IMPATT diode
The IMPATT diode is a special diode for generating high frequencies . The name is derived from the English term Impact Ionization Avalanche Transit Time Diode , in German it is called Avalanche Transit Time Diode (LLD).
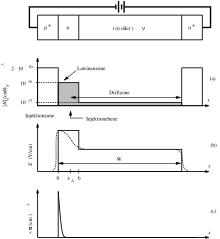
The main representatives of the IMPATT diode family are the read diode, the one-sided abrupt pn junction , the double-sided double drift diode, hi-lo and lo-hi-lo diodes and the pin diode . Further transit time diodes are the BARITT diode , the DOVETT diode and the TRAPATT diode .
The IMPATT diode is one of the most powerful semiconductor generators up to 300 GHz and thus an important source for microwaves of such high frequency or millimeter waves . It achieves over 10 times higher performance than the Gunn diode in the frequency range 10–150 GHz.
It is used in electronic circuits in which high-frequency oscillators are used. These include transmitters in millimeter wave communication, radar for civil air and ground use, or for controlling missiles in the military sector and similar applications.
The advantages of IMPATT diodes are that they have a higher efficiency (12–20% in the range of 5–10 GHz) than Gunn diodes and reflex klystrons, they have a long service life and, compared to Gunn diodes, they have high RF outputs (e.g. at 9.5 GHz 3.5 W continuous and 15 W pulsed).
The high phase noise and the high reactances are disadvantageous . The reactances are strongly dependent on the oscillation amplitude and must therefore be taken into account in the circuit design so that the diode is not detuned or even destroyed.
Working principle
IMPATT diodes are created through the use of impact ionization and transit time properties of the electrons in semiconductor structures to produce a dynamic, effective negative differential resistance at microwave frequencies. This resistance is often referred to by the English term negative dynamic resistance or the corresponding abbreviation NDR. A negative resistance generally means an energy source in the form of a current or voltage source. The negative resistance results from two delay times, which in turn cause a time delay between the high-frequency current and the high-frequency voltage . In this context, one speaks of a negative phase shift between the high-frequency current and the high-frequency voltage. One delay time arises from the “avalanche breakdown time” caused by the avalanche breakdown, the second shift arises from transit time delays caused by the diode in the n + pip + structure or p + nin + structure in the drift region. If the two delay times add up to half a period, there is a negative electrical resistance at the corresponding frequency.
history
The first experimental observation of an IMPATT oscillation by Johnston, deLoach and Cohen took place in 1965. It was a silicon diode operated with reverse polarity in the avalanche breakdown range and microwave range.
The physicist William B. Shockley envisaged this negative resistance as early as 1954 due to its structural simplicity. The big advantage is that, in contrast to transistors, which have three connections, it is a component with two connections. In 1958, Read proposed the development of a high-frequency semiconductor diode which should consist of an avalanche zone at one end and a drift zone with a relatively high resistance. The drift zone serves as a transit time range for the generated charge carriers. CA Lee, RL Batdorf, W. Wiegman, and G. Kaminsky were the first to document this oscillation. Misawa, Gilden and Hines developed the small signal theory for this , which underpins that a negative resistance with IMPATT properties can be obtained from diode barriers or semiconductor-metal contacts regardless of the doping profile.
Inpatient case
The following text considers the field distribution, the breakdown voltage and space charge effects under stationary conditions. The first picture above shows the doping profile and the field distribution of an idealized read diode. The ionization integrand is given by:
here, α n and α p are the respective ionization rates of electrons and holes. And W is the depletion zone width. In between is the drift zone.
The avalanche breakdown condition is given by:
Due to the strong dependence of the alphas on the electric field, one can see that the avalanche zone is very local. That is, the multiplication process in a very narrow region near the highest electric field intensity between 0 and x A takes place.
Special cases of the Read diode are the one-sided abrupt p + -n junction and the pin diode, also known as Misawa diode. Another embodiment is the double-sided abrupt p + -pnn + transition. For silicon, the avalanche zone is located near the center of the depletion zone. Because α n and α p are very different for silicon. In GaP , the alphas are almost the same and the following simplification can be made
therefore the avalanche zone is almost symmetrical to the depletion zone center.
