Mars Science Laboratory
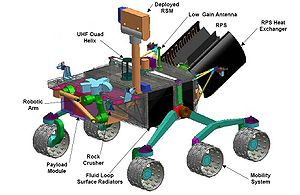
The Mars Science Laboratory (MSL) is a NASA rover scheduled to be launched on September 15, 2009[1] and perform the first ever precision landing on Mars in July-September of 2010. This rover will be three times as heavy and twice the width of the Mars Exploration Rovers (MERs) that landed in 2004. It will carry more advanced scientific instruments than any other mission to Mars to date, including analysis of samples scooped up from the soil and drilled powders from rocks. It will also investigate the past or present ability of Mars to support life. The international community will provide some of the instruments onboard.
The MSL rover will be launched by an Atlas V 541. MSL will be expected to operate for at least 1 martian year (668 Martian sols/686 Earth days) as it explores with greater range than any previous Mars rover.
History
In September 2006, MSL was approved by NASA HQ for a 2009 launch. Several JPL engineers working on MSL have informally stated that the MSL design will likely be used on future rovers after the first MSL is launched in 2009.
In April 2008, USA Today reported that the project is $235 million, or 24%, over budget. The money to compensate this overrun should come from other NASA Mars missions that will need to be cut.[2] In August 2008, it was announced that the third MSL workshop would be held to summarize the data on the 6 potential landing sites.[3]

Specifications

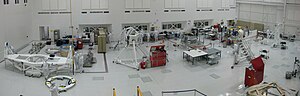
MSL is expected to weigh over 800 kg (1,760 lb) including 65 kg (143 lb) of scientific instruments, compared to the Mars Exploration Rovers which weigh 185 kg (407 lb) including 5 kg (11 lb) of scientific instruments. A YouTube video shows a MSL mockup compared to the Mars Exploration Rover and Sojourner Rover.[4] MSL will be able to roll over obstacles approaching 75 cm (29 in) in height. Maximum terrain-traverse speed is estimated to be 90 m (295 ft) per hour via automatic navigation, however, average traverse speeds will likely be about 30 m/h (98 ft/h), based on variables including power levels, difficulty of the terrain, slippage, and visibility. MSL is expected to traverse a minimum of 6 km (3.7 mi) in its two-year mission.
Proposed scientific payload
At present 10 instruments have been selected for development or production for MSL:
Cameras (MastCam, MAHLI, MARDI)
All cameras are being developed by Malin Space Science Systems; all share common design components such as on-board electronic imaging processing boxes and 1600x1200 color CCDs.
- MastCam: This system will provide multiple spectra and true color imaging with two-camera stereoscopic (three-dimensional) vision. True-color are at 1200x1200 pixels and up to 10 frames per second hardware-compressed, high-definition video at 1280x720. For comparison the Mars Exploration Rover (MER) panoramic cameras can only produce 1024x1024 black&white images. The same filter wheel design for multiple spectra images from MER will be used on MastCam. Both cameras will have mechanical zoom and can image objects as far away as 1 km at a resolution of 10 cm per pixel.
- Mars Hand Lens Imager (MAHLI): This system will consist of a camera mounted to a robotic arm on the rover. It will be used to acquire microscopic images of rock and soil, like the microscopic imager (MI) on MER. Unlike the MI, MAHLI will take true color images at 1600x1200 pixels with a resolution as high as 12.5 micrometers per pixel. MAHLI will have both white and UV LED illumination for imaging in darkness or imaging fluorescence. MAHLI will also have mechanical focusing in a range from infinite to mm distances.
