Sun
Sun![]() |
|
---|---|
![]() |
|
The sun on June 7, 1992 | |
Observation data | |
Medium distance | 149.6 million km = 1 AU |
Smallest distance to earth Largest distance to earth |
147.1 million km 152.1 million km |
Apparent diameter | 31.5–32.5 ′ (arc minutes ) |
Apparent brightness ( V ) | −26.74 mag |
Physical Properties | |
Equatorial radius | 696,342 km = 1 solar radius ( R ☉ ) |
Mass ( M ) | 1.9884 · 10 30 kg ± 2 · 10 26 kg = 1 solar mass ( M ☉ ) |
Solar gravitational constant ( G · M ) | 1,327.124.400.41 10 20 m 3 / s 2 ± 1.0 10 10 m 3 / s 2 |
Medium density | 1.408 g / cm 3 |
Main components ( amount of substance in the photosphere ) |
|
Gravitational acceleration | 274 m / s 2 |
Escape speed | 617.3 km / s |
Rotation period (sidereal) | 25.38 days |
Inclination of the axis of rotation | 7.25 ° |
Luminosity | 3.846 10 26 W = 1 solar luminosity ( L ☉ ) |
Absolute brightness ( V ) | +4.83 mag |
Effective surface temperature | 5,778 K |
Spectral class | G2V |
Age | 4.57 billion a |
Planets | 8th |
![]() |
|
Photo montage to compare the size of the earth (left) and the sun. The core area ( umbra ) of the large sunspot is about 5 times the diameter of the earth. |
The sun is the star that is closest to the earth and forms the center of the solar system . It is an average-sized star in the outer third of the Milky Way . The sun is a dwarf star that is in the main sequence development stage. It contains 99.86% of the mass of the solar system. At 1.4 million kilometers, their diameter is around 110 times that of the earth. The sun's surface shows a changing number of sunspots that are related to strong magnetic fields . In addition to other phenomena, they are referred to as solar activity .
The solar radiation is one of the prerequisites for the development of life on Earth. The sun draws the energy for solar radiation from the fusion of hydrogen to helium .
The course of the sky of the sun divides the day and the year . In prehistoric times she was worshiped in this role in sun cults.
The astronomical symbol of the sun is ☉.
Etymology and name
The common Germanic female noun "sun" ( Middle High German sunne , Old High German sunna ) is based on the Indo-European root sāu̯el- (cf. also Latin sol , Lithuanian sáulė and Greek hḗlios ).
The name of the star is also in astronomy, as in colloquial language, simply "Sun", usually with the definite article, in English "Sun" (correctly with a capital letter, since it is a proper name). In science fiction novels and films - for example in Isaac Asimov's Foundation cycle - the Latin translation "Sol" (also with a capital letter) is occasionally used when the sun is mentioned as one of the many stars; this is supposed to form a parallel to other star names, which often come from Latin. This term is not used in modern astronomy.
Quantitative classification
The sun exceeds 700 times the total mass of all eight planets of the solar system and 330,000 times that of the earth , which fits in 109 times in diameter. With an energy emission that is 20,000 times the primary energy conversion per second since the beginning of industrialization, it falls into luminosity class V. A main sequence star like the sun thus releases more energy per second than all nuclear power plants on earth in 2011 in 750,000 years . The earth has an annual mean output of 1.367 kilowatts per square meter as extraterrestrial radiation .
The sun shines with a color temperature of around 5800 Kelvin . As a star of the spectral class G2V, it lies in the Hertzsprung-Russell diagram in the middle of the so-called main sequence , which represents all stars in radiation equilibrium. 1.4 to 1.5% of heavier elements in the convection zone (mass fraction, for the mole fractions see the box), the sun is considered the " metal-rich " and is one of the most numerous population I to. Like the solar system as a whole, it is around 4.57 billion years old. During this time it has converted around 14,000 Earth masses of hydrogen into helium by nuclear fusion , with 90 Earth masses of energy being released. Due to the accumulation of helium in the non-convective core - the mass fraction in the center is now 60% - it becomes more and more compact and includes more material, which slowly increases the luminosity and diameter of the sun. In about 7 billion years, the sun will turn into a red giant relatively quickly .
Perceived color
The maximum radiation of the sun is in visible light (by no means in infrared ) and is perceived by the human eye as pure white . However, if you look at the solar disk through a strong neutral density filter , you will usually see it as white-yellow or yellow, or as orange when positioned close to the horizon. This is explained by the fact that the short-wave (violet and blue) part of the visible solar radiation is mainly scattered by Rayleigh scattering in the earth's atmosphere and thus reaches the eye from a different direction than that of the perceived solar disk ( sky blue ).
If the chromatic adaptation of the eye is set to the overall perceived radiation (which appears as white when it is perceptible as a mixture - e.g. as a diffuse reflection on clouds -), the radiation still perceived from the direction of the sun itself becomes , unscattered visible radiation perceived as yellow or orange due to the (depending on the distance from the horizon less or more) reduced short-wave component. Outside the earth's atmosphere, when all light actually comes from the direction of the perceived solar disk, this appears to the human eye as pure white for this reason and regardless of the actual composition of the sunlight.
Lord Kelvin and the ages of the sun and earth
The fact that the age of the sun is measured in billions of years results consistently from modern star models and radiometric dating of rocks in the solar system. The persistence of solar radiation already became an urgent physical problem when Charles Darwin roughly estimated a duration of 300 million years for the erosion process of the southern English chalk . Lord Kelvin doubted Darwin's result, because in 1862 he identified the release of gravitational binding energy proposed by Hermann von Helmholtz as the most permanent energy source for solar radiation and, assuming that the mass of the sun is strongly concentrated towards the center, calculated a solar age of very probably below 100 million years ago. He later narrowed the cooling time of the earth's mantle to 20 to 40 million years. He witnessed, but did not publicly accept, that Ernest Rutherford proposed radioactive decay as a source of geothermal energy in 1904 . The energy released by the sun over geological time periods could only be explained by nuclear fusion from 1920 .
Physical structure
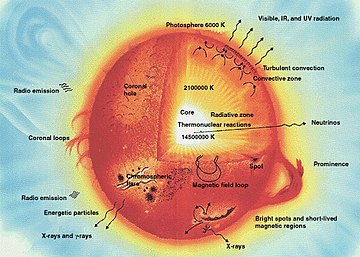
The sun consists of bowl-shaped zones, some of which can be sharply delimited. A rough division is the core zone as a fusion furnace, the inner atmosphere up to the visible surface and above that the outer atmosphere.
core
Half of the solar mass is concentrated within 25% of the solar radius , i.e. H. to about 1.5% of the solar volume. The acceleration due to gravity at the edge of this core zone is 8 times greater than on the surface of the sun and 220 times greater than on the earth's surface. The material thus puts itself under pressure : in the center it is 200 billion bar . Since the temperature there is comparatively low at 15.6 million K , the plasma can only apply the counterpressure necessary for stability through its high density , 150 g / cm³ in the center, 13 times the density of lead and 200 times that mean density of the inner atmosphere.