Breakdown voltage
The general methods for determining the breakdown voltage in abrupt transitions can also be used for symmetrical double-sided abrupt transitions such as p + -pnn + transitions. It is calculated to
In this equation, the maximum field at the point x = 0. Depending on the material , x is shifted from the plane of symmetry due to the different alphas. The maximum field at the point x = 0 can be read from a diagram as soon as the doping is known. Then I have the breakdown voltage calculated using the above equation. The reverse voltage at breakdown is U B - U e . In this equation, U e is the diffusion voltage (built-in voltage of the pn junction ), which is given by 2⋅ (k⋅T / q) ⋅ln ( N B / n i ). For symmetrical abrupt transitions, the diffusion voltage is negligible in practice.
The breakdown voltage and depletion zone width for the read diode and for the hi-lo diode are given by the following equations
The epitaxial layer is decisive for the width of the depletion zone in the Read diode. The same tables for the maximum field can be used for the calculation as for the abrupt pn transition. Provided that the n or p region is given, these values apply almost completely (with a deviation of about one percent) to the read diode and hi-lo diode as well. However, with the restriction that x A is smaller than b .
The breakdown voltage of a lo-hi-lo diode with a very small Q " lump " is given by
In this equation, Q is the number of impurities per square centimeter in the "lump". The maximum field can be calculated from the field-dependent ionization coefficient.
Regions
The avalanche zone of an ideal pin diode extends over the entire intrinsic layer. However, this region for the read diode and the pn junctions is limited to a very narrow region in the vicinity of the metallurgical junction. A good approximation for x A can be obtained with the help of the avalanche breakdown condition. With increasing distance of x from the metallurgical transition, the contribution to the integral decreases, so that a reasonable contribution can be assumed at 95%. For the read diode, x A is calculated from
Similarly, for the hi-lo diode, the one-sided abrupt transition and the double-sided transition (in the event that they are operated at breakdown voltage), the following equation is used
Effects
In operating conditions, the high current densities during the avalanche breakdown, which cause a considerable increase in temperature at the junction and space charge carrier effects, must be taken into account.
The ionization rates of electrons and holes decrease with increasing temperature. As a result, the breakdown voltage increases with increasing temperatures. As the DC power (the product of reverse voltage and reverse current) increases, both the temperature at the junction and the breakdown voltage increase. In order to avoid a total failure of the component due to an extreme increase in heat in spatially very limited areas, IMPATT diodes must have suitable heat dissipation.
Space charge carrier effects result from the generation of charge carriers, which cause fluctuations in the electric field in the depletion zone. This leads to a change in the differential DC resistance. This increases for abrupt transitions and decreases for pin diodes.
Dynamic case
In the following, the injection phase and the transit time of an ideal component are considered. This means that the current is injected at the point with a phase of φ and that the injected charge carriers move with a saturation speed of in the drift region. The magnitude of the AC line current density at the point is equal to the total AC current density with phase shift.
The total alternating current in the drift region is made up of the sum of the line current and the displacement current. Here is the complex alternating field.
The complex alternating field is obtained from the two equations above.
The impedance Z is obtained by integration .
In this equation is the capacity per area . And is the transit angle and is calculated as . For the real and the imaginary part we get the following expressions from the above equation
Small signal analysis
The first considerations on small signal analysis were made by William T. Read. M. Gilden and MF Hines developed these theories further. The following simplifications were made: and the saturation speeds of electrons and holes are the same.
According to literature, the current density is at the avalanche breakdown and is the total alternating current density . The current density at the avalanche breakdown is the alternating current density of the particles in the avalanche region. Assuming a thin avalanche region, there are no delay times for the current density before entering the drift region. With the second assumption, when the current density in avalanche breakdown as a continuous wave propagates (in which only the phase changes), the drift velocity calculated to
Large signal analysis
The picture shows a read diode in the large-signal working area.
Manufacturing
Epitaxy , diffusion and ion implantation are used to manufacture IMPATT diode crystals . There are also combinations of these methods. It is crucial that the sequence and amount of the doping is carried out with high accuracy.
Frequently is molecular beam epitaxy (MBE) was used. With MBE, the thickness of the doping and the layer can be determined on an almost atomic scale. It is used especially for millimeter wave diodes. It is not only the most accurate, but also the most expensive method.
The diode chip is finally attached in a metal housing. The diffused side or the metal electrode is in contact with a metallic surface so that the heat loss can be dissipated well. Common housings are z. B. screwable and press the end with the chip on the heat sink, which is also the waveguide wall.
The semiconductor material silicon carbide is often used for Impatt diodes .
