Atmospheric electricity

Atmospheric electricity (also air electricity ) is the observation of electrical phenomena, especially electrical discharges , in the earth's atmosphere or the atmosphere of another planet. The study of atmospheric electricity is an interdisciplinary subject with a long history that includes concepts from electrostatics and electrodynamics , atmospheric physics , meteorology, and earth sciences .
Atmospheric electricity can be felt during thunderstorms in which lightning transports charges, but even in fine weather there is an electrostatic field of the earth with a field strength of about 100–130 V / m .
The cause is the continuous electrification of the air through ionization due to cosmic radiation and natural radioactivity . The resulting movement of charges between the earth's surface, the atmosphere and the ionosphere is known as the global atmospheric electrical circuit.
history
The first scientists like Francis Hauksbee , Isaak Newton , William Wall, Jean-Antoine Nollet and Stephen Gray discovered in experiments with the Leyden bottle that lightning is caused by electrical discharges. In 1708, William Wall discovered that a charged piece of amber gave off sparks that looked like lightning. Benjamin Franklin showed in his experiments that electrical phenomena in the atmosphere are not fundamentally different from the lightning bolts generated in the laboratory. In 1749 Franklin observed that lightning had all the properties that can be found in electrical machines.
In July 1750, Franklin hypothesized that electricity could be drawn from clouds with the help of metal antennas with a sharp tip. Before Franklin could carry out the experiment, Thomas-François Dalibard had built an iron antenna in Marly-la-Ville near Paris in 1752 , which he used to attract lightning from thunderclouds. With an antenna connected to a grounded cable and fitted with an insulated wax handle, he was able to observe a lightning discharge from the antenna to the cable. So he confirmed that Franklin's theory was correct.
Around 1752 Franklin reported a famous kite experiment. The experiment was repeated by Romas, who observed sparks on a metal rod, and by Tiberius Cavallo , who made important observations on atmospheric electricity. Louis Guillaume Le Monnier reproduced Franklin's experiment with an antenna in 1752, but replaced the grounded cable with dust particles. He also documented the electrical charge of the atmosphere in good weather. Giovanni Battista Beccaria confirmed Lemmonier's data in 1775 and confirmed that the atmosphere is positively charged in good weather. In 1779, Horace-Bénédict de Saussure recorded data demonstrating the induced charge in the atmosphere. Saussure's instrument, two balls on thin cables, is the forerunner of the electrometer . Saussure found that the electrification of the atmosphere in good weather conditions varied annually and is different depending on the altitude.
In 1785 Charles-Augustin de Coulomb discovered the electrical conductivity of air. His discovery was contrary to the prevailing opinion at the time that atmospheric gases were insulators (which, to a certain extent, they are if they are only slightly ionized). Paul Erman theorized in 1804 that the earth is negatively charged and Jean Charles Athanase Peltier confirmed that.
Numerous scientists contributed to the growing body of knowledge about atmospheric electrical phenomena. Francis Ronalds began observing a potential gradient and air currents around 1810, recording continuous electrometer data. In 1840, as director of King's Observatory / Kew Observatory, he summarized his scientific work, with which he created the first data set of meteorological records. He also lent his equipment worldwide with the aim of collecting global data on atmospheric electricity. Kelvin's water drop collector and ring electrometer were presented at the Kew Observatory in 1860, and atmospheric electricity remained the specialty of the Observatory until it closed. At that time, kites, weather balloons or aerostats were used to measure altitude in order to lift the equipment for the experiments into the air. Early experiments were even carried out in hot air balloons .
Hoffert identified various thunderstorms with one of the early cameras in 1888. Elster and Geitel , who also worked on thermionic emissions, proposed a theory in 1885 that explained the electronic structure of thunderstorms and later (1899) they discovered atmospheric radioactivity, as well as the existence of positive and negative ions in the atmosphere. Pockels (1897) estimated the current intensity of weather lighting by measuring the flashes of light with basalt (c. 1900) and he studied the magnetic field that builds up during weather lighting. Discoveries about the electrification of the atmosphere were made with sensitive measuring instruments and the theory that the earth is negatively charged was developed in the 20th century, with Charles Thomson Rees Wilson playing an important role. Inspired by Franz-Serafin Exner's work in the field of air electricity, the later Nobel Prize winner Victor Franz Hess dealt with this topic, which led to the detection of cosmic rays.
