Chromosome territory

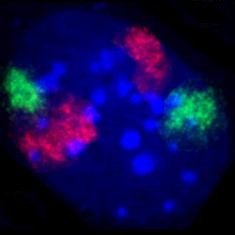
A chromosome territory is the area within a cell nucleus that is occupied by a chromosome . Chromosome territories have a variable shape that differs not only between different chromosomes, but also from nucleus to nucleus for the same chromosome. They are not solid structures; other molecules such as proteins can diffuse through them . The arms of chromosomes and individual chromosome bands also form definable areas. Chromosome territories are dynamic structures whose genes can be shifted at the onset of transcription .
history
As early as the 1880s, Carl Rabl had proposed for the first time that chromosomes between two nuclear divisions ( mitosis ) do not mix, but are also separated from one another in the cell nucleus of the interphase . He suggested the term territory for the area of a chromosome. Rabl's views on this were supported by Theodor Boveri at the beginning of the 20th century. When, in the later course of the 20th century, electron microscopy allowed insights into biological specimens with previously unknown resolution, it was not possible to observe the boundaries between the chromatin of individual chromosomes in the cell nucleus . As a result, the view that chromatin threads from the various chromosomes mix strongly within the cell nucleus, similar to long spaghetti on a plate , has become increasingly popular . Evidence that chromosomes do form territories emerged in the 1970s from experiments in which cell nuclei were irradiated with UV lasers. In these experiments, only a small part of the nucleus of living cells was irradiated. If chromosomes had actually been distributed throughout the nucleus like spaghetti, all or at least most of the chromosomes would have had to be hit during the irradiation. In the mitosis that followed the irradiation, however, it was shown that only a few chromosomes showed radiation damage, but the majority were intact. This spoke in favor of a regionally limited spread of the chromosomes in the cell nucleus. A direct visualization of chromosome territories was possible about ten years later with the help of fluorescence in situ hybridization (see also figures).
Occurrence
Detection of chromosome territories requires that probes be available for fluorescence in situ hybridization that contains the exact DNA of a chromosome. Such probes are complex to manufacture and are therefore only available for a few types . Chromosome territories were found in cell nuclei in humans and in all animals and plants examined . The species studied include a number of primates , mice ( house mouse and others), and the domestic chicken . Detection in plants was difficult because no suitable probes were available for a long time. Here too, chromosome territories could ultimately be detected, for example in the thale cress ( Arabidopsis thaliana ), which is often used for experiments . The further distribution among the eukaryotes is not certain. In organisms with small chromosomes, such as the yeast Saccharomyces cerevisiae , detection is hardly possible due to the small chromosome size and the limited resolution of the light microscope. It is conceivable that with such small chromosomes a mixing of the chromatin threads of different chromosomes takes place and that there is no formation of territories.
arrangement
The arrangement of the chromosome territories in the cell nucleus is very variable. However, when many nuclei are examined, it can be seen that certain patterns are more common than others. The territories of gene-rich chromosomes are more often found in the middle of the nucleus than those of poorer-gen territories. Such a non-random radial distribution of the territories has been described, for example, in human lymphocytes , in which the similarly sized, but very gene-rich or very gene-poor chromosomes 18 and 19 were examined. These observations of a radial arrangement in the nucleus as a function of gene density were confirmed by analyzes covering all human chromosomes. However, there are pronounced cell-to-cell variations in the neighborhood arrangements of these territories in all cell types investigated to date. This gene-density-dependent distribution of chromosome territories was also found in cell nuclei of mice, so that it was conserved over at least a period of 60 million years. This speaks for a significant advantage of this arrangement.
The "bodyguard" hypothesis of TC Hsu tries to answer the question of the biological significance of this arrangement. It says that gene-rich material inside the cell nucleus is protected from external damage. Hsu assumed that low-gene, constitutive peripheral heterochromatin can protect the euchromatin from chemical mutagens and X-rays , since its position is adjacent to the nuclear membrane during the interphase . It is therefore positioned in such a way that it absorbs more environmental risks than central euchromatin and can thus protect the DNA there from damage and mutations. The low-gene chromosome sections at the edge of the cell nucleus could also serve as protection against external mechanical influences.
