Scanning electron microscope


As a scanning electron microscope ( SEM ) ( English scanning electron microscope , SEM ) is called an electron microscope in which an electron beam in a specific pattern is increased over the object to be imaged is performed (rasterized) and interactions of the electrons used with the object of forming an image of the object become. The images typically generated with a scanning electron microscope are images of the object surfaces and have a high depth of field . A scanning-based imaging can also be in transmission perform (Engl. Scanning transmission electron microscopy , STEM), this suitably equipped are transmission electron microscopes or dedicated scanning transmission electron microscopes needed.
history
Hans Busch discovered in 1925 that a magnetic field can be used as an electron lens, analogous to the glass lens in light rays. In 1931 Ernst Ruska built the first electron microscope together with Max Knoll . It was, however, a transmission electron microscope (transmission electron microscope - TEM) and did not provide images of the surface, but the distribution of the mass in the object. The resolution of this first electron microscope was initially very limited for technical reasons. Two years later Ernst Ruska constructed his second electron microscope with a resolution of 50 nm, which by far exceeds the resolution with light beam scanning.
The scanning electron microscope was invented by Manfred von Ardenne in 1937 . He developed and built the first high-resolution scanning electron microscope with high magnification and scanning of a very small grid (side length 10 µm; resolution in line direction 10 nm) with a two-stage reduced and finely focused electron beam (probe diameter 10 nm). Von Ardenne used the scanning principle not only to open another avenue in electron microscopy, but also specifically to eliminate the chromatic error inherent in electron microscopes. In his publications he described and discussed the theoretical principles of the scanning electron microscope and the various detection methods, and shared his practical implementation. Further work came from the Vladimir Zworykin group (1942), later from the Cambridge groups in the 1950s and early 1960s under the direction of Charles Oatley. All of this work eventually led to the marketing of the first commercial scanning electron microscope "Stereoscan" (1965) by Cambridge Scientific Instruments Company. An account of the early history of SEM was written by McMullan.
Working principle

Electron beam generation
The electron beam is generated in an electron source. The simpler devices are a hairpin- shaped bent wire made of tungsten or a LaB 6 crystal ( lanthanum hexaboride ). This is heated and emits electrons (so-called hot cathode ), which are then accelerated in an electric field with a voltage of typically 8 to 30 kV.
Field emission technology is used in more expensive devices. The field emission cathode (engl. Field emission gun , FEG) is composed of a very fine tip, from which, by applying a very high electrical field strength, the electrons "tunnel out". A distinction is made between cold field emission, in which the electrons emerge from a fine tungsten tip without heating the cathode, only due to the applied electric field , and thermal field emission, in which a Schottky cathode is slightly heated. The thermal field emission has the advantage of higher beam intensity. Instruments with such electron sources are characterized by particularly good image quality even at a very low acceleration voltage. The reason for the better image quality is that the electrons have a defined speed.
Raster process
The scanning electron microscope is based on the scanning of the object surface using a finely focused electron beam. The entire process usually takes place in a high vacuum to avoid interactions with atoms and molecules in the air.
With the help of magnetic coils of the electron beam is at a point on the object is focused . When the electron beam hits the object, various interactions are possible, the detection of which provides information about the nature of the object. The intensity of the signal is evaluated.
The primary electron beam coming from the cathode is now guided line by line over the surface of the object like a tube television (raster), while the signal is converted into gray value information and displayed synchronously on the screen. When all lines of the image have been scanned, the rastering starts again at the upper edge of the image and a new image is generated.
The magnification is nothing else than the ratio between the scanned sample area and the monitor size. The magnification can be adjusted almost continuously on most devices.