It is not the density that directly causes the back pressure, but the particle concentration, in the center almost 250,000 mol / ℓ . A good half of them are electrons , but due to the prevailing density-temperature conditions they have not yet degenerated . The radiation pressure also has a small share - so the gas law applies in the sun .
The particle density of the protons in the center is about 1000 times greater than in water. Since the frequency of the nuclear fusion reactions depends quadratically on the particle density and exponentially on the temperature, 99% of the fusion power of 3.9 · 10 26 W is released within the dense, hot core zone. The power density is higher within a narrower radius: half of its power is generated in a thousandth of the volume of the sun; that is an average power density of just under 140 watts per cubic meter, no more than in a compost heap. The great overall output of the sun is therefore more the result of the large volume and the high core temperature a result of the thick insulating layer.
The release of energy in the sun occurs through the proton-proton chain . In the first step, two protons fuse to form a deuterium nucleus. This reaction is very unlikely, on average a proton needs 10 10 years to react with another proton. This also explains the long lifespan of the sun.
The fact that the strongly temperature-dependent fusion reaction does not go through thermally and the sun explodes (or switches off) is due to the fact that additional heat does not make the interior of stars hotter, but colder, because the normal thermal expansion of the gas is increased by the gravitational pressure of the raised layers subsides. This negative feedback works very quickly because compression waves pass through the sun in well under an hour, see helioseismology .
Radiant zone and convection zone
Almost 2% of the fusion power is carried away by the neutrinos that are created . These only weakly interacting particles reach the sun's surface within a few seconds and the earth after a good eight minutes. The energy of the other reaction products thermalizes at the place of origin. The thermal radiation is in the range of soft X-rays and dominates the thermal conductivity of the material: in the center it has an intensity of around 3 · 10 21 W / m 2 . However, the individual photons only cover short distances before they are reabsorbed, not much longer than a few core distances. The short sections of the path add up criss-cross to form a random walk that takes between 10,000 and 170,000 years to the surface. In addition, since the energy “parks” by far the greatest amount of time in the thermal movement of the gas, the energy containment time is much longer, around 17 million years.
The radiation transport is efficient: At 25% of the radius, the energy flux density is 100 kW / cm 2 , but the temperature gradient is only about 0.1 K / m. The fact that this gradient, ten times steeper than in the earth's atmosphere, is not sufficient to drive convection , is due to the even steeper pressure gradient - a consequence of the high acceleration due to gravity, see adiabatic temperature gradient .
Outwardly, there is little change in the stability of the stratification, as the influencing factors partially compensate each other: the thermal radiation becomes weaker with decreasing temperature (see Stefan-Boltzmann law ), the material becomes more optically transparent with decreasing density, the power flow is distributed on a larger spherical shell surface and the acceleration due to gravity decreases.
Finally, there is an additional effect: the electrons, which are no longer quite as hot, begin to feel the individual nuclei, those with a high nuclear charge first, and even recombine for a short time. This prevents the radiation from spreading (increasing opacity) so that the temperature gradient becomes steeper again. At 71% of the radius it reaches the adiabatic value, the stratification becomes unstable. This defines the boundary of the so-called radiation zone. Above, the heat flow is increasingly transported convectively.
The further course of the opacity no longer influences the course of temperature and pressure, which are determined by the gravitational field and adiabatic, but the intensity of the convection. In large parts of the convection zone, the flow velocity is low, a few 10 m / s, and the convection cells are large and stable (months to years) and thus influenced by both the rotation of the sun and its internal magnetic field, see below.
In the area 20,000 to 1,000 km below the visible solar surface, free-free transitions at He + and H + also make a strong contribution to opacity. As a result, the convection becomes smaller and reaches speeds of over 1 km / s. This is the seething that can be seen as granulation with a telescope . The more intensive impulse transport in this area is noticeable in the radial course of the rotation rate.
Sun surface and surroundings
Just below the surface
The degree of ionization of hydrogen drops sharply at the upper limit of the above-mentioned range. According to the Saha equation , it is mainly dependent on the temperature. At a depth of around 1000 km, at a temperature of 10,000 K and a density of just under 1 g / m 3, it is still almost 80%, but at 6000 K and slightly lower density it is only a hundredth of it. This makes encounters between electrons and ions four orders of magnitude less frequent. Rupert Wildt found out in 1938 why the material has not long since become transparent (the energy of the photons is not sufficient to ionize hydrogen) : The neutral H atom can bind another electron with one twentieth of the binding energy and that also occurs at an even lower hydrogen ionization rate, since electrons from the ionization of metals are available.
Photosphere
Because the density decreases faster and faster - the scale height decreases with the temperature - the material finally becomes transparent and the photons can escape to the outside almost unhindered. This zone is called the photosphere, Greek for “ spherical shell of light”. The average depth from which the solar radiation escapes varies by a few 100 km depending on the wavelength and the exit angle. At the edge of the sun you can see a higher, colder layer at a shallower angle, which makes the edge appear darker , see the sun photo at the beginning of the article. A clear definition of the solar radius is therefore problematic, see star surface . By agreement, the solar radius is given as the one at which the gas temperature matches the energy flow density (63.18 MW / m 2 ). This effective radiation temperature is 5778 Kelvin. Due to the more directed radiation at shorter wavelengths, the color temperature of the solar radiation is slightly higher, at around 6000 Kelvin.
Chromosphere

Above the photosphere lies the chromosphere. The convection zone with its negative temperature gradient due to expansion of the gas (from just under 1 to 0.003 g / m 3 ) extends about 500 km into the chromosphere. Above a sharp minimum of 4100 K, radiation equilibrium results in a temperature of around 7000 K, while the density decreases to 10 −7 g / m 3 .
Radiation from the photosphere is absorbed to a small extent in the chromosphere and emitted again. Against the background of the photosphere, the Fraunhofer absorption lines arise in the solar spectrum, while in total solar eclipses the mostly almost 2000 km thick chromosphere can be seen for a few seconds as a reddish glowing line, its Greek name means “layer of paint”. Mass ejections of chromospheric density, numerous small spicules and less frequent protuberances (see below) glow in the same color.
Outside atmosphere
corona


The corona is located above the chromosphere . It passes into interplanetary space without any sharp boundary. Its “halo” (lat. Corona , “ crown ” , see picture on the left), which is visible in every total solar eclipse, astonished people thousands of years ago. It extends - depending on the solar activity and the exposure time - over one to two solar radii. In the corona, the influence of gas pressure on the movement of matter is negligible; magnetic fields and gravity rule.