With the accumulation of many active genes inside the cell nucleus, common regulation could play a role by sharing transcription machineries, splicing factors, etc. Inside the cell nucleus, transcription factors have been found that find their genes in close proximity. This would allow expression to be regulated more easily and more efficiently.
mechanism
There are various explanations for this mechanism, which is responsible for this special arrangement of chromatin in the cell nucleus. The biophysical concept of "macromolecular crowding" by Zimmerman and Minton states a change in the molecular interactions in response to a change in the crowding conditions. "Crowding" is defined here as the occurrence of macromolecules that occupy a certain volume. You reduce the available volume for other molecules. The originally widely distributed molecules form compact compartments in a system overflowing with molecules. Denser compaction results in a less precluded volume in which the molecules can move. This increases the entropy of the entire system. The binding of heterochromatin to the lamina of the nucleus could also play a role in the arrangement of chromatin in the nucleus. If this mechanism is disturbed, serious diseases can occur.
construction
The DNA is not present in the cell nucleus as a free molecule, but as chromatin . In the first packaging step, it is packed into nucleosomes with the help of histones . The folding of the DNA into nucleosomes results in a 6-fold shortening. Variants of histones that may be present in nucleosomes, post-translational modifications, and other markings can control DNA accessibility for transcription at this packaging level. Further chromatin condensation to 30 nm fibers (i.e. in zigzag or solenoid shapes) has been suggested but is not certain. The structure and organization of higher order chromatin loops within a chromosome territory is also unclear.
There is evidence that the chromatin is packaged in higher order units, each ~ 1 Mb in length. Individual ∼1-Mb domains could be composed of smaller loop domains, while larger chromatin clusters could consist of clusters of several ∼1-Mb domains. Chromosome territories visualized by fluorescence in situ hybridization (3D-FISH) appear as structures with diverse shapes made up of higher-order chromatin domains.
The ∼1 Mb chromatin domains could be the basic structural units that make up chromosome territories. These domains were first represented in S-phase nuclei as centers of replication and later turned out to be permanent, higher-order chromatin structures. So far, neither their ultrastructural organization nor the packaging of chromatin that connects these areas has been fully clarified.
Chromosome territories can be shown to be divided into areas of early DNA replication. These have genetically dense 1Mb domains that are located inside the cell nuclei. Around them are poorer sections with a middle to late replication time. These are more on the core periphery and have contact with the core lamina . Around the nucleolus there are also poorer 1Mb domains with a late replication time. A hierarchy of chromatin fibers could be responsible for this higher order within the cell nucleus.
Models
With the development of biochemical high-throughput techniques such as 3C (" Chromosome conformation capture" ) and 4C ("Chromosome conformation capture-on-chip" and "circular chromosome conformation capture") numerous spatial interactions between neighboring chromosomal segments have been described. These descriptions were supplemented by the creation of spatial maps for the entire genome. Together with the data from fluorescence in situ hybridizations, these observations and physical simulations have led to different models of the spatial organization of chromosome territories.
Both the ICN model and the CT-IC models are able to explain functional chromatin interactions. Both models can also explain intra- and interchromosomal rearrangements. Researchers prefer the chromatin domain view for the core organization, as provided by the CT-IC model. This is based on the belief that this model best fits the current experimental evidence. Although the models presented here do not agree on some points, they are by no means mutually exclusive.
Interchromosome Domain Model (ICD)
In the Interchromosome Domain Model (ICD) from 1993, chromosome territories are separated from the so-called interchromatin area by a smooth surface. This area forms a network of branched channels that extend from the nuclear pores over the entire cell nucleus. Factors for transcription, DNA repair and splicing of the RNA are located in these channels.
Active genes are in direct contact with the ICD compartment because they are on the surface of chromosome territories or the intrachromosomal channels. Rare chromatin loops that extend from chromosome territories can invade the ICD space, which is likely to contain little or no chromatin. Further observations that transcription also occurs within chromosome territories led to the questioning of this model, which represents the processes too simplified.
Chromosome territory - Interchromatin compartment (CT-IC)
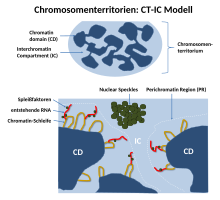
Since it was observed that transcription is not limited to the edge of a chromosome territory, Thomas and Christoph Cremer introduced the “chromosome territory - interchromatin compartment” model (CT-IC model). To this end, they further developed the ICD model. This model describes two areas in a cell nucleus: chromosome territories ( CTs ) and the interchromatin compartment ( IC ). In this model, chromosome territories build an interconnected chromatin network that is connected to an adjacent space, the interchromatin compartment. The latter can be observed with the help of both light and electron microscopy.
