Graphite intercalation compounds
Graphite intercalation compounds are complex materials with the formula CX m , where the ion X n + or X n− is embedded ( intercalated ) between the oppositely charged carbon layers . Typically, m is significantly less than 1. The resulting materials are usually clearly colored solids that have a wide range of electrical and redox properties in potential applications.
Manufacture and construction
It is generally represented by treating graphite with a strong oxidizing or reducing agent :
- C + m X → CX m
This reaction is reversible.
The host lattice, i.e. the graphite, and the guest component X interact by means of charge transfer . An analogous process forms the basis of commercial lithium-ion batteries .
In a graphite intercalation compound, however, not every layer has to be occupied by the guest component. This is only the case in the so-called level 1 connections , in which graphite and guest component layers actually alternate. In level 2 connections , on the other hand, two graphite layers without a guest component alternate with a guest component layer. The actual composition, however, may vary and therefore the resulting compounds are often examples of non-stoichiometric compounds . For this reason, the exact composition is therefore given along with the stage.
Due to the guest ions, the individual layers of the graphite have greater gaps than in free graphite.
Examples
Alkali and alkaline earth derivatives
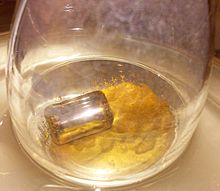
One of the best studied graphite intercalation, KC 8 is, by melting of potassium prepared by graphite powder. The potassium is absorbed by the graphite and the color of the material changes from black to bronze. The resulting solid is pyrophoric . The composition can be explained by the assumption that the potassium – potassium distance corresponds twice to that between two hexagons in the carbon skeleton. The bond between the graphite layers and the potassium cations is also ionic and the electrical conductivity of the material is greater than that of α-graphite. KC 8 is a superconductor with a very low critical temperature of T c = 0.14 K. The heating of KC 8 leads to the formation of a series of decomposition products in which potassium atoms are eliminated.
- 3 KC 8 → KC 24 + 2 K
Via the intermediate KC 24 (blue solid), KC 36 , KC 48 , KC 60 is finally formed .
The stoichiometry MC 8 was observed for the metals potassium, rubidium and cesium . For smaller ions such as Li + , Sr 2+ , Ba 2+ , Eu 2+ , Yb 3+ and Ca 2+ , the limiting stoichiometry is MC 6 . Calcium graphite CaC 6 is produced by immersing highly oriented pyrolytic graphite in a liquid lithium-calcium alloy for 10 days at 350 ° C. The product crystallizes in the space group R 3 m and the distances between the carbon layers increase from 3.35 to 4.52 Å due to the calcium incorporation, while the carbon-carbon distances increase from 1.42 to 1.44 Å.
If barium and ammonia are stored at the same time , the cations are solvated, which leads to a stoichiometry of Ba (NH 3 ) 2.5 C 10.9 in stage 1 . For a mixture of cesium , hydrogen and potassium, the stoichiometry in stage 1 is CsC 8 · K 2 H 4/3 C 8 .
In contrast to other alkali metals, the amount of intercalating sodium is relatively small. Quantum mechanical calculations explain this phenomenon with the fact that, compared to other alkali or alkaline earth metals , sodium and magnesium only form weak chemical bonds with a substrate. This behavior can be traced back to the comparison between the trends in the ionization energy and the ion-substrate coupling in a column of the periodic table . However, a considerable amount of sodium can be stored in graphite if the ion is in a solvent cage, i.e. if co-intercalation takes place.
It was also possible to store a complex magnesium (I) species in graphite.
Graphite hydrogen sulfate, perchlorate and hexafluoroarsenate: Oxidized derivatives
The intercalation compounds graphite hydrogen sulfate and graphite perchlorate can be obtained by reacting graphite with strong oxidizing agents in the presence of strong acids. In contrast to the alkali and alkaline earth derivatives, the graphite layers are oxidized:
In graphite perchlorate, the planar carbon layers are spaced 7.94 Å apart. The ClO 4 - anions are located between them . The cathodic reduction of graphite perchlorate is analogous to the heating of KC 8 and leads to the sequential elimination of HClO 4 .
Both graphite hydrogen sulfate and graphite perchlorate are better electrical conductors than graphite. Their conductivity is based on a hole mechanism .
The reaction of graphite with [O 2 ] + [AsF 6 ] - leads to the salt [C 8 ] + [AsF 6 ] - .