The spectral lines of the corona could not be identified initially because they do not occur in terrestrial conditions. Since it was recognized that they come from highly ionized iron with only very few electrons, corresponding to temperatures of over 10 6 K, two hundred to five hundred times the photosphere temperature , there has been speculation about the heating mechanism of the corona. It can only get so hot because it is almost transparent in wide areas of the electromagnetic spectrum and emits only weakly; a consequence not only of the low density, but also of the high temperature: the free electrons are so fast that they can barely perceive the more common, light elements, especially hydrogen and helium, even though they are completely ionized. Further loss mechanisms (see below) are the release of heat to the comparatively cold chromosphere and, especially in the area of coronal holes , the formation of solar wind .
A weak X-ray continuum is created on the rare, but often charged, heavier ions, which enables the corona to be observed in front of the photosphere, which is dark in the hard X-ray light, see picture above on the right. Limited to narrow emission lines, this is also possible with less hard radiation, see picture on the right. It comes from the TRACE satellite , which specializes in observing the sun in the extreme UV range , with high spectral and spatial resolution.
Transition region
XUV emission lines from less highly ionized species, such as C IV, O IV, O VI, S VI, originate from a narrow transition region , the border between the corona and the chromosphere, with temperatures between 10,000 and 700,000 K. There are two sharp temperature jumps ( corresponding to the ionization of hydrogen and helium), which cannot be spatially resolved in the foreseeable future. It is possible that the local velocity distribution of the electrons is also non-thermal there. Over the transition region, which is a few 100 km thick, the density also changes by three orders of magnitude, from 10 −7 to 10 −10 g / m 3 . The hot corona burns itself, as it were, into the chromosphere and ultimately fails due to the radiation losses that increase with the square of the density. The shape of the transition region adapts to the dynamic processes on the sun's surface - the main influencing factors are the density of the structures and the heat output in the corona.
Observations with TRACE suggest that the heating mechanism of the corona must be in its lower area, near the transition region, because the plasma arcs, the density of which is much greater near their base points than in the vertex, are hot up to the base points and shine brightly there.
Solar wind

In the corona, probably in connection with the heating mechanism in the lower corona, the solar wind is created , a supersonic flow mainly of protons and electrons. In coronal holes , especially in the polar regions, with high solar activity but also numerous near the equator, there is hardly less solar wind than in the denser areas of the corona, especially streamers , but it flows faster, at 800 km / s instead of 300 km / s. Eruptive prominences produce large quantities and high velocities, and if they hit Earth, cause geomagnetic storms .
Dynamic properties
Rotation, magnetic field and sunspots
The movement known since ancient times sunspots shows that the sun is not flat, but a rotating ball: You walk every day, close to the edge seemingly slower and with foreshortened form and durable spots appear even after two weeks on the eastern edge again . The sun follows the main direction of rotation in the solar system ( clockwise ). Around 1860, Richard Christopher Carrington discovered that spots near the equator move faster than spots at higher latitudes ( differential rotation ). For the indication of longitudes on the sun, he introduced a reference system that rotates 360 ° in 25.38 days ( sidereal , synodic on average about 27.2753 days). This corresponds to the movement of the spots at about 26 ° latitude.
Today the rotation of the sun's surface is much more precise and also in latitudes where spots are rare, determined by the shift of spectral lines through the Doppler effect , see solar rotation . The comparison with the movement of the sunspots shows that they are moving westward faster than the surface. This fits in with the idea that the magnetic fields that cause the spots are “anchored” below the surface and that deeper layers rotate faster due to the conservation of angular momentum . The necessary radial impulse transport is given by the violent, isotropic convection in the upper part of the convection zone (up to a depth of about 4% of the sun's radius). The more complex convection at greater depths is responsible for the slower polar rotation.

In the early 1990s, helioseismic measurements showed that the radiation zone rotates uniformly with a period of just under 27 days. The tachocline said transition area to the differentially rotating convection is very flat with few percent the solar radius. The angular velocity gradients are correspondingly steep there. The position and thickness of the tachocline, the formation of the sun's magnetic field there and the course of the differential rotation within the convection zone are theoretically not yet understood.
The high electrical conductivity of the plasma inside the sun - it corresponds to that of copper at room temperature - causes a strong coupling of the magnetic field and matter, see magnetohydrodynamics . At high density the material conducts the magnetic field, at low density it is the other way around. In the convection zone, the differential rotation means that the field lines there no longer run in the NS direction, but rather coiled in the EW direction, which greatly increases the magnetic tension . It is reduced by reversing the polarity every 11 years. The so-called activity of the sun also fluctuates in this rhythm . When the magnetic tension is high, the magnetic field breaks out of the sun and forms arcs in the corona. Entrained material is visible in emission as a protuberance ; in front of the light pane these arcs appear as dark filaments in visible light.
The magnetic field on the sun's surface can be observed spectroscopically: spectral lines of elements that are normally observed at a uniform wavelength appear to be divided into three parts when a magnetic field is present (normal Zeeman effect ), the distance between these lines being proportional to the strength of the field. Where the magnetic field strength is particularly high in the photosphere, the field hinders convection, the surface cools to 3700 to 4500 K and is less bright, which is perceived as sunspots. The field strength in the vicinity of the sunspots can be up to 0.4 Tesla (4000 Gauss) and is therefore up to ten thousand times stronger than the terrestrial magnetic field on the earth's surface. These local magnetic fields are also responsible for the coronal mass ejections emanating from sunspots .
In these protuberances, the field strength can only rarely be measured due to the low density and has recently proven to be greater than expected.
The large-scale magnetic field of the calm sun can only be roughly described by a dipole field . It is associated with an electrical current on the order of 10 12 amps circulating in the sun . The field strength of this dipole field on the sun's surface is around 100 µT (1 Gauss ), only about twice as strong as the earth's magnetic field on the earth's surface.
A similar winding with field reinforcement happens with the magnetic field carried along by the solar wind in interplanetary space . As a result, on the one hand, the solar wind carries much more angular momentum with it than with free, radial movement. This explains how the sun has been able to give off a large part of its angular momentum since its formation without giving off much mass - currently only about 10 9 kg / s. On the other hand, the heliospheric current layer is created in the form of the "Parker spiral", whereby the magnetic field strength decreases more slowly than would be expected with a dipole field (the field strength is a few nT away from the earth). Finally, the propagation speed of the shear Alfvén waves falls below that of the solar wind, so that the solar wind then spreads radially and carries the magnetic field with it. This limit at around twenty solar radii is considered to be the beginning of the heliosphere , which extends to the heliopause , where the solar wind meets interstellar matter .
Vibrations

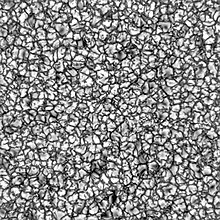
The strong convection near the sun's surface causes pressure fluctuations. If the frequencies were n't so low - 2 to 7 mHz, corresponding to the typical lifespan of the granulation of five minutes - it would sound like the rustling of the forest in the wind. The pressure fluctuations run into the sun as sound waves , and because the speed of sound increases there with the temperature , they return in an arc to the surface, where the density jump reflects them again. In this way, the waves run around the sun several times and are superimposed to form standing waves with a characteristic frequency depending on the oscillation pattern.
