PCR optimization
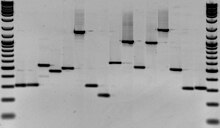
The PCR optimization comprises the targeted modification of the reaction conditions of a polymerase chain reaction .
Optimization parameters
Optimizing PCR-related methods involves changing various parameters in a targeted manner.
- Choice of a suitable thermostable DNA polymerase
- Design of the sequence of the primer ( primer design )
- Change in the concentrations of substrates (DNA template, primer template, dNTP concentration)
- Change in the concentration of products (more copied DNA, correct product, less pyrophosphate),
- Avoidance of interfering substances (partly substrate-like inhibitors )
- Controlling the ambient conditions ( temperature , thermal stability , ionic strength , osmolarity , cofactors ).
Polymerase
The choice of a suitable DNA polymerase has an influence on the synthesis amount and error rate.
Primer
The primer design includes the selection of suitable DNA sequences for the primers and the primer hybridization temperature (synonym annealing temperature) based on them in the PCR. The annealing temperature of the PCR is usually two degrees Celsius below the melting temperature of the primers, which represents a compromise between the most complete possible primer hybridization and the highest possible specificity of the primer binding.
Substrate concentration
The final concentration of the primers during the PCR is between 0.1 μM and 1 μM (mostly 0.4 μM), the concentration of dNTPs for each of the four nucleobases is around 100 - 1000 μM (mostly 400 μM each) and the mass concentration of the DNA of 1 to 20 ng per microliter of the PCR mixture (mostly 200 ng per mixture).
Temperature cycle
The dissociation (melting) of the double-stranded DNA is mostly carried out at 95 ° C. The hybridization ( annealing ) then takes place at a primer-dependent temperature, and finally the DNA is synthesized at the respective optimum of the respective thermostable DNA polymerase at 72 ° C (type A polymerases) or 68 ° C (type B) Polymerases).
Error rate
The error rates of various polymerases ( fidelity ) are known and have been described. Bacterial thermostable DNA polymerases (type A) usually have a higher error rate than archaic ones (type B), which is due to the proof reading exonuclease activity of the B-type polymerases. The error rate of Taq polymerase is 8 · 10 −6 errors per base pair , that of KOD polymerase 3.5 · 10 −6 errors per base pair, that of Tli polymerase and Herculase 2.8 · 10 −6 errors per base pair that of Pfu polymerase 1.3 · 10 −6 errors per base pair and that of Pfu Ultra 4.3 · 10 −7 errors per base pair.
Synthesis rate
The overall synthesis rate of a DNA polymerase depends, among other things, on the basic synthesis rate and the processivity of the polymerase as well as the presence of inhibitors (e.g. inhibiting products, PCR inhibitors).
The basic synthesis rates of different polymerases ( productivity ) have been compared. The synthesis rate of Taq polymerase is around 60 base pairs per second. Among the unmodified thermostable DNA polymerases, only the synthesis rate of the KOD polymerase is above 100 base pairs per second (approx. 120 bp / s). Various mutations that increase the rate of synthesis have been described among the modified thermostable DNA polymerases. The KOD polymerase and some modified thermostable DNA polymerases ( iProof , Pfu Ultra , Phusion , Velocity or Z-Taq ) are used in a PCR variant with shorter amplification cycles ( fast PCR , high-speed PCR ) due to their high synthesis rate .
The processivity (engl. Processivity ) describes the average number of base pairs before a polymerase from the DNA template (engl. Template ) drops. The processivity of the polymerase used limits the maximum distance between the primer and the probe in real-time quantitative PCR . The processivity of a Taq polymerase is around 200 base pairs.
When the dNTPs are heated in aqueous solutions , the cytosine residues in the dCTP continuously deaminate to uracil residues, which reduce the rate of synthesis of the DNA polymerases. In order to counteract this reduction, known as dUTP poisoning , a thermostable dUTPase is occasionally added to products of over 5 kilobases in a PCR with archaic polymerases . Similarly, the adenosine residues in the dATP also deaminate in parallel to form deoxyinosine triphosphate (dITP), which can also lead to an inhibition of the archaic DNA polymerases. Therefore a thermostable dITPase can also be added to the PCR with archaic DNA polymerases.