Metal halide derivatives
There are also intercalation compounds of metal halides in graphite. The most extensively investigated were chloride derivatives with the structure MCl 2 (M = Zn , Ni , Cu , Mn ), MCl 3 (M = Al , Fe , Ga ) or MCl 4 (M = Zr , Pt ). These materials consist of closely packed metal halide layers between the carbon layers.
The derivative C ~ 8 FeCl 3 has properties of spin-glass . It also turned out to be a productive system for investigating phase transitions.
An n-stage graphite magnetic intercalation compound has n graphite layers separating successive magnetic layers. As the number of steps increases, the interaction between the spins in successive magnetic layers becomes weaker and 2D magnetism can occur.
Halogen and oxide derivatives
Chlorine and bromine can be reversibly intercalated in graphite, while iodine cannot be intercalated at all. With fluorine, however , the process is irreversible. In the case of bromine, the following stoichiometries are known: C n Br for n = 8, 12, 14, 16, 20 and 28.
Due to the irreversibility of the intercalation of fluorine, graphite fluoride is often not classified as an intercalation compound. It has the empirical formula (CF) x and is obtained through the reaction of fluorine with graphitic carbon at 215 to 230 ° C. It's grayish, white, or yellow, and the bond between the fluorine and carbon atoms is covalent. Tetracarbon monofluoride (C 4 F) is produced by reacting graphite with a mixture of fluorine and hydrogen fluoride at room temperature, has a black-bluish color and is electrically non-conductive. It was used as a cathode material in a type of primary (non-rechargeable) lithium battery .
Graphite oxide is an unstable, yellow solid.
Properties and uses
Graphite intercalation compounds have been of interest to materials scientists for many years because of their electronic and electrical properties.
Superconductivity
Among the superconducting graphite intercalation compounds, CaC 6 has the highest critical temperature with T c = 11.5 K. This increases under elevated pressure and is at 8 GPa example 15.1 K. The superconductivity of this compound class is on the role of an intermediate layer state (English: interlayer state ) is returned. This is an electron-like band that is about 2 eV above the Fermi energy . Superconductivity occurs only in the case of an occupation of this intermediate layer state. The analysis of pure CaC 6 by means of high-quality ultraviolet radiation was carried out using angle-resolved photoelectron spectroscopy and showed that a superconductive gap in the π * band contributes significantly to the overall strength of the electron-phonon coupling of the π * interlayer-intermediate band interaction.
Reganzien in chemical synthesis
The bronze-colored KC 8 is one of the strongest known reducing agents . It was also used as a catalyst for polymerization reactions and as a coupling reagent for halogen aromatic compounds with biphenyls . In one study, freshly made KC 8 was reacted with 1-iodododecane, resulting in a modification that is soluble in chloroform . The modification can be described as micrometer-sized carbon flakes with long alkyl chains that increase solubility. Another potassium graphite compound, KC 24 , was used as a neutron monochromator . A new essential application for potassium graphite has arisen with the invention of potassium ion batteries . Similar to lithium ion batteries , the potassium ion battery uses a carbon-based instead of a metallic anode . In this case, the stable structure of potassium graphite is an important advantage.
See also
- Magnesium diboride , a compound made up of hexagonal planar layers of boron instead of layers of carbon
Additional information
- Suzuki, Masatsugu., Endo, Morinobu .: Graphite Intercalation Compounds and Applications. Oxford University Press, USA, Oxford 2003, ISBN 978-0-19-535184-2 ( OCLC 437108496 [accessed February 29, 2020]).
- MS Dresselhaus, G. Dresselhaus: Intercalation compounds of graphite . In: Advances in Physics . tape 30 , no. 2 , April 1981, ISSN 0001-8732 , pp. 139-326 , doi : 10.1080 / 00018738100101367 . , reissued as: MS Dresselhaus, G. Dresselhaus: Intercalation compounds of graphite . In: Advances in Physics . tape 51 , no. 1 , January 2002, ISSN 0001-8732 , p. 1-186 , doi : 10.1080 / 00018730110113644 .
- D. Savoia, C. Trombini, A. Umani-Ronchi: Applications of potassium-graphite and metals dispersed on graphite in organic synthesis . In: Pure and Applied Chemistry . tape 57 , no. 12 , January 1, 1985, ISSN 1365-3075 , pp. 1887-1896 , doi : 10.1351 / pac198557121887 .
- Itsuko S. Suzuki, Ting-Yu Huang, Masatsugu Suzuki: Magnetic phase diagram of the stage-1 CoCl 2 graphite intercalation compound: Existence of metamagnetic transition and spin-flop transitions . In: Physical Review B . tape 65 , no. 22 , June 13, 2002, ISSN 0163-1829 , p. 224432 , doi : 10.1103 / PhysRevB.65.224432 .