These oscillations can be made visible with spectroscopic methods: They move the photosphere slowly up and down and the component of the speed in the direction of observation shifts the absorption lines of the solar spectrum due to the Doppler effect . The speed amplitudes of the vibrations are, however, a maximum of a few meters per second, which is not easy to prove due to the strong Doppler broadening of the spectral lines. However, by averaging the measurement results over many months, it was possible to identify numerous vibration modes and determine their frequencies down to fractions of a μHz. The different modes are dependent on the speed of sound at different depths to different degrees, so that a common evaluation of all modes allows the determination of the depth dependency of the speed of sound.
The natural vibrations of the sun are observed and analyzed by helioseismology . Important results concern
- the confirmation of the solar model at the time of the solar neutrino problem ,
- the measurement of the differential rotation in the convection zone,
- the discovery of the nearly rigid rotation of the radiation zone and
- the observation of active regions on the far side of the sun.
Optical phenomena and observation
Optical appearances
If you look at the sun from space , it appears white. Their usual yellow color is explained by the influence of the earth's atmosphere. Short-wave (blue) light is scattered much more strongly by the air molecules (nitrogen, oxygen, noble gases and carbon dioxide) than long-wave (red) light. Thus, the sky shines diffusely blue, but sun rays that hit the earth's surface appear yellow. The longer the path that the sun's rays cover on their way through the atmosphere, the more blue light is scattered out. The low sun therefore appears strongly reddish.
The sun can only be seen with the naked eye when the sky is hazy, shortly after sunrise or shortly before sunset . The earth's atmosphere absorbs most of the light, especially UV radiation. However, the atmosphere near the horizon also greatly reduces the image quality and causes a vertical compression of the sun image as a result of the refraction of light . The fact that the setting sun looks larger near the horizon is not, as is often assumed, a consequence of the refraction in the air layers, but an optical illusion that is examined and explained by perceptual psychology under the term moon illusion .
Although all phenomena in atmospheric optics are directly or indirectly linked to sunlight, many of them appear directly next to or with the sun. This applies primarily to sunrise and sunset , but also to almost all halo phenomena , such as the 22 ° halo, the secondary suns or pillars of light . A special phenomenon that has coined the term “sun rays” are the bundles of rays . Green flashes are very rare .
Observation of the sun
With telescopes can be activities of the sun in the form of protuberances and sunspots visible. Violent outbreaks, so-called flares , can also be observed there , which can be identified as lighter and thus hotter areas even with small instruments. Viewed from the earth, the solar disk has a diameter of about 32 arc minutes , the exact size of which depends on the current distance between the earth and the sun. The sun disk appears largest in perihelion and smallest in aphelion . The apparent size difference in their diameter between aphelion and perihelion is just over three percent. The easiest way to observe the sun is to project the eyepiece image from a telescope or binoculars onto a white surface (for example a canvas or a piece of cardboard). This image of the sun can be viewed safely. This process is called eyepiece projection . Direct observation with or without a telescope can lead to irreversible blindness due to the bright solar radiation .
Observation is also possible with the help of special solar filters , these are foils or coated glasses that are held in front of the eye or attached to the lens . A detailed observation is also possible with a Herschel prism . This only works on a refractor .
In all of the observation methods described, the entire spectrum of sunlight is attenuated, the sun is observed in "white light". Sunspots, flares and granulation become visible.
Special components or telescopes are required to observe protuberances. With a protuberance approach , the sun is covered by a disc - an artificial total solar eclipse is created, so to speak. The prominences rising at the edge of the sun are observed through a so-called H-alpha filter . This is a particularly narrow-band interference filter that only lets through the deep red light of the excited hydrogen.
H-alpha telescopes enable observation of the entire surface of the sun in this spectral range . With this, protuberances, filaments, spots and flares can be observed. These telescopes have become very inexpensive in recent years and are increasingly being used by amateur astronomers .
The corona can only be observed during a total solar eclipse or by means of a special device, the coronograph .
Evolution of the sun
phase | Duration in millions of years |
Luminosity (in L ☉ ) |
Radius (in R ☉ ) |
---|---|---|---|
Main sequence star | 11,000 | 0.7 ... 2.2 | 0.9 ... 1.6 |
Transition phase | 700 | 2.3 | 1.6 ... 2.3 |
Red giant | 600 | 2.3 ... 2300 | 2.3 ... 166 |
Beginning of He-burning | 110 | 44 | about 10 |
He cup burning | 20th | 44 ... 2000 | 10… 130 |
Unstable phase | 0.4 | 500… 5000 | 50 ... 200 |
Transition to white dwarf with planetary nebula |
0.1 | 3500… 0.1 | 100 ... 0.08 |
The solar system was formed 4.6 billion years ago through the gravitational collapse of an interstellar gas cloud (→ star formation ). The subsequent history of the development of the sun leads via its current state ( yellow dwarf ) to that of a red giant and finally via an unstable final phase at the age of about 12.5 billion years to a white dwarf , which is surrounded by a planetary nebula .
This process can be modeled on the computer using the laws of physics and knowledge of core physical processes from laboratory experiments . The characteristics of the individual phases are given in the table (Sackmann, 1993). The index zero marks the current state variables of the sun, i.e. at the age of 4.6 billion years.
It is possible that the sun was formed in an open star cluster along with many other stars. This star cluster disintegrated after about 100 million years. Today the individual members are scattered all over the Milky Way . In 2014 a star was found with HD 162826 , which is chemically very similar to the sun and thus could be a solar sibling from the same original star cluster.
Protostar
The transition from a pre-stellar compression with planetary dimensions to a protostar clearly separated from the rest of the gas and dust cloud began with the thermal dissociation of the hydrogen , which absorbed energy at a temperature of a few 1000 K in the core area and allowed it to compress more quickly. The still light protostar obtained its rapidly increasing radiation output from the collapse of further mass, then only from its own contraction, because it had blown away the rest of the mass in its surroundings - apart from the planetesimals that condensed from it .
The contraction of the core zone of the early sun ended after tens of millions of years due to the onset of nuclear fusion.
Main sequence star
It took about the same time for the state variables in the inner atmosphere to become stationary with the shell structure shown above. This went hand in hand with the approach to the main series . Since then, the mass fraction of hydrogen in the convection zone has increased by a few percentage points by diffusing upwards at the lower limit of the convection zone through the slowly sinking heavier elements. The relative frequencies of the 'metals' have not changed as a result. In the main sequence stage, the sun lingers for eleven billion years. During this time, the luminosity increases three times from 0.7 L ☉ to 2.2 L ☉ and the radius almost doubles from 0.9 R ☉ to 1.6 R ☉ . At the age of 5.5 billion years, i.e. in 0.9 billion years, the mean temperature on the earth's surface exceeds the value of 30 ° C, which is critical for higher living beings. Another billion years later, 100 ° C will be reached. At 9.4 billion years old, the hydrogen in the sun's center dries up, and the fusion zone shifts into a bowl-shaped area around the center that moves outward over time. However, this process does not initially lead to a change in the externally visible solar parameters.