- MSL Mars Descent Imager (MARDI): During the descent to the Martian surface MARDI will take approximately 500 color images at 1600x1200 pixels starting at distances of about 3.7 km to near 5 meters from the ground. MARDI imaging will allow the mapping of surrounding terrain and location of landing. On September 16, 2007 NASA announced that MARDI would not be included in the MSL mission due to funding problems.[5] MARDI was subsequently reinstated after the contractor, Malin Space Science Systems, agreed that there would be no additional costs to NASA for its completion.[6] MARDI will take images at a rate of 5 frames per second for about 2 minutes during descent and landing.[7]
ChemCam
ChemCam is a remote Laser-induced breakdown spectroscopy (LIBS) system that can target a rock from up to 13 meters away, vaporizing a small amount of the underlying mineral and then collecting a spectrum of the light emitted by the vaporized rock by using a micro-imaging camera with an angular resolution of 80 microradians. It is being developed by the Los Alamos National Laboratory and the French CESR laboratory (in charge of the laser). A infrared laser with 1067 nm wavelength and a 5 ns pulse will focus on a spot with 1 GW/cm², depositing 30 mJ of energy. Detection will be done between 240 nm and 800 nm.[8] [9] [10] In October 2007 NASA announced that they would cap funding for the ChemCam because of a 70% cost overrun and that the instrument has to be built with the money already provided.[11] Since most of the money for ChemCam came from the French CNES, The Los Alamos National Laboratory was able to deliver the engineering model to JPL in February 2008.[12]
Alpha-particle X-ray spectrometer (APXS)
This device will irradiate samples with alpha particles and map the spectra of X-rays that are reemitted. It is being developed by the Canadian Space Agency for determining the elemental composition of samples. The APXS is a form of PIXE. Similar instruments have been part of Mars Pathfinder and the Mars Exploration Rovers.[13]
CheMin
Chemin stands for "Chemistry & Mineralogy X-Ray Diffraction/X-Ray Fluorescence Instrument". Chemin is a X-ray diffraction/X-ray fluorescence instrument that will quantify minerals and mineral structure of samples. It is being developed by Dr. David Blake at NASA Ames Research Center and the NASA's Jet Propulsion Laboratory.[14]
Sample Analysis at Mars (SAM)
The SAM instrument suite will analyze organics and gases from both atmospheric and solid samples. It is being developed by the NASA Goddard Space Flight Center and Laboratoire Inter-Universitaire des Systèmes Atmosphériques (LISA).[15]
SAM Consists of the 74 sample cups of the Sample Manipulation System[16] which can be heated to a temperature of 1000°C and the Chemical Separation and Processing Lab for enrichment and derivatization of the organic molecules of the sample. The gas chromatograph mass spectrometer is a quadrupole mass spectrometer with a mass range of 2–235 u which gets the sample from one of the six gas chromatography columns. The Tunable Laser Spectrometer is capable of measuring the isotope ratios of carbon and oxygen in CO2, and carbon in CH4. [17]
Radiation Assessment Detector (RAD)
This instrument will characterize the broad spectrum of radiation found near the surface of Mars for purposes of determining the viability and shielding needs for human explorers. Funded by the Exploration Systems Mission Directorate at NASA Headquarters and developed by Southwest Research Institute (SwRI) and the extraterrestrial physics group at Christian-Albrechts-Universität zu Kiel, Germany.
Dynamic Albedo of Neutrons (DAN)
A pulsed neutron source and detector for measuring hydrogen or ice and water at or near the martian surface, provided by the Russian Federal Space Agency.
Rover Environmental Monitoring Station (REMS)
Meteorological package and an ultraviolet sensor provided by the Spanish Ministry of Education and Science. It will be mounted on the camera mast and measure atmospheric pressure, humidity, wind currents and direction, air and ground temperature and ultraviolet radiation levels.
MSL Entry Descent and Landing Instrumentation (MEDLI)
The MEDLI project’s main objective is to measure aerothermal environments, sub-surface heat shield material response, vehicle orientation, and atmospheric density for the atmospheric entry through the sensible atmosphere down to heatshield separation of the Mars Science Laboratory (MSL) entry vehicle. The MEDLI instrumentation suite will be installed in the heatshield of the MSL entry vehicle. The acquired data will support future Mars missions by providing measured atmospheric data to validate Mars atmosphere models and clarify the design margins on future Mars missions. MEDLI instrumentation consists of three main subsystems: MEDLI Integrated Sensor Plugs (MISP), Mars Entry Atmospheric Data System (MEADS), and the Sensor Support Electronics (SSE).
Engineering cameras
The rover will have the same type of engineering cameras that the MER rovers had: a stereo pair of Navcams used to select safe routes for driving and a set of front and rear stereo-pair Hazcams used for autonomous hazard avoidance during rover drives and for safe positioning of the robotic arm on rocks and soils.
Power source

The rover will be powered by radioisotope thermoelectric generators (RTGs). Solar power is not an efficient power source for Mars surface operations. Solar power systems cannot operate effectively at high Martian latitudes, in shaded areas, nor in dusty conditions. This limitation was emphasized by the July 2007 dust storms that nearly resulted in the permanent failure of the Mars Exploration Rovers. Furthermore, solar power cannot provide power at night, thus limiting the ability of the rover to keep its systems warm, reducing the life expectancy of electronics. RTGs can provide reliable, continuous power day and night, and waste heat can be used via pipes to warm systems, freeing electrical power for the operation of the vehicle and instruments.