The IC consists of a DNA-free or at least largely free, contiguous channel space that begins at the nuclear pores and widens through larger channels and gaps between the higher-order chromatin network described above. The IC houses nuclear speckles and a large number of other nuclear bodies. Within a single chromosome territory, the chromosome is divided into defined areas based on the degree of chromosome condensation. Here the inner part of the interphase chromosome consists of more condensed chromatin domains or higher order chromatin fibers. These consist of several ~ 1Mb large DNA domains that correspond to the replication centers. These so-called 1Mb domains should in turn consist of many small 30-200kb-containing DNA loops. Between these 1Mb domains and the interchromatin compartment there is a thin (<200 nm) layer of decondensed chromatin called the perichromatin region (PR). The chromosome territories themselves are also traversed here by the channels of the interchromatin compartment. As could be shown on the basis of electron micrographs, the perichromatin regions consist of decondensed chromatin loops. As a result, they form a sponge-like structure and are therefore accessible to the proteins of the interchromatin compartment.
Functionally, the perichromatin region is the most important transcription compartment. This is also the region in which RNA splicing takes place. The DNA replication and DNA repair is also mainly carried out region within the Perichromatin. Finally, RNA transcripts, so-called perichromatin fibrils, generated in the perichromatin area are also produced. Perichromatin fibrils are then spliced by the enzymes from the interchromatin compartment if necessary. PR is enriched with regulatory and coding sequences of active genes and represents the most important (but not exclusive) nuclear subdivision for transcription, RNA splicing, DNA replication and repair. Stan Fakan et al. showed around the year 2000 that transcription and DNA replication are preferably carried out within PR.
In the CT-IC model, active genes should be located more on the surface of the folded chromatin fibers, inactive genes more in the interior of the chromatin structure. In order to make internal genes also accessible for transcription, the chromatin structure would be loosened up if necessary. Continuous, restricted chromatin motions were observed from the level of single ∼1 Mbp chromatin domains to entire CTs. Such movements force continuous changes in the actual width of IC channels and lacunae (cavities, "lacunas"). This offers opportunities for dynamic changes in chromatin interactions. According to the CT-IC model, chromosome territories and also chromatin domains (e.g. p and q arms) show little or no intermingling between them, as some studies show. Although the CT-IC model is a robust and well-accepted hypothesis, it remains to be confirmed whether there are higher order structures such as the 100 kbp domain and what functional elements drive such an organization.
CT-IC ANC-INC
The CT-IC ANC-INC model extends the CT-IC model. It integrates current knowledge about the structural organization of the cell nucleus into a framework of two structurally and functionally intertwined compartments, the active nuclear compartment (ANC) and the inactive nuclear compartment (INC).
According to this model, a chromosome territory is built up from “ topologically associated chromatin domains ” ( TAD ). These form a network of chromatin domain clusters ( CDCs ).
The active core compartment ANC contains DNA with a low density. It contains active genes that are currently being read. It is enriched with epigenetic markings for transcriptional chromatin and RNA polymerase II . The ANC is the most important place for RNA synthesis . It contains two main structural units, the interchromatin compartment (IC) and the perichromatin region (PR). The perichromatin region ( PR ) is formed by the transcriptionally active periphery of the chromatin domain clusters (CDCs) of the ANC. These areas are less compact. Regulatory sequences of genes can be exposed within the ANC, regardless of whether genes are active or inactive. Chromin loops can protrude from the CDCs / TADs and serve as the main sites of RNA synthesis.
The inactive core compartment ( INC ) has a high DNA density, which contains the genes that have been shut down in this cell. The INC comprises the compact, transcriptionally inactive core of CDCs. In conventional terminology, the INC can be viewed as part of the facultative heterochromatin.
How the ANC and the INC interact together is still the subject of current research. Thus, the scenario is considered that only regulatory sequences that are actively used in a certain cell are located within the ANC, while those sequences of genes that are permanently switched off in this cell are withdrawn into the INC, which is controlled by the compact and largely inaccessible interiors of CDCs. Or regulatory sequences of genes are always accessible within the ANC, regardless of whether genes are active or inactive.
The IC is connected to the nuclear pores and is used for the nuclear import and export of molecules. IC channels allow e.g. B. a simplified movement of mRNPs along the channels to the nuclear pores. They offer preferred routes for functional proteins such as B. transcription factors (TFs) that penetrate the interior of the nucleus and lead to their DNA binding sites. And they enable rapid intranuclear distribution of factors stored in nuclear bodies, as well as regulatory, non-coding RNAs, from sites where such RNAs are synthesized to sites where such factors and RNAs are needed.
