Cochlea
The cochlea , even cochlea or cochlear ( Latin cochlea , snail ' ; borrowed from ancient Greek κοχλίας ), is part of the inner ear of mammals and represents the receptive field for the hearing perception is your vibration mechanical properties for their research. Georg von Békésy 1961 received the Nobel Prize , contribute to the resolution of different pitches ; likewise the different types of hair cells and their neural connections.
Structure of the cochlea
The cochlea is a snail-shaped cavity in the petrous bone with two and a half turns in humans and, for example, four turns in guinea pigs . It is surrounded by a bone material that is the hardest material in the human body after the teeth. In the context of a congenital malformation ( Mondini dysplasia ) that is associated with hearing loss or deafness , the snail can be reduced to one and a half turns.
The bony axis of the cochlea is called the worm spindle ( modiolus ). It is connected to the internal auditory canal and contains the trunk of the auditory part of the 8th cranial nerve ( vestibulocochlear nerve ).
Inside the cochlea is divided into three superimposed fluid-filled passages. your name is
- Scala vestibuli (vestibule stairs ; Latin scala 'stairs' , 'ladder'; Latin vestibulum 'forecourt' )
- Scala media or cochlear duct (snail duct ) and
- Scala tympani (timpani staircase).
The base of the cochlea is adjacent to the middle ear with the ossicles . The footplate of the stapes is movably fitted into the oval window ( fenestra vestibuli or fenestra ovalis ). Behind the oval window is the vestibule staircase ( scala vestibuli ). This goes at the tip of the snail (Latin apex ) over the snail hole ( Helicotrema ) into the tympanic staircase ( Scala tympani ), so both form a coherent channel. The latter borders on the base of the round window ( Fenestra cochleae ), which is closed to the middle ear by a freely oscillating membrane ( Membrana tympani secundaria ). A pressure of the ossicles on the oval window runs as a traveling wave over the scala vestibuli towards the tip of the cochlea and leads to the deflection of the basilar membrane , which transfers the imported pressure to the scala tympani . This pressure can be compensated through the round window.
The scala media is separated from the scala vestibuli by the Reissner membrane (after Ernst Reissner ) and from the scala tympani by the spiral bone sheet ( Lamina spiralis ossea ) and the basilar membrane ( Lamina basilaris or basilar membrane ) . The scala vestibuli and the scala tympani are filled with perilymph , which is exchanged between the two courses via the helicotrema. The scala media contains endolymph . Both liquids differ in their composition: the perilymph resembles the extracellular milieu, while the endolymph has a high potassium concentration. In this it resembles the cytosol .
From sound to nerve impulse
On the basilar membrane lies the organ of Corti with four rows of hair cells that have different properties:
- The outer hair cells (three rows) are used to amplify the sound waves within the cochlea (so-called cochlear amplifier ). They work as combined sensor and motor cells, whereby they amplify the self-registered sound signals through the motor function of the hair bundles and additionally through the motor function of the cell bodies (sound-synchronous changes in length) and thereby increase the frequency selectivity. This selective reinforcement is passed on to the neighboring inner hair cells.
- The inner hair cells (a row) convert mechanical vibrations into nerve impulses (called transduction ) that are passed on to the brain.
The conversion of sound into nerve impulses essentially depends on the following influences:
- Electrical and mechanical vibration properties of cell bodies and hair bundles of the outer hair cells with constant frequency-specific gradients along the cochlea
- Conversion of the mechanical stimulation of the inner hair cells into nerve impulses
Vibration-mechanical properties of the inner ear
When the sound enters the inner ear, it creates a wave there that travels through the inner ear. One speaks of the traveling wave . It uses the tectorial membrane to deflect the sensory hairs ( stereocilia ) of the outer hair cells, but not those of the inner hair cells (in contrast to the outer ones, these have no contact with the tectorial membrane).
The basilar membrane and cochlea act as a mechanical resonator system. Since the width of the basilar membrane increases from the oval window to the helicotrema at the expense of the lamina spiralis ossea , but the diameter of the bony cochlear canal decreases, the mechanical properties (mass coating, stiffness, damping) and thus also the vibration properties of the system change depending on the distance to the Helicotrema. Near the oval window, the basilar membrane is stiff and therefore resonant with high frequencies; near the helicotrema, it is compliant and resonant with low frequencies. Conversely, due to its inertia , the liquid in the screw channel is stiff for high frequencies and increasingly flexible for low frequencies. As the frequency decreases, waves can penetrate deeper and deeper into the screw channel. Before a wave with a certain frequency reaches the place where it is resonant, it does not cause any great inertial forces, but rather its energy is transported further into the interior hydraulically , through opposing longitudinal movements of the two columns of liquid. The fact that behind the point of resonance the membrane is even more flexible because it is wider and the column of liquid stiffer because it is narrower, so that the wave hardly spreads any further (a short circuit in the sense of the line theory - the lowest frequencies are caused by the helicotrema) contributes to the spectral selectivity shorted to avoid damage).