The first successful Mars landers, Viking 1 and Viking 2 in 1976, were RTG-powered—the Viking 1 lander worked for six years on the Martian surface (ultimately failing due to faulty command sent by ground control that resulted in loss of contact). The proposed power plant will use "next generation" RTGs, either Boeing’s Multi-mission Radioisotope Thermoelectric Generator, which is a more flexible and compact power system under development and based on conventional RTGs, or Lockheed Martin’s Stirling Radioisotope Generator, which is more efficient but untested for use in space. Evidence points to the MMRTG being selected at this point, likely because of reliability and underdevelopment issues with the SRG.
Landing system
MSL will be set down on the Martian surface using a new NASA high-precision entry, descent, and landing (EDL) system that will place it within ten kilometers of an intended target, in contrast to the 150-kilometer error of previous landing systems used on Mars. The rover is folded up within an aeroshell which protects it during the travel through space and during the atmospheric entry at Mars. Much of the reduction of the landing precision error is accomplished by an entry guidance algorithm, similar to that used by the astronauts returning to Earth in the Apollo space program. This guidance uses the lifting force experienced by the aeroshell to "fly out" any detected error in range and thereby arrive at the targeted landing site. In order for the aeroshell to have lift, its center of mass is offset from the axial centerline which results in an off-center trim angle in atmospheric flight, again similar to the Apollo Command Module. This is accomplished by a series of ejectable ballast masses. The lift vector is controlled by four sets of two Reaction Control System (RCS) thrusters that produce approximately 500 N of thrust per pair. This ability to change the pointing of the direction of lift allows the spacecraft to react to the ambient environment, and steer toward the landing zone.
After the entry phase is complete and the capsule has slowed to Mach 2 several kilometers over the ground, a supersonic parachute is deployed. The parachute is similar in design to that used by the Viking landers, Mars Pathfinder, and the Mars Exploration Rovers. The entry vehicle must first eject the ballast mass such that the center of gravity offset is removed and the velocity vector aligns with the parachute deployment vector. This prevents the spacecraft from experiencing a torque due to the pointing differences and increases the spacecraft's stability.
Following the parachute deployment, the rover and descent stage drop out of the aeroshell. A number of passive mechanisms are used to ensure a clean separation. The descent stage is a platform above the rover with variable thrust mono propellant hydrazine rocket thrusters (based on upgraded Viking heritage landing rockets) on arms extending around this platform to slow the descent. Meanwhile the rover itself is being transformed from its stowed flight configuration to a landing configuration while being lowered beneath the descent stage by the "sky crane" system. The descent stage contains the Bridle Umbilical Descent Rate Limiter (BUDrl) which is a next generation design based on MER heritage. This consists of 3 bridles lowering the rover itself and an umbilical cable carrying electrical signals between the descent stage and rover. At roughly 7.5 meters below the descent stage the sky crane system slows to a halt and the rover touches down.
After the rover touches down it waits 2 seconds to confirm that it is on solid ground and fires several pyros (small explosive devices) activating cable cutters on the bridle and umbilical cords to free itself from the descent stage. The descent stage promptly flies away to a crash landing, and the rover gets ready to roam Mars.
Early landing system proposals for the rover featured a "pallet" design (similar to that used for Mars Pathfinder and the Mars Exploration Rovers) with a liquid-fueled rocket thruster system to land the rover in the final seconds of descent. The pallet would have used airbags or a "crushable" design to permit a safe landing. Earlier designs also sometimes included a second parachute for subsonic speeds. The planned "skycrane" powered descent landing system (as it has been referred) has never been used in actual missions before.