Signal types
Secondary electron contrast
The most widely used source of information are the secondary electrons (SE) generated by the electrons of the beam (primary electrons) in interaction with the atoms of the object to be examined . They have an energy of a few electron volts and can be detected by an Everhart-Thornley detector (ETD) or by a so-called inlens detector (detector is located in the column). Due to their low energy, they only come from the topmost nanometers of the surface and thus depict the topography of the object. SE from deeper layers do not reach the surface and are therefore not detected. The volume from which the SEs are captured is therefore much smaller than the area excited by the primary beam. The resolution is almost exclusively determined by the achievable beam diameter and this is comparatively small - therefore SE images allow a very high resolution (a few nm).
The effective image contrast depends on more parameters than with a light microscope . Areas that are inclined towards the detector appear lighter than areas that are turned away from the detector (surface inclination contrast). There are also other contrast mechanisms, such as edge contrast or shadow contrast . In general, the impression is created as if the object is being viewed from above while it is illuminated from the direction of the detector. The samples are therefore often selectively etched to increase the contrast.
The SE yield also depends on the material (due to charges, the atomic number and the chemical bond ). By detaching the SE and the lack of grounding, electrically insulating materials such as B. oxides are positively charged and thereby prevent further detachment of the electrons. This makes them appear darker than a metallic environment. In principle, heavy materials appear lighter than light ones. The inlens detector in particular shows more material contrast, while the Everhart-Thornley detector is more sensitive to topographies due to its lateral position.
Backscattered electron contrast
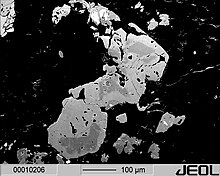
Another commonly used method of imaging is the detection of back-scattered electrons (engl. Backscattered electrons , BSE). These primary electrons backscattered from the object are more energetic than secondary electrons and have a typical energy of a few keV. The intensity of the signal depends primarily on the mean atomic number of the material. Heavy elements create a strong backscatter, so that corresponding areas appear bright. Areas with lighter elements, however, appear darker. The BSE image is therefore also referred to as a material contrast image and enables conclusions to be drawn about the chemical nature of the object material or the distribution of different materials or elements in the image.
When interpreting material contrast images, it should also be noted that the topography of the sample (surface inclination, shadowing, charging , etc.) can also influence the contrast and simulate material inhomogeneities. The interaction volume from which electrons are backscattered depends not only on the material of the examined sample (atomic number) but also on the acceleration voltage. It is typically a few cubic micrometers - therefore BSE images have a poorer resolution than secondary electron images.
The Everhart-Thornley detector can be used to detect the backscattered electrons . If the voltage on the detector grid is negative, only the more energetic backscattered electrons can reach the detector, the secondary electrons are shielded. Since the Everhart-Thornley detector is located on the side of the sample, the yield of backscattered electrons is relatively low. A dedicated backscattered electron detector is the Robinson detector , in which a scintillator with a hole is placed over the sample so that the primary electron beam hits the sample through the hole. More modern detectors are semiconductor-based, often have several segments and are placed directly on the pole piece. Another design are detectors integrated directly into the column ("InLens" detector).
In the meantime there are also technical solutions (electron optics, detectors) that allow backscatter contrast to be used for imaging at very low acceleration energies (1 keV and below). Because of the small interaction volume with these energies, a significantly better spatial resolution is obtained.
X-ray analysis (EDX / WDX)
Characteristic X-rays are often used in the SEM to characterize the elemental composition of the smallest sample areas. This occurs when an electron of the electron beam in the atom of the sample knocks an electron close to the nucleus out of its position. This gap is immediately filled by a more energetic electron from a higher orbital. The energy difference is released in the form of an X-ray quantum. The resulting X-rays are characteristic of the transition and the atom, i.e. the element.
By means of suitable detectors ( semiconductor detectors ), the energies, the intensity of which is characteristic of the elements contained in the sample, can be recorded and thus inferred directly about the element. The common method on REM is the energy dispersive X-ray analysis (Engl. Energy dispersive X-ray analysis , EDX) - while the energy of the X-ray quantum is evaluated. At some SEMs there is also the wavelength dispersive X-ray analysis ( " wavelength dispersive X-ray analysis ," WDX), finds but mainly (e-beam) microprobe use: see electron probe microanalysis (EPMA).
