Sand casting
![]() |
Sand casting is a type of casting in which a metal part (itself called a casting) is cast in an expendable mold made from sand packed around a replica of the object to be cast (called a pattern). After the sand is packed around it, the pattern is removed, and the molten metal is poured. After the metal has solidified and cooled, the sand is swept away, freeing the casting. Sand castings not further worked by polishing or peening are readily recognized by the sand-like texture imparted by the mold. As the accuracy of the casting is limited by imperfections in the mold-making process, there may be extra material to be removed by grinding or machining, more than is required by other, more accurate casting processes. Furthermore, because the mold is destroyed in order to retrieve the object, a new mold must be made for each casting. The molding sand is reusable after adjusting the content of some additives lost in the thermal casting process. The pattern may be used indefinitely to produce molds. Sand molding and casting offers an important possibility of automation of the casting process and advantage allowing production of castings of iron, steel, or bronze, whose melting points are too high for processes such as die casting. Often aluminum will be sand cast where production quantities are small or large objects are to be cast because of favorable low initial costs when compared to die casting.
Manual sand casting process
Patterns
From the design, provided by an engineer or designer, a skilled pattern maker builds a pattern of the object to be produced, using wood, metal, or plastic; other materials such as expanded polystyrene. Sand can even be ground, swept or even strickled into shape. The metal to be cast will contract during solidification, and this may be non-uniform due to uneven cooling. Therefore, the pattern must be slightly larger than the finished product, a difference known as contraction allowance. Pattern-makers are able to produce suitable patterns using 'Contraction rules' (these are sometimes called "shrink allowance rulers" where the ruled markings are deliberately made to a larger spacing according to the percentage of extra length needed). Different scaled rules are used for different metals because different metals / alloys contract by differing amounts. Patterns also have core prints; these create registers within the molds, into which are placed sand 'cores. Such cores, sometimes reenforced by wires, are used to create under cut profiles and cavities which cannot be molded with the cope and drag, such as the interior passages of valves or cooling passages in motor blocks.
Paths for the entrance of metal, during the pouring (casting) process into the mold cavity constitute the runner system and include the sprue, various feeders which maintain a good metal 'feed' and 'runners', and in-gates which attach the runner system to the casting cavity. Gas and steam generated during casting exit through the permeable sand or via the riser, are added either in the pattern itself, or as separate pieces.

Molding box and materials
A multi-part molding box (known as a casting flask, the top and bottom halves of which are known respectively as the cope and drag) is prepared to receive the pattern. Molding boxes are made in segments that may be latched to each other and to end closures. For a simple object—flat on one side—the lower portion of the box, closed at the bottom, will be filled with prepared casting sand or green sand—a slightly moist mixture of sand and clay. The sand is packed in through a vibratory process called ramming and, in this case, periodically screeded level. The surface of the sand may then be stabilized with a sizing compound. The pattern is placed on the sand and another molding box segment is added. Additional sand is rammed over and around the pattern. Finally a cover is placed on the box and it is turned and unlatched, so that the halves of the mold may be parted and the pattern with its sprue and vent patterns removed. Additional sizing may be added and any defects introduced by the removal of the pattern are corrected. The box is closed again. This forms a "green" mold which must be dried to receive the hot metal. If the mold is not sufficiently dried a steam explosion can occur that can throw molten metal about. In some cases, the sand may be oiled instead of moistened, which makes possible casting without waiting for the sand to dry. Sand may also be bonded by chemical binders, such as furane resins or amine-hardened resins.
Chills
To control the solidification and metallurgical structure of the metal, it is possible to place metal plates—chills— in the mold. The associated rapid local cooling will form a finer-grained structure and may form a somewhat harder metal at these locations. In ferrous castings the effect is similar to quenching metals in forge work. The inner diameter of an engine cylinder is made hard by a chilling core. In other metals chills may be used to promote directional solidification of the casting. In controlling the way a casting freezes it is possible to prevent internal voids or porosity inside castings.
Cores
To produce cavities within the casting—such as for liquid cooling in engine blocks and cylinder heads—negative forms are used to produce cores. Usually sand-molded, cores are inserted into the casting box after removal of the pattern. Whenever possible, designs are made that avoid the use of cores, due to the additional set-up time and thus greater cost.

