Plasma (physics)
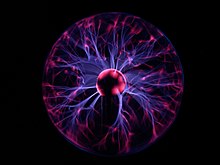
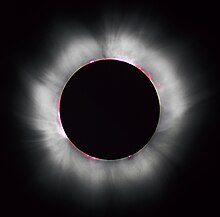

In physics, plasma (from ancient Greek πλάσμα plásma , German 'the formed, formed' ) is a mixture of particles at the atomic-molecular level, the constituents of which are partially charged components, ions and electrons . This means that a plasma contains free charge carriers . The degree of ionization of a plasma can be less than 1%, but also 100% (complete ionization). An essential property of plasmas for the behavior of plasmas, but also for technical use, is their electrical conductivity .
The name plasma goes back to Irving Langmuir (1928). Since the plasma state by further supply of energy from the gaseous state of aggregation may be generated, it is often referred to as the fourth state.
properties
A plasma is generally a mixture of neutral and charged particles (partially ionized plasma). It is characterized by the species present (electrons, positive and negative ions, neutral atoms, neutral and charged molecules), their densities and temperatures (which do not have to be the same) and spatial structure, in particular charge and currents or electric and magnetic fields . In special cases, only charged particles, electrons and ions and / or charged molecules are present (fully ionized plasma).
Depending on the particle densities , temperatures and the relative strength of the active fields (e.g. electrical , magnetic or gravitational fields and combinations thereof), plasmas can behave like gases , but also completely different. In certain cases a plasma can simply be described as an electrically conductive gas with the help of magnetohydrodynamics . In general, however, transport processes (radiation transport, transport of thermal energy, particle transport, impulse transport) must also be taken into account, as well as other processes that determine the plasma composition (i.e. ionization, recombination, dissociation, molecule and / or exciton formation and chemical reactions of the species present, among others , Excitation and absorption processes), so that a complete description can become much more complex.
Among other things, plasmas can be characterized by the following three properties:
- The Debye length is small compared to the dimensions.
- The plasma parameter (number of particles in a sphere with a radius equal to Debye's length) is large.
- The time between bursts is long compared to the period of the plasma oscillations .
Plasmas are usually quasi-neutral; that is, the charges of the ions and electrons are approximately in equilibrium. The net charge density is very small compared to the electron density. Exceptions are limited to regions the size of Debye longitude, e.g. B. in the edge layer .
The ratio between ion mass and electron mass is large, at least 1836 (for a hydrogen plasma). Many properties of plasmas can be derived from this.
A characteristic of plasmas is their typical glow, which is caused by the emission of radiation from excited gas atoms, ions or molecules. Exceptions are plasmas that are very cold (as is often the case in space) or that are so hot that the atoms are completely ionized (as in the center of stars).
Designations
If there is a neutral particle component whose kinetic energy is small compared to the kinetic energy of the free charge carriers, this is often referred to as background gas or also neutral gas .
Plasmas are often given more specific names depending on the properties present or predominant. So one speaks z. B. of high or low pressure plasmas, cold or hot plasmas, non-ideal plasmas or dense plasmas. The components of a plasma can also be used to denote such as B. High pressure mercury plasma. In addition, the generation mechanism also plays a role in the characterization of plasmas: For example, noble gas low-pressure discharge means an electrically generated noble gas plasma with low plasma pressure.
In particle physics , the quasi-free state of quarks and gluons is analogously referred to as quark-gluon plasma .
Occurrence
The thin matter in space between the celestial bodies, such as the solar wind or interstellar matter , is partly in the plasma state; also the sun and other stars . More than 99% of all visible matter in the universe is in the plasma state.
Natural plasmas are found in the ionosphere and in lightning bolts on Earth . Despite only weak ionization (depending on the temperature), flames also have some properties of a plasma.
There are no practically usable natural plasmas in the biosphere . A plasma must therefore be generated in order to be able to use it technically. This is usually done with the help of a gas discharge .
Applications

Various chemical or physical processes occurring in the plasma can be exploited.
The application of plasmas can be broken down as follows:
- Gas discharge lamps : energy-saving lamps , fluorescent lamps and arc lamps, among others , contain matter in the plasma state
- Surface technology: Plasmas are used in semiconductor technology for plasma etching and plasma-induced material deposition ( PECVD ). In coating technology, functional layers such as B. mirror coatings or non-stick layers applied. In materials technology, plasmas are used for surface modification (roughening), for plasma-induced material deposition (PECVD and plasma polymerisation ), for surface hardening, cleaning, activation or plasma oxidation.
