Geostationary satellite
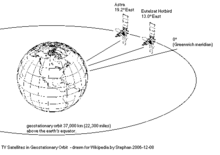

A geostationary satellite is an artificial earth satellite that is located in a circular orbit 35,786 km above the earth's surface above the equator . There is the geostationary orbit (English: Geostationary Earth Orbit , GEO for short), d. H. Satellites stationed there move at an angular velocity of one orbit around the earth per day and follow the rotation of the earth at an airspeed of about 3.07 km / s. As a result, geostationary satellites are ideally always above the same point on the earth's surface or the equator.
Typical examples of geostationary satellites are communication satellites , television satellites , and weather satellites .
A radio signal sent from the earth, which a geostationary satellite forwards back to a receiver on earth, experiences due to the latitude-dependent distance of approx. 35,800 to 41,700 kilometers, which must be traversed twice, and the speed of light , which is also used for radio waves holds, a delay ( latency ) of approximately 0.24 to 0.28 seconds. In the case of digital transmission, the use of data compression , encryption or data encoding also increases the delay times.
Types
There are two different types of geostationary satellites.
Spin stabilized satellites

Spin stabilized satellites were first developed. They are drum-shaped and their outer surface is covered with solar cells. The spin axis is parallel to the rotation vector of the satellite orbit and therefore always perpendicular to the current orbital velocity vector and because of the equatorial orbit also in north-south direction, i.e. parallel to the earth's axis.
If the satellite is not crossing the Earth's shadow, the spin aligns the solar cells arranged next to one another along the circumference one after the other towards the sun, with one half of the cells being completely shaded at any point in time and the other half being illuminated at more or less favorable angles . Communication satellites of this type usually have on their (northern) top side an untwisted antenna module with directional antenna (s) fixed to certain areas of the earth. The nozzle of the apogee motor protrudes from the (southern) underside of the satellite.
Spin or three-axis stabilized satellites

Spin or three-axis stabilized satellites usually have a cuboid main body. The front of the main body faces the earth. The back, on the other hand, points to the zenith and the apogee motor protrudes from it. The sides facing north and south carry the solar cell wings that track the sun while the satellite orbits the earth.
The front side carries the instruments, which are aligned with the earth - or, in the case of some communications satellites, an antenna module with (sometimes fold-out) directional antenna (s). These surround the base of a tower that carries the horn antennae that are in the focal points of the directional antenna (s). In other communications satellites, the horn antennas are mounted on the upper outer edge of the main body and irradiate very large directional antennas that are too large to be mounted on the top. They are attached to the sides that are still free to the east and west and are unfolded after take-off. There are also satellites that have antennas on both the top and the west and east sides.
A shot
Typically, the launcher places the satellite on a highly elliptical geostationary transfer orbit (GTO). From there, the satellite's own apogee motor propels it into geostationary orbit (GEO), usually with an electric drive . The satellite consumes most of its fuel or support mass supply (mostly xenon , with chemical propulsion nitrogen tetroxide and monomethylhydrazine ), so that after arriving at the GEO it has only about half as much mass as when it was launched. The remaining fuel reserves are then sufficient to carry out all orbit corrections during the lifetime of the satellite in the GEO.
The fact that the launcher drops the satellite directly in the GEO only occurs with Russian satellites and US military satellites. Consideration is given to introducing a new Ariane 5 re-ignitable upper stage that could also bring satellites directly into the GEO.
The costs for transporting payloads in the GTO are between 30,000 and 50,000 euros / kg , for those in the GEO between 300,000 and 400,000 euros / kg
Satellite orbit
The influence of the moon, the sun and especially the earth deformations disrupt the geostationary orbit. A satellite holds its position in only four positions, and only two of them are stable: 105.3 ° W and 75.1 ° E. The other two, 11.5 ° W and 161.9 ° E, are unstable. Small disturbances cause a drift to the stable position. Positioning a satellite outside these points therefore requires continuous orbit corrections. Satellites that get out of control and can no longer be transferred to a cemetery orbit will cluster at these two points. Currently (2010) there should be more than 160.