Other types of signals
- Sample stream : absorbed electrons generate / represent a current that flows through the sample to the earth and can be used to map the surface.
- Cathodoluminescence: Cathodoluminescence arises from the fact that some substances emit light when exposed to electrons. This is imaged with an elliptical concave mirror, since an ellipse has two focal points. The sample is located in one of the two focal points and the detector unit in the other. The light can be spectrally broken down and therefore provides information about ranges of different wavelengths. For this purpose, a wave-selective image is generated. With the help of cathodoluminescence radiation, information on internal and defect structure as well as trace elements can be obtained.
- Auger electrons: Another mechanism of interaction is the generation of Auger electrons . Auger electrons can be evaluated using additionally connected spectrometer devices.
- EBSD: With the help of electron backscatter diffraction (. EBSD of engl electron back scatter diffraction ) there is the crystallographic determine orientation of crystals on the object surface. This is of great importance for the characterization of material properties in materials science and geology, for example. For this purpose, the electrons reflected from the crystal surfaces of the object are projected onto a detector screen and the resulting Kikuchi lines are analyzed with the aid of a computer and assigned to crystallographic directions.
Sample requirements and sample preparation
The sample must be vacuum-stable, since the examination takes place in a high vacuum or, with ESEM, in a slight vacuum.
Charging effects are a major problem when examining insulators. If the energy of the electrons is too low, only very few secondary electrons are emitted and the sample is locally negatively charged. If the primary beam is too strong, parts of the surface can become positively charged. To avoid these effects, insulating materials can be provided with a very thin layer of noble metal (e.g. gold, platinum, platinum-palladium mixtures or chrome sputtering ) or with carbon (graphite) vapor-deposited. An alternative is the use of acceleration voltages at which current equilibrium prevails (irradiated electron current = emitted electron current). The values for this are usually below 3 kV and must be found individually for each sample. A third possibility is to tilt the sample more strongly and to attach a positive suction electrode, ideally above the sample. Then you can use a common, laterally arranged secondary electron detector to image many insulator samples without coating.
Variants of scanning electron microscopy
ESEM
A variant of the scanning electron microscope is the ESEM ( environmental scanning electron microscope , ESEM), in which only the electron beam is generated in a high vacuum. The sample chamber and the electron-optical column in which the beam manipulation is located are only under a slight vacuum. The residual gas in the chamber acts as an oscillator and amplifier . In addition, the residual gas ensures charge compensation, so that the samples do not need to be coated.
STEM
The scanning transmission electron microscope (STEM) is a special variant of the transmission electron microscope . With this method, the detector is located behind the sample (viewed in the direction of the electron beam). The scattering of the electrons in transmission is measured. To do this, the sample must be very thin (typically between 50 and 500 nm). For some time there have also been semiconductor detectors for scanning electron microscopes.
SEMPA
The scanning electron microscope with polarization analysis (SEMPA) is a special variant of the scanning electron microscope. With this method, not only the number but also the spin of the secondary electrons (SE) in the detector is analyzed. Here, two components of the electron spin are measured simultaneously. If a magnetic sample is examined, the emerging secondary electrons are spin-polarized. An image of the magnetic domain structure of the sample surface can be obtained by a location-dependent investigation of the spin polarization of the SE.
Comparison with other microscopic techniques
The images generated with a scanning electron microscope are images of the object surfaces and have a greater depth of field compared to images generated with light-optical transmitted light microscopes . Apart from the beam diameter, the resolution is of course strongly dependent on the sample and the selected image signal and is typically around 1 nm ... 2 nm under favorable conditions. The maximum useful magnification factor is around 1,000,000: 1, while it is around 2000: in light microscopy: 1 lies.