With a completed mold at the appropriate moisture content, the box containing the sand mold is then positioned for filling with molten metal—typically iron, steel, bronze, brass, aluminum, magnesium alloys, or various pot metal alloys, which often include lead, tin, and zinc. After filling with liquid metal the box is set aside until the metal is sufficiently cool to be strong. The sand is then removed revealing a rough casting that, in the case of iron or steel, may still be glowing red. When casting with metals like iron or lead, which are significantly heavier than the casting sand, the casting flask is often covered with a heavy plate to prevent a problem known as floating the mold. Floating the mold occurs when the pressure of the metal pushes the sand above the mold cavity out of shape, causing the casting to fail.
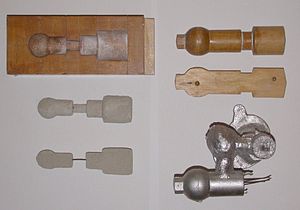
After casting, the cores are broken up by rods or shot and removed from the casting. The metal from the sprue and risers is cut from the rough casting. Various heat treatments may be applied to relieve stresses from the initial cooling and to add hardness—in the case of steel or iron, by quenching in water or oil. The casting may be further strengthened by surface compression treatment—like shot peening—that adds resistance to tensile cracking and smooths the rough surface.
Design requirements
The part to be made and its pattern must be designed to accommodate each stage of the process, as it must be possible to remove the pattern without disturbing the molding sand and to have proper locations to receive and position the cores. A slight taper, known as draft, must be used on surfaces perpendicular to the parting line, in order to be able to remove the pattern from the mold. This requirement also applies to cores, as they must be removed from the core box in which they are formed. The sprue and risers must be arranged to allow a proper flow of metal and gasses within the mold in order to avoid an incomplete casting. Should a piece of core or mold become dislodged it may be embedded in the final casting, forming a sand pit, which may render the casting unusable. Gas pockets can cause internal voids. These may be immediately visible or may only be revealed after extensive machining has been performed. For critical applications, or where the cost of wasted effort is a factor, non-destructive testing methods may be applied before further work is performed.
Essential improvements of the foundry technology
An increasing demand for castings in the growing car and machine building industry during and after the World War One and Two, stimulated new inventions in mechanization and later automation of the sand casting process technology. The bottleneck for a faster casting production was not only the molding speed, but also slow molding sand preparation in the slow sand mullers, slow core manufacturing processes and slow metal melting rate in the cupola furnaces. In 1912 sand slinger was invented by the American Company Birdsley & Piper. In 1912 the first sand muller with individually mounted revolving plows was marketed by Simpson Company. In 1915 first experiments started with bentonite clay in stead of simple fireclay as bonding additive to the molding sand. This increased tremendously the green and dry strength of the moulds. In 1918 the first fully automated foundry fabricating hand grenades for the U.S. Army. In 1930 first high-frequency coreless electric furnace was installed in the U.S. In 1924 Henry Ford sets record producing 1 million cars, consuming one-third of the total casting production in the U.S. In 1943 ductile iron was invented by adding magnesium to the widely used grey iron. In 1940 thermal sand reclamation was applied for molding and core sands. In 1952 D-process was developed for making shell molds with fine, pre-coated sand. In 1953 hotbox core sand process was invented by thermally curing cores. In 1954 a new core binder - water glass hardened with CO2 from the ambient air, was applied.
Fast molding & sand casting processes
With the fast development of the car and machine building industry the casting consuming areas called for steady higher productivity. The basic process stages of the mechanical molding and casting process are similar to those described under the manual sand casting process. The technical and mental development however was so rapid and profound that the character of the sand casting process changed radically.
Mechanized sand molding
The first mechanized molding lines consisted of sand slingers and/or jolt-squeeze devices that compacted the sand in the flasks. Subsequent mould handling was mechanical using cranes, hoists and straps. After core setting the copes and drags were coupled using guide pins and clamped for closer accuracy. The moulds were manually pushed off on a roller conveyor for casting and cooling.
Automatic high pressure sand molding lines
Increasing quality requirements made it necessary to increase the mould stability by applying steadily higher squeeze pressure and modern compaction methods for the sand in the flasks. In early fifties the high pressure molding was developed and applied in mechanical and later automatic flask lines. The first lines were using jolting and vibrations to precompact the sand in the flasks and compressed air powered pistons to compact the molds.
Horizontal sand flask molding
In the first automatic horizontal flask lines the sand was shot or slung down on the pattern in a flask and squeezed with hydraulic pressure of up to 140 bars. The subsequent mould handling including turn-over, assembling, pushing-out on a conveyor were accomplished either manually or automatically. In the late fifties hydraulically powered pistons or multipiston systems were used for the sand compaction in the flasks. This method produced much more stable and accurate molds than it was possible manually or pneumatically. In the late sixties mold compaction by fast air pressure or gas pressure drop over the pre-compacted sand mold was developed (sand-impulse and gas-impact). The general working principle for most of the horizontal flask line systems is shown on the sketch below.