Current research on atmospheric electricity focuses primarily on weather lights, especially on energetic particles and light phenomena, which - even in the absence of thunderstorms - affect weather and climate in electrical processes in the atmosphere.
description
Atmospheric electricity is always present; Even in good weather without a storm front, the air above the earth's surface is positively charged, while the ground on the earth's surface is negatively charged. This can be understood as the potential difference between a point on the earth's surface and a point in the air layer above. The electric field strength on the surface is 100 V / m. The potential gradient in most areas is lower than this value because the average charge decreases with every thunderstorm and every atmospheric turbulence. A weak stream of atmospheric ions moves through the atmospheric electric field, about 2 picoamps per square meter, and the air is poorly conductive because of the presence of atmospheric ions.
Variations
Global daily cycles of the atmospheric magnetic field were studied by the Carnegie Institute in Washington in the 20th century. The Carnegie curve has been referred to as the electrical "heartbeat of the planet". Atmospheric electricity can vary widely even without thunderstorms, but in general the electric field is strengthened by fog and haze, while the electrical conductivity is reduced.
Near-Earth space
The electrosphere is a layer (from 10 km from the earth's surface to the ionosphere) that has a high electrical conductivity at a constant electrical potential. The ionosphere is the inner edge of the magnetosphere and part of the atmosphere that is ionized by the rays of the sun. Photoionization is a physical process in which a photon hits an atom, ion or molecule and knocks one or more electrons out of it.
Cosmic rays
The earth and almost all living things on it are exposed to constant radiation from space. This radiation consists primarily of protons from extraterrestrial sources. The radiation interacts with atoms in the atmosphere and creates an air stream of ionizing radiation such as X-rays , electrons , protons , alpha particles , pions and muons . The ionization that emanates from this secondary radiation means that the atmosphere is slightly conductive and that there is a slight current flow over the earth's surface, which compensates for the current flow from thunderstorms. Ions have characteristic parameters such as electron mobility, life span and generation rate, which vary with the height of the atmospheric air layer.
Thunderstorms and lightning
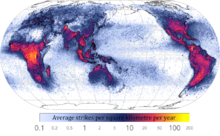
The potential difference between the ionosphere and the earth is maintained through thunderstorms, as the lightning strikes transfer negative charge from the atmosphere to the ground. Collisions between ice and sleet inside the thunderclouds ( cumulonimbus ) cause a charge separation between positive and negative charges inside the clouds, which creates the lightning. How the lightning occurs is still in the focus of the discussion: Scientists propose various mechanisms as the cause, from atmospheric air turbulence (wind, moisture and atmospheric pressure) to collisions with solar wind and energy particles. An average lightning strike carries a negative electric current of 40 kiloamps (some lightning strikes can also have 120 kA), transfers a charge of 5 coulombs and an energy of 500 megajoules, enough to operate a 100 watt light bulb for almost two months. The voltage depends on the length of the lightning bolt, the airflow of which has a dielectric breakdown of 3 million volts per meter, and which is approximately several hundred meters long. However, the development of lightning is not only dependent on the dielectric breakdown of the air and the electric field required for the lightning strike can be several orders of magnitude smaller than the dielectric breakdown. In addition, the potential gradient within the kickback channel is a few orders of magnitude volts per meter (or less) due to the ionization, which leads to a real current force of the order of a few megawatts per meter with a strong kickback current of 100 kA. If the amount of water that has condensed in a cloud and then rained down is known, the total energy of a thunderstorm can be calculated. In an average thunderstorm, energy of 10,000,000 kilowatt hours (3.6 * 10 13 joules) is released, a strong thunderstorm is 10 to 100 times more energetic.