At the age of 11 to 11.7 billion years, the burned-out core zone of helium condenses. The associated rise in temperature increases the energy consumption in the hydrogen shell. The solar radius increases to 2.3 R ☉ . The sun becomes more reddish and begins to move away from the main sequence in the Hertzsprung-Russell diagram . Up to this point the total loss of mass due to solar wind is less than one per thousand.
Red giant
At a solar age of 11.7 to 12.3 billion years, a dramatically accelerated increase in luminosity and radius begins. At the same time, the surface temperature decreases and the radiation spectrum shifts towards the red area (see thermal radiation ). In the final phase of this development, the sun reaches a luminosity of 2300 L ☉ and a radius of 166 R ☉ . This corresponds roughly to the radius of the orbit of Venus . Venus and Mercury will be destroyed. Seen from the earth, the sun now takes up a large part of the sky and the earth's crust is melted into a single lava ocean. Due to the low gravity on the sun's surface, the sun loses 28% of its mass in this phase through solar wind, a proportion of up to 1.3 · 10 −7 M ☉ flows into space as interstellar gas per year. Due to the lower solar mass, the force of attraction on the planets also decreases, so that their orbital radii increase by 38% each.
Helium flash and burn phase
Since in the core zone of the sun no more fusions take place and thus no more energy is released, it gives way to gravity and contracts until finally the density there has risen to around 10 6 g / cm 3 , 10,000 times the current value . As a result, the temperature there eventually rises to 10 8 K.
At this temperature, the fusion of helium to carbon begins. Due to the extreme density in the center and the associated neutrino cooling, this fusion reaction initially ignites within a hotter spherical shell-shaped zone around the center. Usually the energy released would lead to an expansion of the core, which stabilizes the temperature. However, the core zone is in a special quantum mechanical degeneracy state , which means that the energy is first invested in the dissolution of the degeneracy. Therefore, no stable state initially is possible so that the helium fusion in the form of a gigantic explosion uses that as a helium flash ( helium flash is called). The solar output increases to 10 10 L ☉ for several seconds . This corresponds to about 10% of the luminosity of the entire Milky Way. Only after a conversion of 3% of the helium reservoir does an expansion begin and this performance excursion stops. This explosion only takes place in the central area and is initially not noticeable externally. However, it pushes the hydrogen fusion zone further outwards, the temperature of which therefore decreases and with it the energy conversion. Paradoxically, as an external consequence of the helium flash, the luminosity will decrease by almost a factor of 100 within the next 10,000 years. This is followed by a phase of one million years in which the solar parameters oscillate until a stable state of helium fusion is established in the center, which then lasts for 110 million years. At the same time, the bowl-shaped hydrogen fusion zone also migrates further outwards. During this time the luminosity remains almost constant at 44 L ☉ and the radius at 10 R ☉ .
Helium shell burning
Then the helium in the sun's center is also used up and a phase of helium shell burning begins, which lasts 20 million years. There are now two nested, bowl-shaped fusion zones. Carbon collects in the center and contracts gravitationally. This is associated with another enormous increase in luminosity to 2000 L ☉ and an increase in radius to 130 R ☉ . Towards the end, the sun loses a mass fraction of 0.1 M ☉ .
In the last 500,000 years of this phase, one expects further unstable situations in connection with the interaction between the contracting core and the helium fusion zone, in which short-term power excursions through helium fusion with about 10 6 L können can occur. A likely scenario would be, for example, four such helium flashes about 100,000 years apart. As a result of each of these helium flashes and the associated expansion of the hydrogen shell, the fusion there may temporarily come to a complete standstill for the next 200 years. The external consequence of a helium flash would be a decrease in luminosity. After 400 years the energy of the helium lightning reaches the surface. Luminosity and radius increase and relax again over the next 10,000 years. Variations in luminosity between 500 L ☉ and 5000 L ☉ and radius variations between 50 R ☉ and 200 R ☉ are expected . In the phases of maximum expansion, the sun's surface reaches up to today's earth's orbit. Only because of the increase in the diameter of the earth's orbit does the earth escape total annihilation. At the same time the sun comes in these phases overall mass of further 0.05M ☉ from.
White dwarf and planetary nebula
As a result of the aforementioned mass losses, the sun loses its entire outer shell, including the hydrogen and helium fusion zone. Around 100,000 years after the last helium flash, the hot inner core is therefore exposed, which essentially consists of highly compressed carbon and oxygen. Its radius is only 0.08 R ☉ , but its surface temperature is 120,000 K. Its luminosity is initially 3500 L ☉ . Due to the high temperature, this radiation contains an enormous amount of ultraviolet radiation , which now stimulates the expelled gas cloud from the sun to glow. Since the speed of the solar wind is constantly increasing, the gases emitted earlier are caught up by the later ones and often compressed into a spherical gas shell. To an outside observer, the glowing gases appear in this case as a ring, which is referred to as a planetary nebula . When the gas evaporates, this phenomenon disappears after a few 10,000 years, and the shining remainder of the sun, known as the white dwarf, remains in the center.
It is only about the size of the earth, but has a mass of 0.55 M ☉ . Its density is therefore about one ton per cubic centimeter. It has no internal energy source, so its radiation leads to a loss of heat. After a comparatively rapid cooling in the initial stage due to the extreme luminosity, the surface temperature drops to values at which radiation is possible for several dozen billion years due to the significantly lower luminosity before the sun goes out completely as a black dwarf in the optical spectral range .
Cosmic environment
The sun is currently traversing an area about 30 light-years in size, known as a local cloud or local flake because of its increased density . Also in the local flake are the neighboring stars Altair , Wega , Arktur , Fomalhaut and Alpha Centauri . The local flake for its part is embedded in a largely dust-free region with a lower particle density, the local bubble . The local bubble extends at least 300 light years in the direction of the galactic plane . It is located near the inner edge of the Orion arm of the Milky Way. It is about 6500 light years to the neighboring arm of Perseus , and about 28,000 light years to the center of the galaxy. One orbit, with about 250 km / s, lasts 210 million years (Galactic year).
The sun also traverses the Gouldian Belt , a large-scale arrangement of young stars (around 20–60 million years old) and star formation regions with an extension of more than 2000 light years. Since these stars are much younger than the sun, it cannot belong to the objects of the Gouldian belt.
Exploring the sun
Early observations
As the most important celestial body for earthly life , the sun enjoyed careful observation of people even before history was written . Places of worship like Stonehenge were built to determine the position and course of the sun, especially the times of the solstices . It is believed that some older sites were also used for solar observation, but this is not certain. The daily course of the sun and its seasonal fluctuations as well as solar eclipses have been carefully observed and documented by different cultures. Records from ancient China confirm the observations of particularly violent sunspot activity. Sunspots can be seen with the naked eye when the sun is low on the horizon and the sunlight is "filtered" by the dense earth's atmosphere.