- Analytical technology: for breaking down sample materials ( plasma ashing ) and in measuring devices for detecting traces of metals ( inductively coupled plasma (ICP), ICP-MS ; English inductively coupled plasma mass spectrometry , LIBS ; English laser induced breakdown spectroscopy , see atomic spectroscopy )
- Material processing: plasma welding , spark erosion and plasma cutting
- Plasma screen
- Nuclear fusion reactor : plasma state is necessary for every thermonuclear reaction
-
Plasma Medicine :
- Plasma disinfection: disinfection of objects, body parts, wounds, etc.
- Coblation : The energy-rich ions in the plasma can separate human tissue at relatively low temperatures below 70 ° C. This is used for surgical measures on intervertebral discs , tonsils or turbinates.
Lighting technology
The glow typical of plasmas is used. In the plasma, collision processes of fast electrons with gas atoms or molecules lead to the electrons being supplied with energy from the shell of the impacted particles . This energy is then released as emitted light at a later point in time. The resulting spectrum strongly depends on the gases present, the pressure and the mean energy of the electrons.
In some cases the emitted light can be used directly, e.g. As in some metal vapor high-pressure lamps (eg sodium lamps - to acknowledge the strong yellow light) which used in street lighting were used and come or certain noble gas - high-pressure discharges (eg. Xenon ). In other cases, when the emission is more in the UV range (mainly mercury vapor lamps ), the electromagnetic radiation has to be converted into light that is visible to humans. This is achieved with phosphors which are applied to the wall of the discharge vessels. The ultraviolet radiation is absorbed in the luminescent material and emitted again as radiation in the visible. Examples of this are the fluorescent and energy-saving lamps used in interior lighting and the high-pressure mercury lamps used in projectors and outdoors.
Plasma chemical applications
The use of plasmas for chemical reactions is based on the high concentrations of chemically reactive molecular fragments they deliver. In the past there have been attempts to use plasma chemical processes industrially for synthesis. However, the complex plasma composition makes such reactions very complex and inefficient. Plasma-chemical processes are therefore practically no longer used in chemical synthesis today, but only for the disposal of toxic gases.
An example of its successful application is the synthesis of diamonds . A diamond is deposited onto a surface from the plasma. This diamond layer is polycrystalline and does not have the quality of jewelry diamonds. The growth rates of this layer are very small (approx. 1 µm / h). Therefore, thicker layers are very expensive.
Plasma chemistry continues to be carried out on a large scale in the semiconductor industry. Here plasmas are used for (dry) etching ( plasma etching ) and for layer deposition PECVD . In contrast to lighting technology, etching processes utilize direct contact between the plasma and the surface in order to achieve targeted material removal. The electric fields prevailing near the wall, which are characteristic of edge layers , play a key role here . The ions contained in the plasma form another large part of the etching removal. These can be accelerated with the help of magnetic fields and thus achieve additional, directed etching removal. Plasma etching does not have to be associated with chemically reactive processes and is therefore a physical application.
Physical applications
Plasmas are used for plasma cutting , plasma welding and soldering with plasma torches . The plasma is mostly generated by means of an electric arc . New processes use plasmas that are generated at 2.45 GHz, work with wear-free copper electrodes and can also be used for the finest cuts, including scalpels.
Furthermore, plasmas are used for the pretreatment (cleaning and activation) of adhesive connections, which is now the standard process in the German automotive industry. Two types of plasma can be used here: On the one hand, the low-pressure plasma , which cleans and activates the surfaces at room temperature. With this type of plasma, however, it is also possible to coat or etch components in a hydrophobic or hydrophilic manner. The excitation takes place here mostly via generators with frequencies of 40-100 kilohertz , 13.56 megahertz or 2.45 gigahertz (microwave excitation ). On the other hand, plasma jets based on arc or spark discharge can also be used for cleaning and activation .
The magnetosphere plasma dynamics describes the behavior of flowing plasmas in a magnetic field. Electric energy can be obtained (MHD generator) or it is used to propel spacecraft ( magnetoplasmic dynamic propulsion ).
High density hot plasmas - generated by laser pulse irradiation or by electrical discharges - serve as the EUV radiation source. EUV lithography is a potential user .
Classification
A classification of the very different forms of plasma can be made on the basis of several criteria. One of them is plasma density . Naturally occurring plasmas vary in their density by more than ten orders of magnitude. The plasma has an extremely high density in the interior of the sun, extremely low density prevails in interstellar gas nebulae. The differences in the physical properties of plasmas are correspondingly extreme.
Further parameters for differentiating plasmas are plasma pressure and plasma temperature.