Proposed landing sites
At the first MSL Landing Site workshop, 33 potential landing sites were identified.[18] The current engineering constraints call for a landing site less than 45° from the Martian equator and less than 1 km above the reference datum.[19]
Name | Location | Elevation | Target |
---|---|---|---|
Nili Fossae Trough | ≈22°N, ≈75°E | −0.6 km | Phyllosilicates |
Holden Crater Fan | 26.4°S, 325.3°E | −2.3 km | Layered Materials |
Terby Crater | 28°S, 73°E | −5 km | Layered Material |
Mawrth Vallis | 22.3°N, 343.5°E | ≈−2 km | Phyllosilicates |
Eberswalde Crater | 24.0°S, 326.3°E | −0.8 and −0.4 km | Delta |
Gale Crater | 4.6°S, 137.2°E | −4.5 km | Interior Layered Deposits |
Candor Chasma | Various | −4 km | Sulfate Deposits |
North Meridiani Planum | 2.7°N, 358.8°E | −1.5 km | Sedimentary Layers |
Juventae Chasma | 5°S, 297°E | −2 km | Layered Sulfates |
Nilo Syrtis | ≈23°N, ≈76°E | ≈−0.5 km | Phyllosilicates |
Melas Chasma | 9.8°S, 283.6°E | −1.9 km | Paleolake |
East Meridiani Planum | 0°, 3.7°E | ≈−1.3 km | Sedimentary Layers |
Athabasca Vallis | 10°N, ?°E | −2.4 km | Cerberus Rupes Deposits |
Iani Chaos | 2°S , ≈342°E | Below −2 km | Hematite, Sulfate |
Crater in Nili Fossae | 18.4°N, 77.68°E | −2.6 km | Valley Networks, layers |
Eos Chasma | ≈11°N, ≈320°E | ≈−4 km | Chert |
Crater lake in Meridiani Planum | 5.6°N, 358°E | ≈−1.5 km | Crater lake sediments |
NE Syrtis Major | ≈10°N, ≈70°E | ≈1 km | Volcanics |
Basin in Margaritifer Terra | 12.77°S, 338.1°E | −2.12 km | Fluvial Deposits |
Eastern Melas Chasma | 11.62°S, 290.45°E | Below −2 km | Interior Layered Deposits |
Hellas Planitia/Dao Vallis | 40°S, 85°E | Below −2 km | A major valley |
Xanthe Terra/Hypanis Vallis | 11°N, 314°E | Below −2 km | Delta |
Becquerel Crater | 21.8°N, 351°E | −2.6 to −3.8 km | Layered Sedimentary Rocks |
SW Arabia Terra | 2–12°N, 355–348°E | −1 km | Sed. Rocks, Methane |
Gullies/Hale Crater | 35.7°S, 323.4°E | −2.4 km | Gullies |
W. Arabia Terra | 8.9°N, 358.8°E | −1.2 km | Sedimentary Rocks |
Argyre Planitia | 56.8°S, 317.7°E | −1.5 km | Glacial Features |
NW Slope Valleys | ≈0, 145°E | ≈−2 km | Flood Features |
Western Meridiani Planum | 1.8°S, 7.6°E | ≈−1.0 to −1.5 km | Sediments, Hematite |
Elysium Planitia/Avernus Colles | 1.0°S, 169.5°E | Below −2 km | High iron abundance |
Meridiani Bench | 7.5°N, 354°E | ≈−1 to −1.5 km | Layered Sediments |
SML Craters | 49°S, 14°E | Above −0.5 km | Recent Climate Deposits |
Isidis Planitia Escarpment | 5–15°N, 80–95°E | Below −2 km | Volatile sink |
By the second workshop in late 2007, the list had grown to include almost 50 sites. These sites were presented in more detail at the Second MSL Landing Site Workshop,[20] and the list was reduced to six based on the votes of the members of the science community that were present as well as the votes of the science working group (the PI's of the instruments aboard MSL).[21] One of the sites, Miyamoto crater (on the southwest corner of Meridiani Planum), was not on the first workshop list and was identified as a desirable landing site on the basis of mineralogical data acquired with CRISM in the time between workshops. Also, the locations of some of the targets have been refined relative to the first list.
Name | Location |
---|---|
Miyamoto Crater | 2.9°S, 7°W |
Nili Fossae Trough | 21°N, 74°E |
Holden Crater Fan | 26.4°S, 325.3°E |
North Meridiani Planum | 2°N, 357°E |
Mawrth Vallis | 24°N, 341°E |
Eberswalde Crater Delta | 24°S, 327°E |
At the third workshop in September 2008, the Gale Crater, a landing site proposed for the first workshop, were revived as a contender because of new data. The workshop voted to rank the landing sites as follows. A final workshop in April 2009 is to select a single top choice from the list below.
Name | Location |
---|---|
Eberswalde Crater Delta | 24°S, 327°E |
Holden Crater Fan | 26.4°S, 325.3°E |
Gale Crater | ?°S, ?°E |
Nili Fossae Trough | 21°N, 74°E |
Mawrth Vallis | 24°N, 341°E |
Miyamoto Crater | 2.9°S, 7°W |
North Meridiani Planum | 2°N, 357°E |
References
- ^ NASA's Shuttle and Rocket Missions
- ^ "Troubles parallel ambitions in NASA Mars project".
- ^ "Mars Exploration Science Monthly Newsletter" (PDF). August 1, 2008.
{{cite web}}
: Check date values in:|date=
(help) - ^ "Mars Rovers". YouTube. 2008-04-12. Retrieved 2008-09-12.