Observations with telescopes
At that time, sunspots were also noticed in Europe, although they were considered to be “atmospheric vapors”. It was not until the development of the telescope that systematic research into the phenomenon was carried out. In 1610, Galileo and Thomas Harriot first observed the spots using a telescope. Johann Fabricius was the first to describe them in a scientific treatise in 1611. He correctly attributed the observed migration of the spots on the solar disk to the sun's own rotation. In 1619 Johannes Kepler postulated a solar wind, as the tail of comets is always directed away from the sun. In 1775 Christian Horrobow already suspected that sunspots are subject to a certain periodicity.

In 1802, William Hyde Wollaston demonstrated for the first time dark lines ( absorption lines ) in the solar spectrum . Joseph von Fraunhofer systematically examined the lines from 1814 onwards, which is why they are also known as " Fraunhofer lines ". In 1868, during a solar eclipse , Jules Janssen found a line of the then unknown helium, which got its name from the Greek name of the sun.
In 1843, Samuel Heinrich Schwabe published his discovery of the cycle of sunspot activity. In 1849 the sunspot relative number was introduced, which shows the number and size of sunspots. Since then, the spots have been observed and counted regularly. In 1889 George Ellery Hale developed the spectroheliograph . Henry Augustus Rowland completed an atlas of the solar spectrum in 1897, which contains all spectral lines. In 1908, George Ellery Hale discovered the splitting of spectral lines in the area of sunspots by magnetic forces ( Zeeman effect ). In 1930, Bernard Ferdinand Lyot observed the solar corona outside of total darkness.
In 1960 the vibration of the photosphere was detected. This was the beginning of helioseismology , which investigates the natural vibrations of the sun and derives the internal structure and processes from it.
In the course of time, special solar observatories were set up, which are used exclusively for observing the sun.
Other observation methods
In 1942, James Hey determined that the sun was a radio source . In 1949 Herbert Friedman demonstrated solar X-rays .
Huge underground detectors were set up to measure the solar neutrinos . The discrepancy between the theoretical and actually measured neutrino flux has led to the so-called solar neutrino problem since the 1970s : only about a third of the expected neutrinos could be detected. This allowed two possibilities. Either the solar model was wrong and the expected solar neutrino flux was overestimated, or the neutrinos can transform into a different "species" on their way to earth ( neutrino oscillation ). The first indications for this neutrino oscillation were found in 1998 on the Super-Kamiokande and have since been generally confirmed.
Exploration by satellites and spacecraft

A number of satellites were sent into orbit to observe the sun. In particular, the satellites can be used to investigate wavelength ranges ( ultraviolet , X-rays) that would otherwise be absorbed by the earth's atmosphere . For example, the Skylab space station , launched in 1973, had an X-ray telescope on board.
With the help of space probes one tried to get closer to the sun in order to be able to study the surroundings of the sun. This was and remains a technically very difficult undertaking due to the very high temperatures and intense radiation. The German-American Helios probes launched in 1974 and 1976 were only able to approach the sun up to 43.5 million kilometers.
The Ulysses spacecraft, launched in 1990 , had other goals. She should study the poles of the sun, which are not visible from the earth or from space probes moving in the planetary plane. This could only be achieved with a steeply inclined orbit plane of the space probe. For this purpose, Ulysses first flew to the giant planet Jupiter , where the orbital plane of the probe was changed by means of a swing-by maneuver. As a result, Ulysses was able to leave the planetary plane and since then has already flown twice over the two poles of the sun. With conventional rocket propulsion, without the Jupiter flyby, such a mission would have been much more expensive.
In 1995 the SOHO probe, mostly built by Europe, was launched in the direction of the sun. SOHO is now at Lagrangian point L1 and observes the sun with twelve different instruments. It provides daily images of the sun and thus contributes significantly to the prediction of solar flares and storms. The TRACE satellite followed in 1998 to support SOHO.
The Genesis space probe was launched in 2001 and shortly thereafter moved to a position at Lagrangian point L1, where it collected samples of the solar wind for 2.5 years , which were then to be brought back to Earth. This should determine the exact isotopic composition of the solar wind. In September 2004 the capsule with the samples entered the earth's atmosphere, but hit the earth hard due to an undeployed parachute. Some of the samples survived the impact and are currently being studied by scientists.
The two STEREO space probes started on October 26, 2006 and for the first time deliver a three-dimensional image of the sun and its surroundings. For this purpose, one probe was stationed at Lagrangian point L4 and one at Lagrangian point L5.
On February 11, 2010, NASA launched the Solar Dynamics Observatory (SDO) as the SOHO successor. It is used to research the dynamic processes of the sun and includes the instruments EVE ( measurement of extreme UV radiation ), HMI (detection of helioseismic and magnetic activities) and AIA (high-resolution detection of the solar atmosphere in different wavelength ranges).
China is planning to launch a total of three space probes, which are to examine the sun-earth system more closely in the KuaFu research mission .
In 2018, NASA successfully launched the Parker Solar Probe space probe , which is expected to approach the sun's surface to within 8.5 radii (about 6 million kilometers). It should help answer the following questions:
- How is the corona heated up to five million degrees even though the visible surface of the sun is only around 5500 ° C?
- How are the particles of the solar wind accelerated?
For February 2020, the European Space Agency (ESA) and NASA launched the Solar Orbiter space probe , which is expected to approach the sun to within 0.28 austronomic units (about 42 million kilometers). Above all, the sun-near heliosphere, the solar atmosphere and the formation of the sun's magnetic field are to be investigated.
Cultural history
The sun is the central star in the sky, all life on earth depends on it. People have been aware of this paramount importance for ages. Many earlier cultures worshiped her as a deity. The regular daily and annual return of the sun was sometimes fearfully expected and evoked by means of cultic or magical rituals . Solar eclipses in particular caused great consternation and fear. In ancient China , it was believed that a dragon devoured the sun. They tried to make the beast to release the sun again by making a lot of noise. On the other hand, mankind made the knowledge of the day and year periods, which are fundamental for all life, usable from the earliest times. The sun is - via the rotation of the earth - the natural clock of man, and the sequence of the seasons led to the development of the calendar , which was vital for all cultures , especially after the development of agriculture .
For the Sumerians , the sun embodied the sun god Utu . With the Babylonians it corresponded to the god Shamash , who entered the sky every day and whose rays remained nothing hidden. In ancient Egypt , Ra (also Re or Re-Atum) was worshiped as the sun god. For a short time the “heretic” - Pharaoh Akhenaten only allowed Aton , the personified solar disk, as the only god and abolished the worship of all other Egyptian gods. In China the sun stood as a symbol for east, spring, masculinity ( yang ) and birth as well as for the emperor.