Since the DNA polymerase catalyzes the DNA chain extension by splitting off pyrophosphate , the product concentration can be increased by adding a thermostable pyrophosphatase . The pyrophosphatase catalyzes the hydrolysis of the pyrophosphate to phosphates , which results in less product inhibition since the equilibrium constant of the reaction is changed in favor of the synthesized DNA.
The Stoffel fragment is a Taq polymerase shortened by the exonuclease function , which increases the product concentration and the thermostability of the enzyme.
False negative results
If a PCR is carried out and no duplicated DNA is detected in the result, although the sequence to be detected was actually present, this is referred to as a false negative result.
Substrate refusal
The “refusal” of difficult substrates ( fussiness ), which occurs occasionally with the archaic polymerases, can lead to false negative results, e.g. B. aDNA , DNA with a high GC content , genomic DNA or DNA with secondary structures . The inhibition of the reaction can be reduced by adding weakly chaotropic molecules such as dimethyl sulfoxide , formamide , trehalose or betaine . The use of chaotropic compounds can lower the melting temperature of the primers by up to 5 ° C, which is why the annealing temperature of the PCR must be adjusted accordingly. An increase in the concentration of magnesium - ions , as a cofactor of the thermostable DNA polymerases, 1.5 mM also increases the product concentration at the expense of the error rate. Some alkylated nucleobases cannot base-pair with the four natural nucleobases, which is why the amplification is interrupted at these points. Base pairing in the PCR can be carried out by adding certain modified nucleic bases.
Inhibitors
Various substances can interfere with thermostable DNA polymerases in the PCR, e.g. B. antivirals , hemoglobin , heparin , polysaccharides , hormones , IgG , lactoferrin , myoglobin , bile acids and their salts , uric acid and their salts, phenol , polyphenols , proteases , divalent cations except magnesium, EDTA and other chelators , humic acids , clay , collagen , Hematins , indigo , melanin and tannins . In general, samples containing these materials require further DNA purification prior to use in PCR. If higher concentrations of polyphenols are present, polyvinylpyrrolidone can be added to bind them. Also, bovine serum albumin used for binding inhibitors.
False positive results
In a PCR, a false positive result is the generation of PCR products that do not correspond to the desired DNA sequence to be replicated. It may lead to undesired bands in an agarose gel .
Contaminations
DNA contamination and unspecific hybridization of the primers before and during the PCR can lead to false positive results. Contamination in particular is a major problem. Often DNA from people who are involved in the analysis or who were involved in the manufacture of components gets into the sample. In forensic use (see DNA analysis and genetic fingerprints ), the Heilbronn phantom made headlines: For a long time, criminologists chased an alleged serial offender until it turned out that the DNA actually came from an employee of a supplier company. Bacterial contamination, contamination by parasites or food components and the like can also be problematic in biological samples. For a long time , the worm-like organism Xenoturbella was wrongly viewed as a representative of the molluscs , because DNA from the intestine, which came from food organisms , was mistakenly assumed to be the DNA of the worm itself. Especially with very old, possibly partially fragmented DNA, e.g. B. from fossils , special care is required. This is especially true when sequences related to humans, e.g. B. the genome of the Neanderthal man is analyzed.
Occasionally a uracil-N-glycosylase is added to the reaction mixture for the enzymatic breakdown of possible DNA contamination , which is denatured in the first temperature cycle. The uracil-N-glycosylase degrades uracil-containing PCR products that arise when the laboratory has previously switched from dTTP to dUTP for PCR purely for detection purposes and has used type A polymerases for this purpose. However, this can lead to false negative results at low concentrations of DNA starting material.