Corona discharge

The Elmsfeuer is an electrostatic phenomenon with a plasma generated by a corona discharge that emanates is generated by a grounded object. Weather lights are also often incorrectly referred to as Elmsfeuer, although both are different phenomena. Although it is referred to as “fire”, the Elmsfeuer is actually a plasma of light observed as a star of light at the top of trees or tall objects during a thunderstorm. A corona discharge is caused by an electric field in which the air molecules that surround the object in question are ionized and thus become visible. It takes about 1,000-30,000 volts per centimeter to create an Elms Fire, but this also depends on the shape of the object in question. Sharp tips tend to require less voltage, since electric fields lead to more intense discharges on surfaces with a steep slope. Elm fires and sparks can occur when high electrical voltage meets gases. Elm's fire can be seen during a thunderstorm when the air layer under the thunderstorm is electrically charged and a high voltage has built up in the air between the cloud and the ground. The tension pulls the air molecules apart and the gas begins to glow. The nitrogen and oxygen in the earth's atmosphere make the Elms fire fluoresce blue or purple.
Earth ionospheric cavity
The Schumann resonance is a series of peaks in the low frequency range of the earth's electromagnetic spectrum. It is caused by the cavity between the earth and the conductive ionosphere, where these waves are generated. This cavity is naturally energized by the energy of lightning strikes.
Electrical grounding system
Atmospheric charges can build up undesirable, dangerous, and sometimes fatal voltages in electrical power distributors. Cables hanging in the air for several kilometers and isolated from the ground can store large amounts of charge at high voltage, even without thunderstorms. This charge self-discharges via the path of the slightest isolation, which happens when a person turns on a power switch or uses an electrical device. In order to avoid the build-up of atmospheric charge, an electrical distribution system is earthed at many points, at least at each connector. A grounded cable is regarded as "safe" and ensures the potential reduction / charge discharge without causing damage. The additional grounding cable, in which normally no current flows, plays a second role, namely to ensure immediate current drainage in the event of a short circuit.
literature
Magazines
- FJ Anderson, GD Freier: Interactions of the thunderstorm with a conducting atmosphere . In: J. Geophys. Res. Band 74 , 1969, pp. 5390-5396 , doi : 10.1029 / jc074i023p05390 , bibcode : 1969JGR .... 74.5390A .
- M. Brook: Thunderstorm electrification. In: SC Coroniti (Ed.): Problems of Atmospheric and Space Electricity. Elsevier, Amsterdam 1965, pp. 280-283.
- WM Farrell, TL Aggson, EB Rodgers, WB Hanson: Observations of ionospheric electric fields above atmospheric weather systems . In: J. Geophys. Res. Band 99 , 1994, pp. 19475–19484 , doi : 10.1029 / 94ja01135 , bibcode : 1994JGR .... 9919475F .
- RF Fernsler, HL Rowland: Models of lightning-produced sprites and elves . In: J. Geophys. Res. Band 101 , 1996, pp. 29653-29662 , doi : 10.1029 / 96jd02159 , bibcode : 1996JGR ... 10129653F .
- AC Fraser-Smith: ULF magnetic fields generated by electrical storms and their significance to geomagnetic pulsation generation . In: Geophys. Res. Lett. tape 20 , 1993, p. 467-470 , doi : 10.1029 / 93gl00087 , bibcode : 1993GeoRL..20..467F .
- EP Krider, RJ Blakeslee: The electric currents produced by thunderclouds . In: J. Electrostatics . tape 16 , 1985, pp. 369-378 , doi : 10.1016 / 0304-3886 (85) 90059-2 .
- BV Lazebnyy, AP Nikolayenko, VA Rafal'skiy, AV Shvets: Detection of transverse resonances of the Earth-ionosphere cavity in the average spectrum of VLF atmospherics . In: Geomagn. Aeron . tape 28 , 1988, pp. 281-282 .
- T. Ogawa: Fair-weather electricity . In: J. Geophys. Res. Band 90 , 1985, pp. 5951–5960 , doi : 10.1029 / jd090id04p05951 , bibcode : 1985JGR .... 90.5951O .
- DD Sentman: Schumann resonance spectra in a two-scale-height Earth-ionosphere cavity . In: J. Geophys. Res. Band 101 , 1996, pp. 9479-9487 , doi : 10.1029 / 95jd03301 , bibcode : 1996JGR ... 101.9479S .