In ancient Greece , the sun god Helios was worshiped, and he drove his sun chariot across the firmament every day. However, the first considerations in which the sun is viewed as a physical object have been handed down from ancient Greece . Probably the oldest of these hypotheses comes from Xenophanes , who named the sun as a fiery evaporation or cloud. This represented a great cultural and historical change, because the perception of the sun as a natural object was on a different level than the previous conception of the sun as part of a divine entity, which was also represented in the later centuries. It was from these very thoughts that the first critical examination of the humanized image of the gods of ancient Greece emerged (“If the horses had gods, they would look like horses”) and the first thoughts on monotheism as a result . With Xenophanes the sun appeared for the first time in European history as an object of physics; one could understand this as the hour of birth of astrophysics . Xenophanes' theses were later adopted by other Greek philosophers. For example, the pre-Socratic Anaxagoras described the sun as a glowing stone. These views did not prevail in the following among all thinkers and many later schools fell back on more mythical explanations.
The Greek god Helios largely corresponded to the Roman god Sol invictus , whose cult was widespread from the imperial era until the beginning of late antiquity . The sun is adopted from antiquity as a symbol of vitality in astrology .
In Norse mythology, the gods formed the sun from a spark and put it in a chariot. The goddess Sol drives a chariot across the sky, pulled by the horses Alsvidr and Arvakr . The team is constantly followed by the wolf Skalli (Skoll). On the day of the end of the world ( Ragnarök ) the wolf will devour the sun.
In early Mexico , the sun god Tonatiuh was worshiped by the Aztecs . The Maya and the Inca were Itzamná or Inti , the main deities.
Observing the sun and other stars and determining their orbit points ( equinox , summer and winter solstice ) was a prerequisite for creating calendars. This enabled important seasonal events to be determined in advance, such as the arrival of the Nile floods in ancient Egypt, the most favorable time for sowing or the arrival of the autumn storms that are dangerous for seafaring. Pre-Christian places of worship such as Stonehenge were evidently built for such observation purposes. The Stonehenge facility is designed in such a way that on the morning of Midsummer's Day , when the sun reaches its highest northern position, the sun rises directly over a position stone ("heel stone") and the sun's rays penetrate in a straight line into the interior of the structure. The Bronze Age Sky Disc of Nebra also seems to have been an instrument for observing the sky. Your golden edges will be u. a. interpreted as "sun boats", a religious symbol of the Bronze Age. The Trundholm sun chariot , in which the disc is interpreted as a sun symbol with a day and night side , also falls at the same time .
The geocentric worldview of antiquity, as it is handed down by Ptolemy , saw the earth as the center of the universe. The sun, moon and the planets moved on circular paths around the earth. This idea lasted for almost 2000 years. However, it had weaknesses: The movement of the planets that could be observed with the naked eyes could only be explained by complicated auxiliary constructions of the epicyclic theory . Aristarchus of Samos already postulated in the 2nd century BC That the sun is the center of the world. The scholars Nikolaus von Kues and Regiomontanus took up this idea again more than 1500 years later. In his work De revolutionibus orbium coelestium, Nicolaus Copernicus tried to create a mathematical basis for what he did not succeed. However, his work stimulated further research, including by Galileo Galilei . As a result, the heliocentric view of the world gradually established itself , which regards the sun as the center of the universe.
The further advances in astronomy showed that the sun does not occupy a prominent position in the universe either. Rather, it is one of a few hundred billion stars in the Milky Way , which in turn is part of even larger structures in the cosmos .
literature
- before 1711: Josef Langer : Theoria motuum Solis et Lunae.
- 1990: Helmut Scheffler, Hans Elsässer : Physics of the Stars and the Sun. BI-Wissenschaftsverlag, Mannheim 1990, ISBN 3-411-14172-7 .
- 1990: Rudolf Kippenhahn : The star we live on. DVA, Stuttgart 1990, ISBN 3-421-02755-2 .
- 1993: I.-J. Sackmann et al: Our Sun. T 3. Present and Future. In: Astrophysical Journal. University of Chicago Press, Chicago Ill 418.1993, 11 (Nov.), 457-468, bibcode : 1993ApJ ... 418..457S .
- 1995: Wolfgang Mattig: The sun. CH Beck, Munich 1995, ISBN 3-406-39001-3 .
- 1996: Kenneth R. Lang: The sun - star of our earth. Springer, Berlin / Heidelberg / New York, NY 1996, ISBN 3-540-59437-X .
- 1997: F. Herrmann, H. Hauptmann: Understanding the stability of stars by means of thought experiments with a model star . At the. J. Phys. 65, 292-295 (1997)
- 2002: Wolfgang Mattig: Before solar observation became solar physics - in Germany and the surrounding area. (PDF; 61 kB) In: SONNE. Bulletin of the amateur sun watchers. Berlin 2002, 103, 67 (online - pdf). ISSN 0721-0094 .
- 2004: C. Bounama, W. v. Bloh, S. Franck: The end of the spaceship earth . In: Spectrum of Science . No. 10. Spectrum, October 2004, ISSN 0170-2971 , p. 52-59 .
- 2004: Michael Stix: The Sun - An Introduction. Springer, New York, NY 2004, ISBN 3-540-20741-4 .
- 2008: Thorsten Dambeck: The cauldron in the solar furnace (PDF; 2.0 MB) in: MaxPlanckForschung , 1/2008, pp. 28–33, ISSN 1616-4172
- 2013: Ulrike Feist: Sun, Moon and Venus: Visualizations of Astronomical Knowledge in Early Modern Rome (= Actus et Imago , Volume 10). Akademie-Verlag, Berlin 2013, ISBN 978-3-05-006365-2 (Dissertation University of Augsburg 2011, 259 pages).
- 2013: Elmar Schenkel, Kati Voigt (eds.): Sun, moon and distance: space in philosophy, politics and literature , PL Academic Research, Frankfurt am Main 2013, ISBN 978-3-631-64081-4 .
Web links
- Website of the Kiepenheuer Institute for Solar Physics , Freiburg
- Internet presence of the optical solar physics at the Astrophysical Institute Potsdam
- Images of the sun ( NASA , English)
- www.sonnenbeobachtung.de - Information on observing the sun with a picture gallery
- Internet presence of the Solar and Heliospheric Observatory (SOHO, English)
- Sun at high performance ( Memento from June 11, 2007 in the Internet Archive ) at www.weltderphysik.de .
- Karl-Otto Eschrich: The riddle of the magnetic fields of the sun and earth - Hommage à Fritz Krause (PDF; 56 kB), UTOPIE Kreativ, issue 208, 2008, pp. 177–181.
- Sounds of the Sun (NASA)
Videos
- Where does the sun get its energy from? from the alpha-Centauri television series(approx. 15 minutes). First broadcast on July 4, 1999.
- What are sunspots and solar storms? from the alpha-Centauri television series(approx. 15 minutes). First broadcast on July 18, 1999.
- Does the sun control our weather? from the alpha-Centauri television series(approx. 15 minutes). First broadcast on Nov 12, 2003.
- Is the sun special? from the alpha-Centauri television series(approx. 15 minutes). First broadcast on Sep 29 2004.
