariff:
- EUR 0.4214/kWh
Contract duration 25 years, linked to electricity price.
Greece
Situation as of 2006. [7]
Feed in Tariffs:
- Mainland <= 100 kWp 0.45 €/kWh
- Mainland > 100 kWp 0.40 €/kWh
- Islands <= 100 kWp 0.50 €/kWh
- Islands > 100 kWp 0.45 €/kWh
Contract duration 20 years, linked to inflation
Investment subsidies: Tax rebates and grants are available.
France
Situation as of 2006. [8]
Feed in Tariffs (mainland, excluding DOM-TOMs):
- EUR 0.30/kWh
- Roof integrated EUR 0.55/kWh
Contract duration 20 years, linked to inflation.
Additional investment subsidies available as tax credits.
Italy
Situation as of 2006.[9]
The legal framework is the Ministry for Industry decree issued on 5th August 2005.
Feed in Tariffs:
- at least EUR 0.445/kWh (too many variants to list here)
Contract duration 20 years, linked to inflation.
United States
California
Starting 1 Jan 2007[10]
Feed in Tariffs and Investment subsidies :
- Systems >100 kWp: $0.39/kWh
- Systems <100 kWp can choose either $2.50/kWp or $0.39/kWh
Additional investment subsidies available as federal tax credits.
Contract duration 5 years, constant remuneration
S Korea
as of Oct 11 2006
Feed in Tariffs:
- Systems >30 kWp: KRW677.38/kWh
- Systems <30 kWp: KRW711.25/kWh (ca $0.75, €0.60)
Additional subsidies available.
Contract duration 15 years, constant remuneration
Photovoltaics research institutes
There are many research institutions and departments at universities around the world who are active in photovoltaics research. Countries which are particularly active include Germany, Spain, Japan, Australia, China, and the USA.
Some universities and institutes which have a photovoltaics research department.
- National Renewable Energy Laboratory NREL
- Institut für Solare Energiesysteme ISE at the Fraunhofer Institute
- Imperial College London: Experimental Solid State Physics
- Instituto de Energía Solar, at Universidad Politécnica de Madrid
- Centre for Renewable Energy Systems Technology, at Loughborough University
- School of Photovoltaic and Renewable Energy Engineering at The University of New South Wales
- Centre for Sustainable Energy Systems at the Australian National University
- Ecole Polytechnique Fédérale de Lausanne
- Advanced Energy Systems at Helsinki University of Technology
- The Centre for Electronic Devices and Materials at Sheffield Hallam University
- The Solar Caliometry Lab at Queen's University
- Energy & Environmental Technology Application Center at the College of Nanoscale Science and Engineering SUNY at Albany
- Institute of microtechnology at University of Neuchatel Switzerland
- University of Konstanz
- Arizona State University Photovoltaic Testing Laboratory
- Institute of Energy Conversion at University of Delaware
- World Alliance for Decentralized Energy
References
- ^ BBC online news 1st August 2006
- ^ Building converts to solar power
- ^ World's largest photovoltaic power plants
- ^ 154MW Victoria (Australia) Project
- ^ Large photovoltaic plant in Muldentalkreis
- ^ Large photovoltaic plant in Beneixama
- ^ [http://city-solar-ag.com/index.php?id=185 Photovoltaic plant in Beneixama
- ^ The largest photovoltaic plant
- ^ Solarpark Bavaria
- ^ Table 1: Installed PV power in reporting IEA PVPS countries as of the end of 2005
- ^ Total photovoltaic power installed in IEA PVPS countries
- ^ Solar land area
- ^ €3,20/kWp = $4,16/kWp
- ^ Inverter and module prices
- ^ a b c Alsema, E.A.; Wild - Scholten, M.J. de; Fthenakis, V.M. Environmental impacts of PV electricity generation - a critical comparison of energy supply options ECN, September 2006; 7p. Presented at the 21st European Photovoltaic Solar Energy Conference and Exhibition, Dresden, Germany, 4-8 september 2006.
External links
- How Stuff Works: Solar cells.
- Energy Atlas of the West
- World's largest photovoltaic power plants
- Global Solar Completed 1.4 MW Solar Power Station; Signs Agreement to Enlarge System to 2.4 MW
- Solarbuzz
- Trends in photovoltaic applications in selected IEA countries between 1992 and 2004
- Information pertaining to photovoltaic solar electricity in each of the IEA PVPS member countries
- Photon Magazine International PV magazine
- Renewable Energy World magazine
- Home Power Magazine
- Power Consumption of a Home
- US Department of Energy Energy Efficiency and Renewable Energy
- DSIRE Listing of US state, local, utility, and federal incentives for renewable energy and energy efficiency.
- SEIA: Solar Energy Industries Association US trade association of solar energy manufacturers, dealers, distributors, contractors
- EPIA: European Photovoltaic Industry association
- JPEA - Japanese Photovoltaic Energy Association in Japanese only
- BSW: German Solar industry Association
See also
- Active solar
- California Solar Initiative
- Green technology
- Microgeneration
- Photoelectrochemical cell
- Photovoltaic components, projects and howtos
- Renewable energy
- Solar thermal
- Solar power
- Solar cell
- Solar panel
Template:Energy Conversion
Template:Sustainability and energy development group