- L. Wåhlin: Elements of fair weather electricity . In: J. Geophys. Res. Band 99 , 1994, pp. 10767-10772 , doi : 10.1029 / 93jd03516 , bibcode : 1994JGR .... 9910767W .
Further references
- Richard E. Orville (Ed.): Atmospheric and Space Electricity "(" Editor's Choice "virtual journal ) -" American Geophysical Union ". ( AGU ) Washington, DC 20009-1277 USA
- BFJ Schonland: Atmospheric Electricity. Methuen and Co., London 1932.
- Donald R. MacGorman, W. David Rust, DR Macgorman, WD Rust: The Electrical Nature of Storms. Oxford University Press, 1998, ISBN 0-19-507337-1 .
- Thomas Gilbert Cowling: On Alfven's theory of magnetic storms and of the aurora. In: Terrestrial Magnetism and Atmospheric Electricity. Volume 47, 1942, pp. 209-214.
- HH Hoffert: Intermittent Lightning Flashes. In: Proc. Phys. Soc. London. Volume 10, No. 1, June 1888, pp. 176-180.
- H. Volland: Atmospheric Electrodynamics. Springer, Berlin 1984, ISBN 3-540-13510-3 .
Books
- James R. Wait : Some basic electromagnetic aspects of ULF field variations in the atmosphere. In: Journal Pure and Applied Geophysics. Volume 114, Number 1, January 1976 p. 15-28. doi: 10.1007 / BF00875488
- National Research Council (US), American Geophysical Union: The Earth's electrical environment. National Academy Press, Washington, DC 1986. (books.google.com)
- DN Baker: Solar Dynamics and Its Effects on the Heliosphere and Earth. International Space Science Institute. (books.google.com)
- Solar variability, weather, and climate. National Research Council (US). Geophysics Study Committee. books.google.com
Web links
- Monte Bateman: Atmospheric Electricity Homepage .
- International Commission on Atmospheric Electricity . Commission of the International Association of Meteorology And Atmospheric Physics.
- Lightning and Atmospheric Electricity . Global Hydrology and Climate Center, NASA .
- Sandy Kieft: The Langmuir Laboratory for Atmospheric Research . New Mexico Institute of Mining & Technology.
- Power from the air . Science and Invention (Formerly Electrical Experimenter ), Feb. 1922, no. 10. Vol IX, Whole No. 106. New York. (nuenergy.org)
- Power from the air . Science and invention (Formerly Electrical Experimenter ), March 1922. (nuenergy.org).
- RF Energy via Ionosphere . RF Energy Concepts Sec. 101 Rev. Nov. 2003
- Peter Winkler: Early observations of and knowledge on air electricity and magnetism at Hohenpeißenberg during the Palatina . German Weather Service, Meteorological Observatory. ( PDF )
- Atmospheric Electricity . Meridian International Research.
- Atmospheric Electricity and Plants .
- Do cosmic rays cause lightning? Ask the Experts - sciam.com January 24, 2008.
- The Earth's Electrical Environment . CPSMA, USA National Academies Press.
- Electric Current through the Atmosphere
- The Global Circuit , phys.uh.edu
- Soaking in atmospheric electricity 'Fair weather' measurements important to understanding thunderstorms. science.nasa.gov
- Atmospheric Electricity HomePage , uah.edu
- Tjt, Fair-weather atmospheric electricity . ava.fmi.fi
- ICAE - International Commission on Atmospheric Electricity Homepage
Individual evidence
- ↑ cf. Flashes in the Sky: Earth's Gamma-Ray Bursts Triggered by Lightning
- ^ J. Alan Chalmers: Atmospheric Electricity . Pergamon Press, 1967.
- ↑ Wolfgang Demtröder: Experimentalphysik 2: Electricity and optics . Springer, 2008, ISBN 978-3-540-68210-3 ( limited preview in Google book search).
- ↑ a b R. G. Harrison: Fair weather atmospheric electricity . In: Journal of Physics: Conference Series . tape 301 , no. 1 , January 1, 2011, ISSN 1742-6596 , p. 012001 , doi : 10.1088 / 1742-6596 / 301/1/012001 (English, iop.org ).