The outer hair cells
The outer hair cells already react to a slight deflection of their hair bundles with motor activity of these hair bundles and additionally with an active change in length of their entire cell body. The outer hair cells are able to do this through a special membrane protein , prestin . This is a contractile protein in the plasma membrane that shortens or elongates depending on the potential . Transgenic mice lacking the gene for prestin have a greatly reduced hearing sensitivity . This is considered evidence that the prestin motors in the cell membrane of the outer hair cells amplify the sound within the inner ear and increase frequency selectivity.
The outer hair cells influence the mechanical vibrations of the basilar membrane-cochlear canal system. At the point of resonance, the vibrations are amplified and the inner hair cells are stimulated more. Beyond the resonance point, the vibrations are strongly dampened, the corresponding frequency hardly spreads any further. This increases the frequency selectivity of the inner ear and makes it easier to break down sounds or human speech into individual tone frequencies (“cochlear amplifier”).
Another effect is that high frequencies, which have their resonance point near the oval window, do not stimulate the inner hair cells for low frequencies. Low frequencies, which only cause a maximum of excitation in the vicinity of the helicotrem, on the other hand also excite the hair cells responsible for high frequencies.
The inner hair cells
The individual frequencies of a sound decomposed in this way stimulate the inner hair cells that specialize in the respective frequencies. The stimulus triggers an electrical signal in the hair cells (mechano-electrical transduction). These send a chemical signal ( transmitter glutamate ) to an auditory nerve fiber (transformation), whereby each auditory nerve fiber passes on the frequency selection of its connected inner hair cell. The auditory nerve fibers react electrically ( action potential ) and extend to the first core area of the auditory pathway in the brain stem . In this way, the sound frequencies are separated and sent electrically to the brain.
The excitation of a hair cell depends on its history. If a mechanical stimulus occurs after a certain period of rest, the hair cell “fires” particularly intensely. If the stimulation persists for a certain time, the number of nerve impulses decreases (so-called adaptation ). The original high number of nerve impulses is only reached again after a certain time with little excitation. This fact is simulated, among other things, in psychoacoustic models with digital signal processors , which are used for audio data compression in sound recording.


Innervation of the hair cells
The hair cells are supplied by afferent and efferent nerve fibers. While the afferent fibers come from the spiral ganglion , the efferent fibers come from the upper olive pits (via the olivocochlear tract or Rasmussen's bundle).
The spiral ganglion consists of over 30,000 bipolar nerve cells . More than 90% of these are myelinated neurons (type I), which are in contact with the inner hair cells. The smaller, unmyelinated neurons (type II) supply the outer hair cells. Both types send impulses to the cochlear nuclei in the medulla oblongata .
The efferent fibers first run with the vestibular nerve into the internal auditory canal , but then branch off via the Oort anastomosis to the cochlear part of the vestibulocochlear nerve . The physiological function consists in influencing (modulating) the afferent connections ( synapses ) of the inner hair cells and the motor activity of the outer hair cells.
The inner hair cells are supplied by the radial afferent fibers and the lateral efferent fibers, the outer hair cells by the spiral afferent and the medial efferent fibers.
Inner hair cells: All type I nerve cells originating from the spiral ganglion are only connected to synapses with the inner hair cells. Their dendrites form the radial afferent system. The axons collect in the modiolus and run with the cochlear nerve to the cochlear nuclei. Each inner hair cell is in contact with about ten afferent fibers. However, this number is significantly higher in the area of best hearing.
Part of the efferent fibers (lateral efferent system) comes into contact with the synapse heads of the afferent fibers on the inner hair cells and forms synapses with them. So these fibers have no direct contact with hair cells.
Outer hair cells: The outer hair cells only have a comparatively low supply of afferent fibers. Only in the last (apical) turn is there a higher supply of afferent fibers. The outer hair cells only form synapses to the unmyelinated type II fibers of the spiral ganglion. These fibers run from the outer hair cells towards the modiolus at the bottom of the Corti tunnel. They accompany the outer hair cells in a spiral (spiral afferent system), and each individual fiber has synaptic contact with several cells.
The medial efferent fibers run freely through the tunnel of Corti as radial tunnel fibers and form synapses with the lower part of the cell body of the outer hair cells (remote from the hair bundle). Here, too, a fiber has synaptic contact with several cells.
Influences on acoustic perception
The way in which sound signals are converted into nerve impulses and where nerve impulses arise in the inner ear influence acoustic perception.
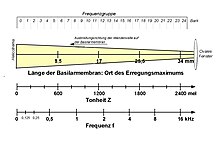
pitch
The pitch that is perceived when sounding at a certain frequency is closely related to the location on the basilar membrane at which there is a maximum of excitation at this frequency. Since the basilar membrane on the oval window is narrow and thick, its natural frequency is high here (with low amplitude). Further towards the Helicotrema, where it is wider and thinner, it vibrates at a lower frequency (with greater amplitude).