Plasma pressure
A distinction can be made between
- Low pressure plasma
- Normal pressure plasmas or atmospheric pressure plasmas
- High pressure plasmas
Low pressure plasmas are generated in dilute gases, the pressure of which is significantly lower than atmospheric pressure. Examples are glow lamps , the aurora borealis or fluorescent lamps .
In the case of high pressure plasmas , the plasma pressure is significantly higher than atmospheric pressure. A typical example are high and extremely high pressure gas discharge lamps . Even in thunderstorms and sparks there is briefly very high pressure.
Normal pressure plasmas are generated at approximately atmospheric pressure . A typical application is the dielectric barrier discharges , which are used, for example, in the processing of plastic materials. Another example are arcs , such as those created during electrical welding .
Thermal equilibrium
An important characteristic of a plasma is to what extent it is in thermal equilibrium (TG):
- In complete thermal equilibrium , the heavy species ( molecules , atoms , ions) have the same temperature as the electrons and the plasma is also in radiation equilibrium with the environment, i.e. it emits cavity radiation .
- In the local thermal equilibrium (LTG or LTE), the particles of all types have approximately the same local temperature, which can change from place to place. However, there is no equilibrium with the radiation field. Characteristic spectral lines and continua deviating from the cavity radiation are therefore emitted. The state of the LTG can always be assumed when collision processes clearly dominate over radiation processes.
This case is e.g. B. in many technically used plasmas with temperature gradients, for example in lighting technology with medium and high pressure discharges.
In the case of plasmas, high plasma pressure or high plasma density do not necessarily have to be assumed for LTG. The dominance of collision processes can also be achieved through great turbulence, sufficiently strong collective effects - i.e. through strong interaction between the particles - or internal magnetic fields.
- In non-thermal plasmas, however, the electrons have a much higher temperature than the heavier species. These typically include low-pressure plasmas. Such a plasma is usually generated and maintained by supplying energy - mostly electrical energy - from neutral atoms of a solid or gas. The electrons can assume temperatures of several 10,000 Kelvin; the temperature of the ions and the neutral gas can at the same time be significantly lower, for example at room temperature. Such plasmas can be used to process workpieces (coating, plasma etching ) without overheating them. Thus, low-temperature plasmas are particularly suitable, for. B. for the surface modification of temperature-sensitive polymers .
Degree of ionization
The degree of ionization of the plasma is another characteristic property. The degree of ionization indicates the proportion of species that have given up electrons through ionization . If TG or at least LTG is present, the Saha equation describes the degree of ionization of this plasma as a function of the temperature, the density and the ionization energies of the atoms.
- Thermal plasmas with high temperatures (e.g. solar corona or fusion plasmas) are almost completely ionized.
- In the case of technically produced low-pressure plasmas, on the other hand, the degree of ionization is at most a few per thousand, and outside the thermal equilibrium they can no longer be described by the Saha equation .
- If the ion density of a plasma is known or can be determined using suitable methods, the degree of ionization of the plasma is simply the ratio of the ion density and the sum of the neutral particle and ion density.
- With a low degree of ionization, many effects in plasmas are determined by the collisions of ions and electrons on the predominantly present neutral gas atoms.
The charge carrier density of a plasma, determined by the degree of ionization and the gas pressure, determines the ability of electromagnetic waves to propagate in the plasma, see also ionosphere .
generation
A plasma can be obtained both by means of internal (e.g. sun) or external (e.g. technical gas discharges) energy supply. If the coupling of energy does not take place or if the energy losses - for example through heat conduction and / or through radiation emission - exceed the energy input, the plasma state is lost. Positive and negative charge carriers can then recombine to form neutral atoms , molecules or radicals .
The charge carriers can be generated by ambipolar diffusion z. B. on the walls of discharge vessels or in the vacuum of space. Ambipolar diffusion can take place even when the plasma state is stable.
In order to compensate for the loss of charged particles, those must be generated, which z. B. happens by impact ionization . Electrons with a sufficiently high kinetic energy are under certain circumstances (if there are appropriate cross-sections for the specific processes) able to knock electrons out of their compound when they collide with atoms, ions or molecules. Under suitable conditions, this process can take place as an avalanche effect , provided that after the collision one electron becomes two (plus one positive ion). In the case of technical plasmas, the spatial delimitation of the plasma can be problematic, because the high-energy particles of the plasma can damage walls, workpieces or electrodes through intense radiation or high-energy particles. In lighting technology, for example, the removal of electrode material is undesirable because of the resulting reduction in the service life of the illuminants. In the technical process of sputtering , however, the removal of material is used in a targeted manner.