- ^ "NASA Memo to Space Science Community: Mars Science Laboratory Project Changes Respond to Cost Increases, Keep Mars Program On Track". SpaceRef Interactive.
- ^ "Mars Science Laboratory Instrumentation Announcement from Alan Stern and Jim Green, NASA Headquarters". SpaceRef Interactive.
- ^ "Mars Descent Imager (MARDI) Update". Malin Space Science Systems. November 12, 2007.
{{cite web}}
: Check date values in:|date=
(help) - ^
Salle B., Lacour J. L., Mauchien P., Fichet P., Maurice S., Manhes G. (2006). "Comparative study of different methodologies for quantitative rock analysis by Laser-Induced Breakdown Spectroscopy in a simulated Martian atmosphere" (PDF). Spectrochimica Acta Part B-Atomic Spectroscopy. 61 (3): 301–313. doi:10.1016/j.sab.2006.02.003.
{{cite journal}}
: CS1 maint: multiple names: authors list (link) - ^ CESR presentation on the LIBS
- ^ ChemCam fact sheet
- ^ NASA Caps Funding for Mars Rover Sensor
- ^ ChemCam Status April, 2008
- ^ R. Rieder, R. Gellert, J. Brückner, G. Klingelhöfer, G. Dreibus, A. Yen, S. W. Squyres (2003). "The new Athena alpha particle X-ray spectrometer for the Mars Exploration Rovers". J. Geophysical Research. 108: 8066. doi:10.1029/2003JE002150.
{{cite journal}}
: CS1 maint: multiple names: authors list (link) - ^ Sarrazin P., Blake D., Feldman S., Chipera S., Vaniman D., Bish D. (2005). "Field deployment of a portable X-ray diffraction/X-ray flourescence instrument on Mars analog terrain". Powder Diffraction. 20 (2): 128–133. doi:10.1154/1.1913719.
{{cite journal}}
: CS1 maint: multiple names: authors list (link) - ^ Cabane M., Coll P., Szopa C., Israel G., Raulin F., Sternberg R., Mahaffy P., Person A., Rodier C., Navarro-Gonzalez R., Niemann H., Harpold D., Brinckerhoff W. (2004). "Did life exist on Mars? Search for organic and inorganic signatures, one of the goals for "SAM" (sample analysis at Mars)". Source: Mercury, Mars and Saturn Advances in Space Research. 33 (12): 2240–2245.
{{cite journal}}
: CS1 maint: multiple names: authors list (link) - ^ Tom Kennedy and Erik Mumm, Tom Myrick , Seth Frader-Thompson. "OPTIMIZATION OF A MARS SAMPLE MANIPULATION SYSTEM THROUGH CONCENTRATED FUNCTIONALITY" (PDF).
{{cite web}}
: CS1 maint: multiple names: authors list (link) - ^ Tarsitano, C.G. and Webster, C.R. (2007). "Multilaser Herriott cell for planetary tunable laser spectrometers". Applied Optics,. 46 (28): 6923–6935.
{{cite journal}}
: CS1 maint: extra punctuation (link) CS1 maint: multiple names: authors list (link) - ^ "MSL Landing Site Selection User's Guide to Engineering Constraints" (pdf). 2006-06-12. Retrieved 2007-05-29.
{{cite web}}
: Check date values in:|date=
(help) - ^ "MSL Workshop Summary" (pdf). 2007-04-27. Retrieved 2007-05-29.
{{cite web}}
: Check date values in:|date=
(help) - ^ "Second MSL Landing Site Workshop".
- ^ GuyMac. "Reconnaissance of MSL Sites". HiBlog. Retrieved 2008-01-12.
{{cite web}}
: Text "date=2008-01-04" ignored (help)
Further reading
M. K. Lockwood (2006). "Introduction: Mars Science Laboratory: The Next Generation of Mars Landers And The Following 13 articles " (PDF). Journal of Spacecraft and Rockets. 43 (2): 257–257. doi:10.2514/1.20678.
External links

- MSL Home Page
- Mars Science Laboratory Mission Profile by NASA's Solar System Exploration
- Malin Space Science Systems
- JPL's Mars Technology Program site
- Mars Science Laboratory Acquisition Program
- Spacecraft: Surface Operations Configuration: Science Instruments: ChemCam
- Overview of the SAM instrument suite
- Short description of EDL system (PDF)
- Next on Mars (Bruce Moomaw, Space Daily, March 9, 2005): An extensive overview of NASA's Mars exploration plans, with many details on the Mars Science Laboratory
- nuclearspace.com overview of MSL
- MSL EDL Video located on YouTube
- Demo of mobility system reported by Planetary Society.