The web disturbances also influence the web inclination. Without corrections, it increases by around 0.5 ° per year, depending on the position. The satellite is no longer stationary in the sky, but moves relative to the earth on a curve in the shape of an eight. The deviation from the circular shape to an elliptical orbit is expressed in an asymmetry of the curve, similar to that of the analemma curve of the sun. Orbit corrections in north-south direction require a lot more fuel than shifts along the equator. That is why the operators let old satellites with almost exhausted fuel supplies commute in what is known as inclined orbit , if possible . With an N / S fluctuation of 10 °, the W / O variation is about 0.5 °.
The International Telecommunication Union allocates frequencies and satellite positions so that satellites do not interfere with one another. In the past the distance was 4 ° to the neighboring satellite, which radiated on the same frequency. Due to the great demand for satellite positions, the distances were reduced to 2 °, corresponding to 1400 kilometers. The actual assigned satellite position is a box in which the operators have to position their satellites to ± 0.14 °, which means an east-west drift of less than 100 kilometers. The radial drift must not vary by more than 75 kilometers.
Co-positioning
It is possible to position more than one satellite on a satellite position. In this case, all co-positioned satellites are within the assigned box. Nowadays it is possible to position eight satellites on one satellite position.
Energy supply during eclipse, sun outage
A geostationary satellite draws its energy almost all year round from solar cells. The nodes of the geostationary orbit are to spring and early fall near the line connecting the Sun-Earth and the Earth's shadow . That is why it stands in the earth's shadow for a maximum of 70 minutes at night from March to mid-April and September to mid-October. During the time of this eclipse, the satellites draw their energy from accumulators that were previously charged by the solar cells, or limit their output (example: TV-SAT ). Twice a year, at a certain time of day, the satellite, earth and sun are almost in line. Then, seen from the antenna, the sun is close to the satellite for a few minutes on several consecutive days. The sun's microwave radiation then superimposes that of the satellite, and there is a short-term deterioration or interruption of the satellite connection ( sun outage ). The exact time when this event occurs depends on the position of the satellite in question and the position of the receiver on earth; Furthermore, the antenna diameter and transmission frequency have an impact on the duration of the interruption.
Calculation of the flight altitude
The following calculation is based on the classical Newtonian law of gravitation , thus neglecting relativistic effects. Furthermore, an undisturbed, radially symmetrical gravitational field of the earth is assumed, and the mass of the satellite is said to be negligible compared to the mass of the earth.
In order for the satellite to move in a circular orbit around the earth, it must have a continuous acceleration towards the center of the circle, i.e. H. learn about the center of the earth. The force that triggers this acceleration comes from the gravitational interaction between the earth and the satellite. The following applies:
Left side: weight due to the gravitational interaction between earth mass and satellite mass , right side: central force, which is necessary for a circular path with the radius and the angular velocity . This assumption of a circular path around the center of the Earth is only justified in approximation when the satellite mass can be assumed to be very small compared to the mass of the Earth, that is .
You can then see that the mass of the satellite can be reduced, so the orbit radius is independent of the satellite mass . With the relation and the solving of the equation for the radius of the orbit one obtains:
The sidereal day length is to be used for the orbit time of the satellite . For metrological reasons, the product is known more precisely than the individual terms ( gravitational constant ) and ( earth mass ). The exact numerical values are:
With the above formula, this results in an orbit radius of around 42,164 km. To calculate the flight altitude, the radius of the earth at the equator , 6,378 km, must be subtracted, which is slightly larger than the mean earth radius due to the flattening of the poles . This results in the altitude above the equator at 35,786 km.
Visibility of a satellite from the earth
Since a geostationary satellite is stationary when viewed from an observer on earth, the horizontal and vertical viewing angles (azimuth and elevation) remain constant. They only depend on the latitude and longitude of the observer and the longitude of the satellite. The latitude of the geostationary satellite is always 0, and its distance from the center of the earth is 42,164 km, the radius of the earth is 6,378 km. The viewing angles, which can also be used for antenna alignment, are calculated as follows:
Difference in geographic longitudes
- Eastern longitudes are positive, western longitudes negative. If it is not between −180 ° and + 180 °, 360 ° must be added or subtracted.
Angle between antenna location and sub-satellite point
This formula calculates the central angle of the orthodromes between the observer's location and the point on the earth's surface directly below the satellite.
Horizontal orientation (azimuth)
The azimuth is measured from north to east.