Hot start
The template specificity of the polymerases to avoid binding to undesired DNA templates or mis-bound primers, and the resulting generation of undesired reaction products before the start of the first temperature cycle, is increased through the use of hot-start polymerases . Examples are the Hot-Start Pfu Turbo inhibited with an antibody , the Platinum Pfx as a commercial KOD polymerase with an inhibiting antibody and the Platinum Taq as the antibody-inhibited Taq polymerase. These polymerases are inhibited , among other things, by inactivation with formaldehyde , by complexing the magnesium with phosphates or by binding an antibody to their active center . When heated to 95 ° C , the formaldehyde hydrolyzes , alternatively the magnesium ions are released or the antibody is denatured . A fourth variant is a polymerase adsorbed on latex beads via hydrophobic effects , which dissolves when the temperature rises. In the fifth, oldest, variant, the reaction mixture without polymerase is covered with wax and the polymerase is applied to the cooled wax. When heated, the wax layer melts and the polymerase mixes with the reaction mixture.
Substrate specificity
The preference for individual nucleotides by a thermostable DNA polymerase is known as nucleotide specificity (English bias 'preference', 'bias'). In the case of PCR-based DNA sequencing with chain termination substrates ( dideoxy method ), their uniform incorporation and thus the uniform generation of all chain termination products is often desired in order to enable higher sensitivity and easier evaluation. For this purpose, a KlenTaq polymerase was generated by deletion and, by site-specific mutagenesis, a phenylalanine at position 667 was exchanged for tyrosine (F667Y for short) and designated as Thermo Sequenase . This polymerase can also be used for the incorporation of fluorescently labeled dideoxynucleotides .
A change in the template specificity of DNA to RNA can be used to generate thermostable RNA-dependent DNA polymerases, which are also referred to as thermostable reverse transcriptases . Conventional reverse transcriptases of retroviral origin used for RT-PCR , such as AMV and MoMuLV reverse transcriptase, are not thermostable at 95 ° C. At the lower temperatures of reverse transcription with these enzymes, however, unspecific bindings of primers to the DNA template and secondary structures in the DNA template occur, which on the one hand favor undesired products and on the other hand can prevent the synthesis of the correct product. However, AMV reverse transcriptase can be used up to 70 ° C.
Therefore, the template specificity of thermostable DNA polymerases was initially reduced by exchanging the cofactor (divalent magnesium ions) for divalent manganese ions , so that with a DNA-dependent thermostable polymerase, RNA could also be used in an RT-PCR as a template for the synthesis of DNA. Since the synthesis rate of Taq polymerase with manganese ions was relatively low, the Tth polymerase was increasingly used in this variant of RT-PCR . However, the addition of manganese ions also increased the number of defective products and increased the amount of template DNA required, which is why these enzymes are rarely used for reverse transcription today. These problems could be avoided with the thermostable 3173 polymerase made from thermophilic bacteriophages , which withstands the high temperatures of a PCR for a long time and prefers RNA as a template.
literature
- J. Sambrook , T. Maniatis , DW Russel: Molecular cloning: a laboratory manual . Cold Spring Harbor Laboratory Press; 3rd edition (2001), ISBN 0-87969-577-3 .
- Cornel Mülhardt: The Experimentator : Molecular Biology / Genomics , Springer 2008, ISBN 3-8274-2036-9 .
Individual evidence
- ↑ J. Cline, JC Braman, HH Hogrefe: PCR fidelity of pfu DNA polymerase and other thermostable DNA polymerases. In: Nucleic Acids Res . (1996), Vol. 24 (18), pp. 3546-51. PMID 8836181 ; PMC 146123 (free full text).
- ↑ a b c d Bahram Arezi, Weimei Xing, Joseph A. Sorge, Holly H. Hogrefe: Amplification efficiency of thermostable DNA polymerases . In: Analytical Biochemistry . tape 321 , no. 2 , October 15, 2003, p. 226-235 , doi : 10.1016 / S0003-2697 (03) 00465-2 , PMID 14511688 . PDF .
- ↑ FreePatentsOnline 20100203594
- ↑ FreePatentsOnline 20130034879
- ↑ FreePatentsOnline 20090280539
- ^ Bernard A. Connolly: Recognition of deaminated bases by archaeal family-B DNA polymerases . In: Biochemical Society Transactions . tape 37 , no. 1 , February 2009, p. 65-68 , doi : 10.1042 / BST0370065 , PMID 19143603 .
- ↑ Y. Cho, HS Lee, YJ Kim, SG Kang, SJ Kim, JH Lee: Characterization of a dUTPase from the hyperthermophilic archaeon Thermococcus onnurineus NA1 and its application in polymerase chain reaction amplification . In: Mar Biotechnol (NY) (2007), Vol. 9 (4), pp. 450-8. PMID 17549447 .