ICN
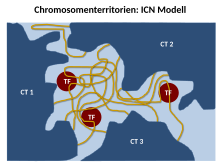
The Branco and Pombo ICN (Interchromatin Network) model predicts that intermingled chromatin fibers / loops can make both cis (within the same chromosome) and trans (between different chromosomes) contacts. This intermingling / intermingling is uniform. Therefore, the distinction between the chromosome territory and the interchromatin compartment is functionally meaningless. Using FISH experiments on ultra-thin frozen sections, they were able to show that up to 20% -30% of the volume of a chromosome territory can be shared with the neighboring territory. Simulations of chromosome translocations based on models of chromosome organization have indicated the existence of a significant amount of mixing of DNA between CTs.
The ICN model suggests that the balance between tissue-specific intra- and interchromosomal associations will define the position and conformation of the chromosome. This occurs along with a functional bond with other nuclear landmarks such as the lamina and nucleoli. In this interchromatin network, loops from one CT can expand to meet loops from another CT. Loop formation was first demonstrated in mammalian cells, where a control region physically interacts with active genes 50 kb away.
The interweaving could be confirmed on the ultrastructural level after the chromosomes had been marked with the immunogold dye and imaged with an electron microscope. There is therefore a high potential for interchromosomal interactions, which is reflected in the frequency of chromosome rearrangements. Indeed, the degree of mixing between pairs of CTs correlates with their translocation potential.
The advantage of the ICN model is that it enables high chromatin dynamics and diffusion-like movements. The authors suggest that sequential transcription affects the degree of intermingling between specific chromosomes by stabilizing the associations between specific locations. Such interactions are likely to depend on the transcriptional activity of the loci and are therefore cell type specific.
Lattice model
The Dehgani et al. The proposed lattice model (grid model) is based on the knowledge that transcription also takes place within the inner, more condensed chromosome territories and not only at the interface between the interchromatin compartment and the perichromatin region. Using ESI (electron spectroscopic imaging), Dehgani showed that chromatin was organized as an array of deoxy-ribonucleoprotein fibers 10-30 nm in diameter. In Deghani's study, the interchromatin compartments, which are described as large channels between chromosome territories in the CT-IC model, were not visible. Instead, chromatin fibers created a loose mesh of chromatin throughout the nucleus that mingled on the periphery of chromosome territories. The inter- and intra-chromosomal spaces within this network are essentially connected and together form the intra-nuclear space.
Other models
The Fraser and Bickmore model emphasizes the functional importance of giant chromatin loops originating from chromosome territories and stretching across nuclear space to share transcription factories. In this case, both cis and trans loops of decondensed chromatin can be co-expressed and co-regulated by the same transcription factory.
The chromatin polymer models assume a wide range of chromatin loop sizes. They predict the observed distances between genomic loci and chromosome territories, as well as the likelihood that contacts will be formed between certain loci. These models apply physical approaches that clarify the importance of entropy for understanding the core organization.
References
- ↑ a b c d e Robert Mayer: Arrangement and structure of chromosome territories in mouse cells: cell type-specific differences and similarities. (PDF) February 1, 2006, accessed on July 7, 2019 (dissertation at the Faculty of Biology Ludwig-Maximilians-Universität München).
- ↑ a b c d e f g h i j k l m n What are chromosomes and chromosome territories? Accessed July 7, 2019 .
- ↑ C. Zorn, T. Cremer, C. Cremer, J. Zimmer: Laser UV microirradiation of interphase nuclei and post-treatment with caffeine. A new approach to establish the arrangement of interphase chromosomes (1976) Hum Genet. 1976 Dec 29; 35 (1): 83-9. PMID 1002167
- ↑ Schardin et al. , Hum. Genet. 71: 281, 1985. Specific staining of human chromosomes in Chinese hamster x man hybrid cell lines demonstrates interphase chromosome territories. PMID 2416668
- ↑ Manuelidis , Hum. Genet. 71: 288, 1985. Individual interphase chromosome domains revealed by in situ hybridization. PMID 3908288
- ↑ Martin A. Lysak, Ales Pecinka, Ingo Schubert. Recent progress in chromosome painting of Arabidopsis and related species (2003) Chromosome Res. 11 (3): 195-204 doi: 10.1023 / A: 1022879608152 .
- ↑ a b c d e f g h i j k l m Kathrin Teller: The architecture of the X chromosome - a "top down" analysis in human fibroblasts. (PDF) February 28, 2008, accessed on July 7, 2019 (dissertation from the Faculty of Biology at the Ludwig Maximilians University of Munich).