- DG Rancourt, C. Meschi, S. Flandrois: S = 1/2 antiferromagnetic finite chains effectively isolated by frustration: CuCl 2 -intercalated graphite . In: Physical Review B . tape 33 , no. 1 , January 1, 1986, ISSN 0163-1829 , pp. 347-355 , doi : 10.1103 / PhysRevB.33.347 .
Individual evidence
- ^ A b Earnshaw, A. (Alan): Chemistry of the elements . 2nd ed. Butterworth-Heinemann, Boston, Mass. 1997, ISBN 0-585-37339-6 ( OCLC 48138330 [accessed March 14, 2020]).
- ↑ HP Boehm, R. Setton, E. Stumpp: Nomenclature and terminology of graphite intercalation compounds (IUPAC Recommendations 1994) . In: Pure and Applied Chemistry . tape 66 , no. 9 , January 1, 1994, ISSN 1365-3075 , pp. 1893-1901 , doi : 10.1351 / pac199466091893 ( degruyter.com [Retrieved on February 8, 2020]).
- ↑ a b D.M. Ottmers, HF Rase: Potassium graphites prepared by mixed-reaction technique . In: Carbon . 4, No. 1, 1966, ISSN 0008-6223 , pp. 125-127. doi : 10.1016 / 0008-6223 (66) 90017-0 .
- ^ A b c d Catherine E. Housecroft, Alan G. Sharpe: Inorganic Chemistry . 3rd ed. Pearson, 2008, ISBN 978-0-13-175553-6 , pp. 386 .
- ^ NIST Ionizing Radiation Division 2001 - Major Technical Highlights . physics.nist.gov
- ^ A b Nicolas Emery, Claire Hérold, Jean-François Marêché, Philippe Lagrange: Synthesis and superconducting properties of CaC 6 . In: Science and Technology of Advanced Materials . tape 9 , no. 4 , December 2008, ISSN 1468-6996 , p. 044102 , doi : 10.1088 / 1468-6996 / 9/4/044102 , PMID 27878015 , PMC 5099629 (free full text) - ( tandfonline.com [accessed February 8, 2020]).
- ↑ a b Yuanyue Liu, Boris V. Merinov, William A. Goddard: Origin of low sodium capacity in graphite and generally weak substrate binding of Na and Mg among alkali and alkaline earth metals . In: Proceedings of the National Academy of Sciences . 113, No. 14, April 5, 2016, pp. 3735-3739. arxiv : 1604.03602 . bibcode : 2016PNAS..113.3735L . doi : 10.1073 / pnas.1602473113 . PMID 27001855 . PMC 4833228 (free full text).
- ^ W. Xu, H. Zhang and MM Lerner, "Graphite Intercalation by Mg Diamine Complexes", Inorg. Chem. 2018, 57, 14, 8042–8045 Publication Date: June 25, 2018 https://doi.org/10.1021/acs.inorgchem.8b01250
- ^ SE Millman, GO Zimmerman: Observation of spin glass state in FeCl 3 : intercalated graphite . In: Journal of Physics C: Solid State Physics . 16, No. 4, 1983, p. L89. bibcode : 1983JPhC ... 16L..89M . doi : 10.1088 / 0022-3719 / 16/4/001 .
- ↑ Nicolas Emery, Claire Hérold, Jean-François Marêché, Philippe Lagrange: Synthesis and superconducting properties of CaC 6 . In: Science and Technology of Advanced Materials . tape 9 , no. 4 , December 2008, ISSN 1468-6996 , p. 044102 , doi : 10.1088 / 1468-6996 / 9/4/044102 , PMID 27878015 , PMC 5099629 (free full text) - ( tandfonline.com [accessed February 8, 2020]).
- ↑ a b Gábor Csányi, PB Littlewood, Andriy H. Nevidomskyy, Chris J. Pickard, BD Simons: The role of the interlayer state in the electronic structure of superconducting graphite intercalated compounds . In: Nature Physics . tape 1 , no. 1 , October 2005, ISSN 1745-2473 , p. 42–45 , doi : 10.1038 / nphys119 ( nature.com [accessed February 8, 2020]).
- ^ A b Soma Chakraborty, Jayanta Chattopadhyay, Wenhua Guo, W. Edward Billups: Functionalization of Potassium Graphite . In: Angewandte Chemie International Edition . tape 46 , no. 24 , June 11, 2007, pp. 4486-4488 , doi : 10.1002 / anie.200605175 ( wiley.com [accessed February 8, 2020]).