- ↑ Holly H. Hogrefe, Connie J. Hansen, Bradley R. Scott, Kirk B. Nielson: Archaeal dUTPase enhances PCR amplifications with archaeal DNA polymerases by preventing dUTP incorporation . In: Proceedings of the National Academy of Sciences . tape 99 , no. 2 , January 22, 2002, p. 596-601 , doi : 10.1073 / pnas.012372799 , PMID 11782527 , PMC 117351 (free full text).
- ↑ YJ Kim, YG Ryu, HS Lee, Y. Cho, ST Kwon, JH Lee, SG Kang: Characterization of a dITPase from the hyperthermophilic archaeon Thermococcus onnurineus NA1 and its application in PCR amplification. In: Appl Microbiol Biotechnol. (2008), Volume 79 (4), pp. 571-8. PMID 18438658 .
- ↑ a b P. B. Vander Horn, MC Davis, JJ Cunniff, C. Ruan, BF McArdle, SB Samols, J. Szasz, G. Hu, KM Hujer, ST Domke, SR Brummet, RB Moffett, CW Fuller: Thermo Sequenase DNA polymerase and T. acidophilum pyrophosphatase: new thermostable enzymes for DNA sequencing. In: Biotechniques (1997), Volume 22 (4), pp. 758-62, 764-5. PMID 9105629 . PDF ( Memento of the original from February 1, 2014 in the Internet Archive ) Info: The archive link was inserted automatically and has not yet been checked. Please check the original and archive link according to the instructions and then remove this notice. .
- ↑ SY Park, B. Lee, KS Park, Y. Chong, MY Yoon, SJ Jeon, DE Kim: Facilitation of polymerase chain reaction with thermostable inorganic pyrophosphatase from hyperthermophilic archaeon Pyrococcus horikoshii. In: Appl Microbiol Biotechnol. (2010), Volume 85 (3), pp. 807-12. PMID 19882151 .
- ↑ PR Winship: An improved method for directly sequencing PCR amplified material using dimethyl sulphoxide . In: Nucleic Acids Research . tape 17 , no. 3 , February 11, 1989, p. 1266 , PMID 2922271 , PMC 331767 (free full text).
- ^ SA Masoud, LB Johnson, FF White: The sequence within two primers influences the optimum concentration of dimethyl sulfoxide in the PCR . In: Genome Research . tape 2 , no. 1 , January 8, 1992, p. 89-90 , doi : 10.1101 / gr.2.1.89 , PMID 1490180 .
- ↑ G. Sarkar, S. Kapelner, SS Sommer: Formamide can dramatically improve the specificity of PCR. In: Nucleic Acids Res. (1990), Vol. 18 (24), p. 7465. PMID 2259646 ; PMC 332902 (free full text).
- ^ Andrej-Nikolai Spiess, Richard Ivell: A Highly Efficient Method for Long-Chain cDNA Synthesis Using Trehalose and Betaine . In: Analytical Biochemistry . tape 301 , no. 2 , February 15, 2002, p. 168-174 , doi : 10.1006 / abio.2001.5474 , PMID 11814287 .
- ^ KA Eckert, TA Kunkel: DNA polymerase fidelity and the polymerase chain reaction . In: Genome Research . tape 1 , no. 1 , January 8, 1991, p. 17-24 , doi : 10.1101 / gr.1.1.17 , PMID 1842916 .
- ↑ Laura A. Wyss, Arman Nilforoushan, Fritz Eichenseher, Ursina Suter, Nina Blatter, Andreas Marx, Shana J. Sturla: Specific Incorporation of an Artificial Nucleotide Opposite a Mutagenic DNA Adduct by a DNA Polymerase. In: Journal of the American Chemical Society. 137, 2015, pp. 30-33, doi: 10.1021 / ja5100542 .
- ^ C. Schrader, A. Schielke, L. Ellerbroek, R. Johne: PCR inhibitors - occurrence, properties and removal . In: J Appl Microbiol . 2012, doi : 10.1111 / j.1365-2672.2012.05384.x , PMID 22747964 . PDF .