- ↑ a b c d e f g T. Cremer, M. Cremer: Chromosome Territories . In: Cold Spring Harbor Perspectives in Biology . tape 2 , no. 3 , March 1, 2010, ISSN 1943-0264 , p. a003889 – a003889 , doi : 10.1101 / cshperspect.a003889 ( cshlp.org [accessed July 7, 2019]).
- ↑ TC Hsu, Kurt Benirschke: Erignathus barbatus (Bearded seal) . In: An Atlas of Mammalian Chromosomes . Springer New York, New York, NY 1975, ISBN 978-1-4684-7996-6 , pp. 137–140 , doi : 10.1007 / 978-1-4615-6434-8_35 ( springer.com [accessed July 7, 2019]).
- ^ SB Zimmerman, AP Minton: Macromolecular Crowding: Biochemical, Biophysical, and Physiological Consequences . In: Annual Review of Biophysics and Biomolecular Structure . tape 22 , no. 1 , June 1993, ISSN 1056-8700 , pp. 27-65 f.07- , doi : 10.1146 / annurev.bb.22.060193.000331 .
- ^ Robert A. Meyers: Epigenetic Regulation and Epigenomics . Ed .: Wiley-Blackwell. ISBN 978-3-527-32682-2 , pp. 453-473 .
- ↑ a b c d e f Miguel R. Branco, Ana Pombo: Chromosome organization: new facts, new models . In: Trends in Cell Biology . tape 17 , no. 3 , March 2007, p. 127-134 , doi : 10.1016 / j.tcb.2006.12.006 ( elsevier.com [accessed July 7, 2019]).
- ↑ T. Cremer, A. Kurz, R. Zirbel, S. Dietzel, B. Rinke: Role of Chromosome Territories in the Functional Compartmentalization of the Cell Nucleus . In: Cold Spring Harbor Symposia on Quantitative Biology . tape 58 , no. 0 , January 1, 1993, ISSN 0091-7451 , p. 777–792 , doi : 10.1101 / SQB.1993.058.01.085 ( cshlp.org [accessed June 26, 2019]).
- ↑ T. Cremer, C. Cremer: Chromosome territories, nuclear architecture and gene regulation in mammalian cells . In: Nature Reviews Genetics . tape 2 , no. 4 , April 2001, ISSN 1471-0056 , p. 292-301 , doi : 10.1038 / 35066075 .
- ↑ a b c d e f g T. Cremer, M. Cremer, C. Cremer: The 4D Nucleome: Genome Compartmentalization in an Evolutionary Context . In: Biochemistry (Moscow) . tape 83 , no. 4 , April 2018, ISSN 0006-2979 , p. 313-325 , doi : 10.1134 / S000629791804003X .
- ^ Françoise Jaunin, Astrid E. Visser, Dusan Cmarko, Jacob A. Aten, Stanislav Fakan: Fine Structural in Situ Analysis of Nascent DNA Movement Following DNA Replication . In: Experimental Cell Research . tape 260 , no. 2 , November 2000, pp. 313–323 , doi : 10.1006 / excr.2000.4999 ( elsevier.com [accessed July 7, 2019]).
- ↑ a b c d e Thomas Cremer, Marion Cremer, Barbara Hübner, Hilmar Strickfaden, Daniel Smeets: The 4D nucleome: Evidence for a dynamic nuclear landscape based on co-aligned active and inactive nuclear compartments . In: FEBS Letters . tape 589 , 20PartA, October 7, 2015, p. 2931–2943 , doi : 10.1016 / j.febslet.2015.05.037 ( wiley.com [accessed July 7, 2019]).
- ↑ a b c Christoph Cremer: Mobility and dynamics in the cell nucleus . In: Heidelberger Jahrbücher Online . September 10, 2018, p. Vol. 3 (2018): Perspektiven der Mobility– , doi : 10.17885 / heiup.hdjbo.2018.0.23823 ( uni-heidelberg.de [accessed on July 7, 2019]).
- ↑ a b Miguel R Branco, Ana Pombo: Intermingling of Chromosome Territories in Interphase Suggests Role in Translocations and Transcription-Dependent Associations . In: PLoS Biology . tape 4 , no. 5 , April 25, 2006, ISSN 1545-7885 , p. e138 , doi : 10.1371 / journal.pbio.0040138 ( plos.org [accessed June 26, 2019]).