In animal experiments it was possible to determine the location on the basilar membrane at which a maximum excitation of the inner hair cells is achieved for a certain frequency. The location of the maximum excitation in humans was derived from this on the basis of physiological comparisons. With the help of listening tests it can in turn be determined which frequency leads to which pitch sensation. A linear relationship was found between the position of the maximum excitation on the basilar membrane (calculated as the distance from the helicotrema) and the perceived pitch.
The audible range is roughly from 20 Hz to 20 kHz, the hearing threshold being different for different frequencies and being lowest at 2–4 kHz; biographically, one hears the high frequencies more and more worse ( presbycusis ). Location principle : If the basilar membrane is excited with a certain frequency, it will vibrate most where it can vibrate best with this frequency. Only a few inner hair cells are responsible for a frequency (which also have a particularly low stimulus threshold for this frequency); Due to the interconnection in the auditory pathway , certain neurons in the primary auditory cortex are only responsible for certain frequencies ( tonotopy ). In addition to this passive component, this vibration maximum of the basilar membrane is also sharply outlined because the outer hair cells are excited at the location of the vibration maximum and amplify the vibration about a thousand times through contraction ( cochlear amplifier ), so that the inner hair cells are only in a very small area be very excited. In addition to this “mechanically active” component, there is also a neural: lateral inhibition along the auditory pathway (especially in the spirale cochleae ganglion), ie strongly excited neurons inhibit neighboring slightly excited neurons (which would transmit the directly neighboring frequency range). This contrasting is used for noise suppression.
Volume and sound
The total number of nerve impulses generated per frequency band is a measure of the perceived loudness of a sound signal. The number of nerve impulses emitted depends on the strength of the excitation of the inner hair cells and thus on the vibrational behavior of the basilar membrane.
The pattern of excitation caused by a particular tone can be traced through masking experiments. If a second, quieter tone can no longer be heard when a tone is present, this indicates that the first tone has already excited the nerve cells that are responsible for perceiving the second tone much more than the second tone can.
Due to the vibration behavior of the basilar membrane, individual tones also stimulate nerve cells that are above their frequency, i.e. belong to frequencies that are not included in the sound signal. In the case of sound signals with a flat frequency response , no nerve cells are excited outside the frequency response of the sound signal. This means that individual tones (or sound signals with strong tonal components) are perceived as louder than broadband sound signals with the same sound level .
On the other hand, the timing of a sound signal influences the number of nerve impulses emitted. If a sound signal sets in (in a frequency range) after a long period of rest, the nerve cells fire particularly strongly. If the sound persists for a long time, the number of nerve impulses drops back to an average value.
As a result, sound signals with sudden sound inserts (e.g. hammering) are perceived as significantly louder than uniform sound signals with the same sound level .
Just like the volume, the perceived sound is also influenced by this, tonal components and sound inserts determine the sound impression much more than the physical spectrum of a sound signal would suggest.
Signal processing of the hearing
The position of the maximum excitation on the basilar membrane not only determines the perceived pitch (see above), but also which signal components are jointly evaluated by the ear .
To do this, the brain divides the audible frequency spectrum into sections, so-called frequency groups . The nerve impulses from a frequency group are evaluated together in order to determine the volume , sound and direction of the sound signal in this frequency range.
The width of a frequency group is approx. 100 Hz for frequencies up to 500 Hz and a minor third above 500 Hz. (This corresponds to approx. 1 bark or 100 mel )
The healthy person can normally perceive frequencies of 20-18000 Hz. The frequency range decreases with age.
Technical application of frequency group effects
The frequency group effects are used in data reduction processes such as MP3 .
Here, as with the hearing, signal ranges are analyzed in frequency groups. Signal areas that cannot be heard due to masking effects (i.e. due to lateral inhibition in the brain) are removed from the signal or transmitted with a lower quality. This reduces the amount of data, but a difference to the original signal is not perceptible to humans.
The healthy ear can distinguish between frequencies at 1 kHz of approximately ± 3 Hz. If there is a hearing impairment, the ability to distinguish between frequencies may be reduced depending on the type and extent of the hearing impairment.
See also
literature
- Martin Trepel: Neuroanatomy. Structure and function. StudentConsult . 3. Edition. Urban & Fischer bei Elsevier, 2006, ISBN 3-437-44425-5 .
- MC Liberman, J. Gao, DZ He, X. Wu, S. Jia, J. Zuo: Prestin is required for electromotility of the outer hair cell and for the cochlear amplifier. In: Nature . tape 419 , no. 6904 , 2002, pp. 300–304 , doi : 10.1038 / nature01059 .
Single receipts
- ^ Anne M. Gilroy, Brian R. MacPherson, Lawrence M. Ross: Atlas of Anatomy. Thieme, 2008, p. 536, ISBN 978-1-60406-151-2