Methods of supplying energy
Thermal stimuli
In the case of thermal excitation , the charge carriers are generated by impact ionization due to the movement of heat. Approx. 15,000 K are required at normal pressure to achieve almost complete ionization. The required temperature increases with increasing pressure. One possibility for this is irradiation with focused laser radiation . If the bundled laser beam hits a solid body, temperatures of several thousand Kelvin arise, so that thermal ionization takes place, which also spreads into the gas space above the surface. The resulting plasma in turn absorbs further laser radiation and intensifies the process. In the case of particularly short laser pulses, the phenomenon of self-focusing or shielding of the beam by the plasma can occur.
Chemical and nuclear reactions
If an exothermic reaction leads to strong heating of the gas, the impact ionization processes caused by the rapid movement of the molecules cause the transition to the plasma state. In response coming chemical combustion , nuclear fission and nuclear fusion in question.
Radiation excitations
When the plasma is excited by radiation , the charge carriers are generated by ionizing radiation . For this, the quantum energy or particle energy must exceed the ionization energy of the irradiated matter. This is already possible in gases with ultraviolet . X-rays and soft gamma rays, on the other hand, are hardly absorbed in gases. However, above a certain energy, pair formation takes place and ionization is effective. Beta and alpha rays have a high ionization potential .
Excitations from electrostatic fields
Electrostatic fields lead to discharges or to pre-discharges . Further ions are generated by electron impact ionization . Examples are thunderstorm lightning and electrostatic discharges .
DC voltage excitation
A sufficiently high electrical direct voltage is applied between two electrodes . With a suitable combination of voltage, electrode spacing and gas pressure, a flashover occurs and a discharge ignites between the electrodes. A distinction is made between gas discharges , spark discharges and vacuum sparks .
In all cases, a plasma is formed, which also enables the discharge to flow. If the current flow is sufficiently high, the electrodes heat up and the escape of electrons is facilitated, an arc is created . Arcs are used in electric welding and in arc lamps (arc lamps). They can also be operated with AC voltage.
The amount of voltage required to ignite a plasma depends on the distance between the electrodes, their shape and the gas pressure ( Paschen's law ).
Wire explosion
When a high current flow (e.g. from a capacitor battery ) through a thin metal wire, it evaporates explosively in a few microseconds to milliseconds. This creates a partially ionized metal vapor cloud and an arc discharge can ignite, which leads to further ionization of the metal vapor. First there is thermal excitation, then excitation by impact ionization. One area of application for the wire explosion is in the Z machine .
In order to prevent the rapid expansion of the plasma, this can take place in a non-conductive tube (capillary discharge).
Excitations from electromagnetic fields
When excited by electromagnetic fields , the charge carriers are generated by electron impact ionization . Very high intensity in the focus of a laser beam can also lead to the formation of a plasma in air (air breakthrough). The very high electric field strength of the waves is responsible. The energy input can be improved by cyclotron resonance .
Capacitive electrical excitation
A sufficiently strong alternating electric field is applied to two plates. A plasma forms between the plates in which charged particles oscillate back and forth at the frequency of the alternating field (high-frequency excitation). Charge carriers do not necessarily emerge from the plates. Which particles oscillate depends on their mass and degree of ionization. The frequency up to which a type of particle can resonate is called the plasma frequency .
The plates can also be attached outside the discharge vessel so that their field only reaches the plasma due to the capacitance of the wall. One then speaks of electrodeless excitation. In this way, contamination from the electrode material and wear on the electrodes are avoided. Some carbon dioxide lasers and discharge lamps with dielectric barriers work according to this principle . See also silent electrical discharge .
Inductive (magnetic) excitation
A high-frequency alternating current through an excitation coil surrounding a vacuum vessel induces ring-shaped currents in a plasma. The process is used in induction lamps and in gas phase deposition ( PECVD ) in pipes.
In tokamaks for nuclear fusion experiments, the plasma is heated in a ring-shaped vacuum container by a parallel, rising current and at the same time enclosed without contact by the strong ring-shaped magnetic field of a second, toroidally wound coil.
Microwave excitation
Here, microwaves from a magnetron are directed into the reaction chamber. The field strength of the electromagnetic wave must first be high enough to cause electrical breakdown and impact ionization. If the plasma is ignited, the field strength and impedance conditions change significantly - the adaptation conditions of the transmitting magnetron change.
Alternatively, atmospheric plasmas are generated by jets (or emitters) via transistor circuits (ranges 2–200 W) which can be easily regulated. Such cold plasmas are generated at frequencies above the plasma resonance (2.45 GHz) so that only the electrons in the plasma are accelerated. These microwave plasmas are often referred to as microplasmas.