Compared to the transmission electron microscope , the scanning electron microscope achieves a lower resolution. However, when preparing the sample for transmission electron microscopy, the sample is greatly changed, since the preparation has to be very thin. In contrast, the sample remains mechanically intact with the scanning electron microscope.
See also
- Atomic Force Microscope (AFM)
- Scanning tunnel microscope (RTM or STM)
- Helium ion microscope
- microscope
- Scanning probe microscopy
- Imaging procedure
- microscopy
- Microphotography and macro photography
- Ultramicrotome
- X-ray fluorescence analysis
literature
- Patent GB511204 : Improvements in electron microscopes. Published on August 15, 1939 , inventor: Manfred von Ardenne.
- Manfred von Ardenne: The scanning electron microscope. Theoretical foundations . In: Journal of Physics . 109, No. 9-10, 1938, pp. 553-572. bibcode : 1938ZPhy..109..553V . doi : 10.1007 / BF01341584 .
- Manfred von Ardenne: The scanning electron microscope. Practical execution . In: Journal for Technical Physics . 19, 1938, pp. 407-416.
- D. McMullan: Scanning electron microscopy 1928-1965 . In: Scanning . tape 17 , no. 3 , 1995, p. 175-185 , doi : 10.1002 / sca.4950170309 .
- D. McMullan: Von Ardenne and the scanning electron microscope . In: Proceedings of the Royal Microscopical Society . tape 23 , 1988, pp. 283-288 .
- Joseph Goldstein et al .: Scanning Electron Microscopy and X-ray microanalysis . 3rd edition, Springer, New York 2003, ISBN 978-0-306-47292-3 .
- Stanley L. Flegler, John William Heckman, Karen L. Klomparens: Electron Microscopy - Fundamentals, Methods, Applications . Spectrum Academic Publishing House, 1995, ISBN 3-86025-341-7 .
- Ludwig Reimer, Gerhard Pfefferkorn: Scanning electron microscopy. 2., ext. Edition Springer, Berlin 1999, ISBN 3-540-08154-2 .
- Karl-Heinz Scharf, Wilhelm Weber: Cytology . Revised edition, ISBN 3-507-10524-1 .
- Frank Eggert: Standard-free electron beam microanalysis with the EDX in the scanning electron microscope . BoD, Norderstedt, 2005, ISBN 3-8334-2599-7 .
Web links
- Images from a scanning secondary electron microscope , (labeled “SEM”); remf.dartmouth.edu
- Images from a scanning electron microscope
- Information on the topics of X-ray microanalysis in the scanning electron microscope and EDX; www.mikroanalytik.de
- Scanning electron microscopy video
Individual evidence
- ↑ Alexander Linnemann, Susanne Kühl: Fundamentals of light and electron microscopy 2007, p. 340.
- ^ The Robinson detector - ESEM Science and Technology . Retrieved December 26, 2018.
- ↑ Upgrade Info AsB Detector . Carl Zeiss Microscopy GmbH. Retrieved December 26, 2018.
- ↑ Robert Frömter, Sebastian Hanke Meier, Hans Peter Oepen, Jürgen Kirschner: Optimizing a low-energy electron diffraction spin polarization analyzer for imaging of magnetic surface structures . In: Review of Scientific Instruments . tape 82 , no. 3 , 2011, doi : 10.1063 / 1.3534832 .
- ↑ Fabian Lofink, Sebastian Hankemeier, Robert Frömter, Jürgen Kirschner, Hans Peter Oepen: Long-time stability of a low-energy electron diffraction spin polarization analyzer for magnetic imaging . In: Review of Scientific Instruments . tape 83 , no. 2 , 2012, doi : 10.1063 / 1.3685629 .
- ↑ High resolution scanning electron microscope (SEM). (PDF; 99 kB) Competence Center for Materials in Microelectronics, Ulm University, accessed on March 23, 2010 .