Today there are many manufacturers of the automatoc horizontal flask molding lines. The major disadvantages of these systems is high spare parts consumption due to multitude of movable parts, need of storing, transporting and maintaining the flasks and productivity limited to approximately 90 –120 molds/hour per molding unit.
Vertical sand flaskless molding
In the end of the fifties foundry industry, as all the others, called constantly for reduction of the labor costs, higher productivity casting quality and their dimensional accuracy. Due to constantly increasing wages reduction of the human labor became important. This required automation. In 1962 Danish company Dansk Industri Syndikat A/S (DISA) implemented an ingenious idea of molding without flasks applying vertically parted and poured moulds. The first automatic DISA molding line could produce up to 240 complete sand molds per hour. Today a modern DISA molding line can achieve a molding rate of 550 sand molds per hour (one complete mold for each 6.5 seconds) and requires only one monitoring operator. Maximal mismatch of two half’s of the castings made on the DISA lines does not exceed 0,1 mm. Apart from the high productivity, low labor requirement and dimensional castings accuracy DISA vertical flaskless moulding lines are very reliable (up to 98% in efficiency].

Virtually there are no other serious manufacturers of the vertical flaskless molding lines but the Danish DISA Industries.
Matchplate sand molding
The principle of the matchplate, meaning pattern plates with two patterns on each side of the same plate, was developed and patented in 1910, fostering the perspectives for future sand molding improvements. However first in the early sixties the American company Hunter Automated Machinery Corporation launched its first automatic flaskless, horizontal molding line applying the matchplate technology.

The method alike to the DISA's vertical moulding is flaskless, however horizontal. It has been improved by several producers.The main suppliers are the DISA Industries, Hunter Automated Machinery and Heinrich Wagner Sinto. The matchplate molding technology is today used widely, particularly in the U.S., China and India. Its great advantage is inexpensive pattern tooling, easiness of changing the molding tooling, thus suitability for manufacturing castings in short series so typical for the jobbing foundries. Modern matchplate molding machine is capable of high molding quality, less casting shift due to machine-mold mismatch (in some cases even 0.15 mm or less), consistently stable molds for less grinding and improved parting line definition. In addition, the machines are enclosed for a cleaner, quieter working environment with reduced operator exposure to safety risks or service-related problems.
Decorative use of wood patterns
Some collectors seek obsolete hardwood patterns, once used to make molds for casting machine parts, to use as interior decorations. These are valued due to the fine woodworking involved, sometimes interesting sculptural shapes of decorative embelishments, and the display of the grain of the wood.
Alternative casting methods
As a supplement to the sand casting other casting methods were succesfully applied.
- Modern casting production methods can manufacture thin and accurate molds—of a material superficially resembling paper mache, such as is used in egg cartons, but that is refractory in nature—that are then supported by some means, such as dry sand surrounded by a box, during the casting process. Due to the higher accuracy it is possible to make thinner and hence lighter castings, because extra metal need not be present to allow for variations in the molds. These thin-mold casting methods have been used since the 1960s in the manufacture of cast-iron engine blocks and cylinder heads for automotive applications.
- Steadily growing types of various automotive components are presently frequently made of aluminum, which for appropriately shaped components may be made either by sand casting or by die casting, the latter an accurate process that greatly reduces both materials use and machining and finishing costs. While the material and the processing setup is more expensive than the use of iron this is one of the most straightforward ways to reduce weight in a vehicle, important as a contributor to both fuel economy and acceleration performance. For front engine vehicles with rear wheel drive the improvement in weight distribution can improve both handling and traction. For all configurations weight saved in the engine is multiplied in that this enables use of lighter suspension components which in turn improves suspension response by reducing unsprung weight
- Starting in the early 1980s, some castings such as automotive engine blocks have been made using a sand casting technique conceptually similar to the lost wax process, known as the lost foam process. In this process, the pattern is made of polystyrene foam, which the sand is packed around, leaving the foam in place. When the metal is poured into the mold, the heat of the metal vaporizes the foam a short distance away from the surface of the metal, leaving the molding cavity into which the metal flows. The lost-foam process supports the sand much better than conventional sand casting, allowing greater flexibility in the design of the cast parts, with less need for machining to finish the casting. This technique was developed for the clay mold casting of abstract art pieces and was first adopted for large quantity commercial production by the Saturn Corporation.
External links
- Iron casting in a hobby foundry
- Aluminum and bronze casting in a homemade foundry
- Horizontal Flask Sand Molding
- Vertical Flaskless Sand Molding
- Matchplate Horizontal Flaskless Sand Molding
Template:Metalworking double box