Practical applications are plasma generators , plasma jets and plasma coating systems, chemical reactors, the sulfur lamp as well as the mercury-free energy-saving lamp and diamond synthesis .
Pinch effect
The current flowing through the plasma creates a magnetic field, which in turn constricts the plasma. This is known as the pinch effect . The plasma becomes denser and hotter. If the power source delivers high currents in the range of a few tens of kiloamps, very dense, hot and very strongly ionized plasmas can be generated that emit X-rays or in which even nuclear fusions take place ( tokamak ). The pinch effect is also the reason why a narrow channel for the current forms in lightning.
literature
- Michael A. Lieberman, Allan J. Lichtenberg: Principles of Plasma Discharges and Materials Processing. Wiley, New Jersey 2005, ISBN 0-471-72001-1 .
- RJ Goldston, PH Rutherford: Plasma Physics. An introduction. Vieweg, Braunschweig 1998, ISBN 3-528-06884-1 .
- K.-H. Spatschek: Theoretical Plasma Physics. An introduction. Teubner, Stuttgart 1990, ISBN 3-519-03041-1 .
- FF Chen: Introduction to Plasma Physics and Controlled Fusion. Plenum Press, New York 1983.
- Subrahmanyan Chandrasekhar : Plasma Physics. University of Chicago Press 1960.
- Eugene N. Parker : Cosmical Magnetic Fields: Their Origin and Their Activity. Clarendon Press, Oxford 1979.
- F. Cap: Introduction to Plasma Physics. I. Theoretical foundations. Vieweg, Wiesbaden 1984.
- Rainer Hippler, Sigismund Pfau, Martin Schmidt, Karl H. Schoenbach: Low Temperature Plasma Physics - fundamental aspects and applications. Wiley-VCH, Berlin 2001, ISBN 3-527-28887-2 .
- Vadim N. Tsytovich: Lectures on Non-linear Plasma Kinetics. Springer, Berlin 1995, ISBN 0-387-57844-7 .
- Hubertus M. Thomas, Gregor E. Morfill : Plasma crystals on board the ISS: complex plasmas in weightlessness. In: Physics in Our Time. 36, No. 2, 2005, ISSN 0031-9252 , pp. 76-83.
- Hannelore Dittmar-Ilgen: High-performance lasers with many perspectives. In: Naturwissenschaftliche Rundschau. 10, 2006, p. 549.
- H. Heuermann, St. Holtrup, A. Sadeghfam, M. Schmidt, R. Perkuhn, T. Finger: Various Applications and Background of 10-200W 2.45GHz Microplasmas. 60th International Microwave Symposium, Montreal, June 2012.
Web links
- Leibniz Institute for Plasma Research and Technology V. (INP Greifswald)
- Madgyver's Plasma Generator - Amateur Trials
- Making plasma
- Plasma portal of the VDI technology center
- Plasma technology. In: TechPortal. Archived from the original on September 18, 2013 .
- Competence network for industrial plasma surface technology INPLAS
- Link collection of the Weizmann Institute
- Record Set for Hottest Temperature on Earth: 3.6 Billion Degrees (Fahrenheit) in Lab
- From the TV show Everyday Life about experiments with plasma on the ISS
- Details about the mercury-free energy-saving lamp
- Nuclear fusion Max Planck Institute for Plasma Physics 2002 ( PDF )
- scinexx.de: Plasma - the fourth state of matter February 1st, 2019
Individual evidence
- ^ Wilhelm Pape , Max Sengebusch (arrangement): Concise dictionary of the Greek language . 3rd edition, 6th impression. Vieweg & Sohn, Braunschweig 1914 ( zeno.org [accessed on March 5, 2019]).
- ^ I. Langmuir: Oscillations in Ionized Gases. In: Proceedings of the National Academy of Science. Volume 14, 1928, pp. 627-637 ( PDF ).
- ^ Max Planck Society yearbook . 1991, ISBN 978-3-525-85397-9 , pp. 292 ( books.google.de ).
- ↑ With "visible matter" is meant here the matter that is not dark matter . No statement can yet be made about dark matter.
- ↑ Operative therapy: disc damage (discopathy). In: Health Lexicon, DocMedicus Verlag. Retrieved September 6, 2018 .
- ↑ ArthoCare ENT: Coblation: The gentle surgical method. (pdf) Retrieved September 6, 2018 .
- ↑ Rich P. Mildren: Dielectric Barrier Discharge Lamps . Macquarie University, Australia, Retrieved November 11, 2008.