Some case distinctions are necessary here:
The observer is further west than the satellite |
The observer is at the same longitude as the satellite |
The observer is further east than the satellite |
The observer is on the longitude opposite the satellite |
|
(Observer in the northern hemisphere) |
The azimuth is between 0 ° and 180 ° | The azimuth is 180 ° (south) | The azimuth is between 180 ° and 360 ° | The azimuth is 0 ° (north) |
(Observer at the equator) |
The satellite is directly above the observer in the zenith . The azimuth is not defined, the elevation is 90 °. | The satellite is on the opposite side of the earth in nadir . The azimuth is not defined, the elevation is −90 °. | ||
(Observer in the southern hemisphere) |
The azimuth is 0 ° (north) | The azimuth is 180 ° (south) |
Vertical alignment (elevation)
The elevation is the angle between the horizon and the satellite. If the satellite is below the horizon, the elevation is negative.
Distance to the satellite
The distance to the satellite is at least 35,786 km for a satellite at the zenith. For a satellite on the horizon, this value can rise to 41,670 km.
Numerical simplification
The ratio is 0.151, so the formulas for elevation and distance can also be written as follows:
graphic
The graphic on the right shows the geostationary satellite orbit for various positions in the northern hemisphere. A geostationary satellite can no longer be seen from Earth above 81 ° latitude. At 70 ° latitude (dashed line) it is a maximum of 11 ° above the horizon in the south. At about east-southeast or west-southwest (63 °) the orbit intersects the horizon. An antenna at 50 ° latitude (dotted line) can use the area from south to ESE or WSW for satellite reception, since the satellite is sufficiently high above the horizon with at least 10 ° elevation.
Footprint
The coverage area of a geostationary satellite (theoretically a maximum of 42% of the earth's surface) depends on the directivity of its antennas. Signals from antennas with low directivity can be received wherever there is geometrical visibility to the satellite. With high gain antennas, the reception area can be focused on selected areas on earth. European satellite operators often want their satellites only to illuminate Europe. The diagram provides information, for example, that an antenna with a diameter of 2 meters in the Ku band covers an area of 500 kilometers in diameter on earth. With suitable multibeam antennas and beam-forming elements , an elongated irradiation surface is created that covers the European mainland from the Iberian Peninsula to Poland.
Another method for influencing the shape of the footprint is for the satellite to use elliptical transmitting antennas, which can be attached to the satellite at an angle to shape the transmission area more precisely. These bundle the signal more strongly on their wider side, so that the footprint on earth becomes narrower in the corresponding direction.
Examples of geostationary satellites
Communication satellites
Weather satellites
See also
Web links
- Online calculation of sun outages for TV Satellite (english)
- Several pages on geostationary satellites from the Space Debris Video 2005
- Time lapse of the starry sky, geostationary satellites can be seen as immobile, slowly flashing points.
Individual evidence
- ^ Arianespace reveals manifest, notes launch market variety. In: Spacenews. September 9, 2019, accessed September 9, 2019 .
- ↑ a b c Peter B. de Selding: Out-of-Control Satellite Threatens Other Nearby Spacecraft. space.com, May 3, 2010, accessed May 4, 2010 (English): “Depending on their position at the time of failure, these satellites tend to migrate toward one of two libration points, at 105 degrees west and 75 degrees east . Figures compiled by XL Insurance of New York, an underwriter of space risks, say that more than 160 satellites are gathered at these two points "
- ↑ a b E. M. Soop: Handbook of Geostationary Orbits. Springer Science & Business Media, 1994, ISBN 978-0-792-33054-7 , p. 71 ( limited preview in Google book search).
- ^ A b Peter Berlin: The Geostationary Applications Satellite. Cambridge University Press, 1988, ISBN 978-0-521-33525-6 , p. 64 ( limited preview in Google Book Search).
- ↑ Wolfgang Steiner, Martin Schagerl: Raumflugmechanik , Springer, Berlin, Heidelberg 2004, ISBN 3-540-20761-9 , p. 156 f.
- ↑ Karl-Otto Saur: Klipp und klar, 100 × television and radio . Bibliographisches Institut AG, Mannheim 1978, ISBN 3-411-01711-2 , p. 68
- ↑ H. Paul Shuch: Calculating antenna bearings for geostationary satellites. (PDF) In: Ham Radio 11 (5). May 1978, pp. 67-69 , archived from the original on September 28, 2006 ; accessed on January 24, 2009 .
- ^ Hans-Martin Fischer: European news satellites, From Intelsat to TV-Sat . Stedinger, Lemwerder 2006, ISBN 3-927697-44-3 , p. 45.