Shift sequences
Numerous different doping profiles have been investigated. A relatively simple possible doping layer sequence is the p + -nn + structure . It is produced either by double epitaxy or by diffusion into an epitaxial layer. The n + substrate should reduce the series resistance. In order to reduce losses and maintain uniformity, which can be disturbed by the skin effect , the substrate is only a few micrometers in size. The thickness of the epitaxial layer must also be controlled: When the breakdown occurs, no epitaxial layer should be left that would make the component unusable.
Another form is a Schottky transition. The structure is n + -n metal. The structure is similar to the first structure, but has some advantages: The maximum field occurs at the metal-semiconductor interface, the heat generated can be quickly conducted away from the metal contact. The component can also have the shape of a trimmed cone. When the maximum field is shifted from the outside to the inside, the breakthrough takes place within the component. Because the diode can be manufactured at relatively low temperatures, it is possible to keep the original high quality epitaxial layer. A disadvantage, however, is that the metal electrode can be attacked by electrons and holes with high energy - the component does not last long.
The frequently used Hi-lo diode has the structure n + -in metal. It is a modified read diode in which the p + layer has been replaced by a metal contact - it is therefore also a Schottky diode. Since it is primarily a majority charge carrier component, the minority charge carrier storage effect is prevented. This gives the diode a higher efficiency, especially for microwaves. Strict control of the doping profiles is necessary so that a certain frequency can be determined. With the help of a self-limiting anodic etching method, the highly doped layer can be made thinner or the surface can be made lightly doped. As a result, the breakdown occurs at a desired voltage and at the same time a desired frequency arises. The disadvantage is that gallium arsenide reacts with platinum at operating temperature , which shifts the pn junction . This changes the breakdown voltage and decreases the performance. Applying a small amount of platinum (200 to 500 Angstroms ) to the surface of the epitaxial layer, followed by a layer of tungsten or tantalum , will reduce the reaction.
A fourth possibility is the structure according to the n + -npp + scheme, i.e. a double drift diode. It is produced by ion implantation and is useful for diodes that are used to generate millimeter waves . Both the output power and the impedance per area double as a result, so this structure also leads to higher efficiency.
literature
- SM Sze: Physics of Semiconductor Devices. 2nd edition, John Wiley & Sons, 1981, ISBN 0-471-05661-8 , pp. 566-636.
- MS Tyagi: Introduction to Semiconductor Materials and Devices. John Wiley & Sons, 1991, ISBN 0-471-60560-3 , pp. 311-320.
- RL Jonston, BC DeLoach Jr., BG Cohen: A Silicon Diode Oscillator. In: Bell Systems Technical Journal. 44, 1965, p. 369.
- H. Komizo, Y. Ito, H. Ashida, M. Shinoda: A 0.5-W CW IMPATT diode amplifier for high-capacity 11-GHz FM radio-relay equipment. In: IEEE Journal 8, No. 1, 1973, ISSN 0018-9200 , pp. 14-20.
- M.-S. Gupta: Large-Signal Equivalent Circuit for IMPATT-Diode Characterization and Its Application to Amplifiers. In: Microwave Theory and Techniques. IEEE Transactions Volume 21. No. 11. 1973, ISSN 0018-9480 , pp. 689-694.
Web links
- Britney's Guide to Semiconductor Physics (English, Fundamentals of Semiconductor Physics ).
- IMPATT Diode Microwave Oscillators
Individual evidence
- ↑ http://web.eecs.umich.edu/~jeast/martinez_2000_0_2.pdf diagram on page 4
- ↑ http://www.microsemi.com/document-portal/doc_view/9680-msc-impatt-diodes-pdf .
- ↑ http://www.radartutorial.eu/21.semiconductors/hl16.de.html
- ↑ cf. WT Read: A proposed high-frequency negative-resistance diode . In: Bell . 1958, p. 401-466 ( PDF ).
- ↑ M. Gilden, ME Hines: Electronic tuning effects in the read microwave avalanche diode . In: IEEE Transactions on Electron Devices . tape 13 , no. 1 , 1966, p. 169-175 , doi : 10.1109 / T-ED.1966.15652 .
- ↑ a b Simon M. Sze, Kwok K. Ng: Physics of Semiconductor Devices . 3. Edition. John Wiley & Sons, 2006, ISBN 978-0-471-14323-9 , pp. 477 .
- ↑ http://www.radartutorial.eu/21.semiconductors/hl16.de.html