- ↑ a b Soaking in atmospheric electricity. March 17, 2008, accessed October 31, 2018 .
- ^ BF Ronalds: Sir Francis Ronalds: Father of the Electric Telegraph . Imperial College Press, London 2016, ISBN 978-1-78326-917-4 .
- ^ BF Ronalds: Sir Francis Ronalds and the Early Years of the Kew Observatory . In: Weather . tape 71 , June 2016, p. 131–134 , doi : 10.1002 / wea.2739 , bibcode : 2016Wthr ... 71..131R .
- ↑ KL Aplin, RG Harrison: Lord Kelvin's atmospheric electricity measurements . In: History of Geo- and Space Sciences . tape 4 , no. 2 , September 3, 2013, ISSN 2190-5010 , p. 83–95 , doi : 10.5194 / hgss-4-83-2013 , arxiv : 1305.5347 , bibcode : 2013HGSS .... 4 ... 83A (English, hist-geo-space-sci.net ).
- ^ HH Hoffert: Intermittent Lightning Flashes. In: Proceedings of the Physical Society. Vol. 9-10, Institute of Physics and the Physical Society, Physical Society (Great Britain), Physical Society of London, 1888, p. 176. (books.google.com)
- ^ Rudolf GA Fricke, Kristian Schlegel: Julius Elster and Hans Geitel - Dioscuri of physics and pioneer investigators in atmospheric electricity . In: History of Geo- and Space Sciences . tape 8 , no. 1 , January 4, 2017, ISSN 2190-5010 , p. 1–7 , doi : 10.5194 / hgss-8-1-2017 , bibcode : 2017HGSS .... 8 .... 1F (English, hist-geo-space-sci.net ).
- ^ A b Vladimir A. Rakov, Martin A. Uman: Lightning: Physics and Effects . Cambridge University Press, 2003.
- ↑ Basalt, a ferromagnetic mineral, becomes magnetically polarized when exposed to a large external field, such as that generated by a lightning strike. See also: Allen Cox: Anomalous Remanent Magnetization of Basalt. Washington 1961. (pubs.usgs.gov)
- ↑ Encyclopedia of Geomagnetism and Paleomagnetism. P. 359. (books.google.com)
- ^ Giles Harrison: The cloud chamber and CTR Wilson's legacy to atmospheric science . In: Weather . tape 66 , no. 10 , October 1, 2011, ISSN 1477-8696 , p. 276–279 , doi : 10.1002 / wea.830 , bibcode : 2011Wthr ... 66..276H (English, wiley.com ).
- ^ R. Giles Harrison: The Carnegie Curve . In: Surveys in Geophysics . tape 34 , 2012, p. 209–232 , doi : 10.1007 / s10712-012-9210-2 , bibcode : 2013SGeo ... 34..209H .
- ↑ Liz Kalaugher: Atmospheric electricity affects cloud height. 2013. (physicsworld.com)
- ^ A. D. McNaught, A. Wilkinson: photoionization . In: IUPAC Compendium of Chemical Terminology (the “Gold Book”) . 2nd Edition. Blackwell Scientific Publications, Oxford 1997, ISBN 0-9678550-9-8 , doi : 10.1351 / goldbook.P04620 (English, corrected version (XML; 2006–) by M. Nic, J. Jirat, B. Kosata; with updates by A. Jenkins - Version: 2.3.3).
- ^ RG Harrison, E. Barth, F. Esposito, J. Merrison, F. Montmessin, KL Aplin, C. Borlina, JJ Berthelier, G. Déprez: Applications of Electrified Dust and Dust Devil Electrodynamics to Martian Atmospheric Electricity . In: Space Science Reviews . tape 203 , no. 1–4 , April 12, 2016, ISSN 0038-6308 , p. 299–345 , doi : 10.1007 / s11214-016-0241-8 , bibcode : 2016SSRv..203..299H (English).
- ^ JD Barry: Ball Lightning and Bead Lightning: Extreme Forms of Atmospheric Electricity. Plenum Press, New York / London 1980, ISBN 0-306-40272-6 , pp. 8-9. (books.google.com)
- ^ NASA - Schumann Resonance. www.nasa.gov, accessed October 31, 2018 .