Individual evidence
- ^ NASA Sun Fact Sheet .
- ↑ Steadly RS, Robinson MS (ed.): The Astronomical Almanac for the Year 2012. US Government Printing Office, ISBN 978-0-7077-41215 , p. K7
- ↑ Luzum B. et al .: The IAU 2009 system of astronomical constants: the report of the IAU working group on numerical standards for Fundamental Astronomy. Celestial Mechanics and Dynamical Astronomy, Vol. 110, Issue 4 (August 2011), pp. 293–304, doi : 10.1007 / s10569-011-9352-4 , p. 296 (Heliocentric gravitational constant, TDB -compatible)
- ^ A b Katharina Lodders et al .: Abundances of the elements in the solar system. In: J. E. Trümper (Ed.): Landolt-Börnstein, New Series. Vol. VI / 4B. Springer, Hamburg 2009, 560–630. arxiv : 0901.1149 .
- ^ The dictionary of origin (= Der Duden in twelve volumes . Volume 7 ). 2nd Edition. Dudenverlag, Mannheim 1989, p. 681 . See also DWDS ( "Sonne" ) and Friedrich Kluge : Etymological dictionary of the German language . 7th edition. Trübner, Strasbourg 1910 ( p. 430 ).
- ↑ https://www.iau.org/public/themes/naming/
- ↑ https://earthsky.org/space/what-is-the-suns-name
- ↑ http://ourfiniteworld.com/2012/03/12/world-energy-consumption-since-1820-in-charts/ World energy consumption since 1820.
- ↑ Carole Stott, Robert Dinwiddie, David Hughes, Giles Sparrow: Space, Das Weltall. Dorling Kindersley Verlag, 2011, ISBN 978-3-8310-1972-4 . P. 209.
- ↑ William J. Chaplin, Sarbani Basu: Perspectives in Global Helioseismology and the Road Ahead. In: Laurent Gizon et al. (Ed.): Helioseismology, Asteroseismology, and MHD Connections. Springer 2008, ISBN 978-0-387-89481-2 . P. 53–75 limited preview in Google Book search
- ^ A. Bonanno, H. Schlattl, L. Patern: The age of the Sun and the relativistic corrections in the EOS . (PDF) In: Astronomy and Astrophysics . 390, 2002, pp. 1115-1118. arxiv : astro-ph / 0204331v2 .
- ^ CJ Hansen et al .: Stellar Interiors - Physical Principles, Structure, and Evolution. Springer, 2004, ISBN 0-387-20089-4 . P. 77 f.
- ^ Charles Darvin in a letter to Asa Gray , 1860, footnote 16.
- ^ William Thomson: On the Age of the Sun's Heat . In: en: Macmillan's Magazine . 5, 1862, pp. 388-393.
- ^ L. Darden: The Nature of Scientific Inquiry. 1998, accessed July 31, 2011 .
- ↑ Sean G. Ryan, Andrew J. Norton: Stellar Evolution and Nucleosynthesis. Cambridge University Press, 2010, ISBN 978-0-521-19609-3 , limited preview in Google Book Search.
- ↑ Hannu Karttunen, Pekka Kröger, Heikki Oja, Markku Poutanen, Karl Johan Donner: Fundamental Astronomy . 5th edition. Springer, Berlin / Heidelberg / New York 2007, ISBN 978-3-540-34143-7 , 10.3 Stellar Energy Sources, p. 235 (English, Finnish: Tähtitieteen perusteet . Helsinki 2003.).
- ^ Klaus Stierstadt: Thermodynamics: From microphysics to macrophysics. Springer, 2010, ISBN 978-3-642-05097-8 , limited preview in the Google book search.
- ^ The 8-minute travel time to Earth by sunlight hides a thousand-year journey that actually began in the core. NASA, accessed May 30, 2008 .
- ^ Michael Stix: On the time scale of energy transport in the sun. In: Solar Physics. Volume 212, 2002, pp. 3-6, doi : 10.1023 / A: 1022952621810 .
- ^ Subrahmanyan Chandrasekhar: Radiative Transfer and Negative Ion of Hydrogen. Chicago Univ. Press, 1989, ISBN 0-226-10092-8 (Vol. 2), limited preview in Google Book Search.
- ↑ KD Abhyankar: A Survey of the Solar Atmospheric Models. In: Bulletin of the Astronomical Society of India. Volume 5, 1977, pp. 40-44 ( PDF ).
- ↑ K. Muglach et al .: The Electron Temperature of the Solar Transition Region as Derived from EIS and SUMER , APJ 708, 2010, p. 550, doi : 10.1088 / 0004-637X / 708/1/550
- ↑ M. Aschwanden et al. (TRACE): Heating Coronal Loops (Astronomy Picture of the Day Sept. 28, 2000).
- ^ Mari Paz Miralles: The Sun, the Solar Wind, and the Heliosphere. Springer, 2011, ISBN 978-90-481-9786-6 , limited preview in the Google book search.
- ^ Wilcox Solar Observatory ( Stanford ): Carrington and Bartels Calendars .
- ↑ David Kuridze et al .: Mapping the magnetic field of flare coronal loops. February 20, 2019 on arxiv: 1902.07514 .
- ↑ DER SPIEGEL: Researchers are presenting more detailed images of the sun than ever before - DER SPIEGEL - Wissenschaft. Accessed January 31, 2020 .
- ↑ NSF's newest solar telescope produces first images- All Images | NSF - National Science Foundation. Accessed February 1, 2020 .
- ^ First Image from NSF's Inouye Solar Telescope in Context of the Full Sun. January 29, 2020, accessed February 1, 2020 (American English).
- ^ Perihelion and Aphelion - Astronomy Picture of the Day, July 3, 2009. Retrieved December 30, 2009.
- ↑ I. Ramirez et al .: Elemental Abundances of Solar Sibling Candidates . In: The Astrophysical Journal . August. arxiv : 1405.1723 . bibcode : 2014ApJ ... 787..154R . doi : 10.1088 / 0004-637X / 787/2/154 .
- ↑ Katharina Lodders: Solar System Abundances and Condensation Temperatures of the Elements . In: The Astrophysical Journal . tape 591 , 2, pages = 1220-1247, July 2003, doi : 10.1086 / 375492 , bibcode : 2003ApJ ... 591.1220L .
- ^ Martin Asplund et al .: The chemical composition of the Sun. In: Annual Reviews of Astronomy and Astrophysics. Volume 47, 2009. pp. 481-522. arxiv : 0909.0948v1 .
- ↑ Ch. Bounama, W. von Bloh, S. Franck: In search of a second earth, Physics in our time, 33rd year 2002, No. 3, pp. 122–128
- ^ Early History of Radio Astronomy nrao.edu; (accessed June 30, 2010).
- ↑ Solar Probe Plus ( Memento from April 18, 2016 in the Internet Archive )
- ↑ Solar Orbiter - Exploration of the Sun and the Heliosphere. DLR, accessed on December 18, 2018 .