- ↑ KL Faber, EC Person, WR Hudlow: PCR inhibitor removal using the NucleoSpin® DNA Clean-Up XS kit. In: Forensic science international. Genetics. Volume 7, Number 1, January 2013, pp. 209-213, ISSN 1878-0326 . doi: 10.1016 / j.fsigen.2012.06.013 . PMID 22784879 .
- ^ Joseph Warren: A Review of PCR Inhibition and It's Implications for Human Identity Testing. Association of Forensic DNA Analysts and Administrators. (PDF) .
- ↑ Priyum K. Koonjul, Wolf F. Brandt, George G. Lindsey, Jill M. Farrant: Inclusion of polyvinylpyrrolidone in the polymerase chain reaction reverses the inhibitory effects of polyphenolic contamination of RNA . In: Nucleic Acids Research . tape 27 , no. 3 , January 2, 1999, p. 915-916 , doi : 10.1093 / nar / 27.3.915 , PMID 9889293 , PMC 148267 (free full text).
- ↑ CA Kreader: Relief of amplification inhibition in PCR with bovine serum albumin or T4 gene 32 protein. In: Applied and environmental microbiology. Volume 62, Number 3, March 1996, pp. 1102-1106, PMID 8975603 , PMC 167874 (free full text).
- ↑ SJ Bourlat, C. Nielsen, AE Lockyer, D. Timothy, J. Littlewood, MJ Telford: Xenoturbella is a deuterostome that eats molluscs in Nature. Vol. 424, 2003, pp. 925-928, nature.com
- ↑ M. Pruvost, T. Grange, EM Geigl: Minimizing DNA contamination by using UNG-coupled quantitative real-time PCR on degraded DNA samples: application to ancient DNA studies. In: Biotechniques (2005), Volume 38 (4), pp. 569-75. PMID 15884675 . PDF ( Memento of the original from March 4, 2016 in the Internet Archive ) Info: The archive link was inserted automatically and has not yet been checked. Please check the original and archive link according to the instructions and then remove this notice. .
- ↑ DJ Bacich, KM Sobek, JL Cummings, AA Atwood, DS O'Keefe: False negative results from using common PCR reagents. In: BMC Res Notes (2011), Volume 4, p. 457. PMID 22032271 ; PMC 3219698 (free full text).
- ^ Wayne M. Barnes, Katherine R. Rowlyk: Magnesium precipitate hot start method for PCR . In: Molecular and Cellular Probes . tape 16 , no. 3 , June 2002, p. 167-171 , doi : 10.1006 / mcpr.2002.0407 , PMID 12219733 .
- ↑ N. Paul, J. Shum, T. Le: Hot start PCR . In: Methods Mol Biol. (2010), Vol. 630, pp. 301-18. PMID 20301005 .
- ^ MF Kramer, DM Coen: Enzymatic amplification of DNA by PCR: standard procedures and optimization . In: Curr Protoc Immunol. (2001), Chapter 10, Unit 10.20. PMID 18432685 .
- ↑ H. FRAENKEL-CONRAT, BA BRANDON, HS OLCOTT: The reaction of formaldehyde with proteins; participation of indole groups; gramicidin. In: The Journal of biological chemistry. Volume 168, Number 1, April 1947, ISSN 0021-9258 , pp. 99-118, PMID 20291066 .
- ↑ H. FRAENKEL-CONRAT, HS OLCOTT: The reaction of formaldehyde with proteins; cross-linking between amino and primary amide or guanidyl groups. In: Journal of the American Chemical Society. Volume 70, Number 8, August 1948, ISSN 0002-7863 , pp. 2673-2684, PMID 18876976 .
- ↑ H. FRAENKEL-CONRAT, HS OLCOTT: Reaction of formaldehyde with proteins; cross-linking of amino groups with phenol, imidazole, or indole groups. In: The Journal of biological chemistry. Volume 174, Number 3, July 1948, ISSN 0021-9258 , pp. 827-843, PMID 18871242 .
- ^ Q. Chou, M. Russell, DE Birch, J. Raymond, W. Bloch: Prevention of pre-PCR mis-priming and primer dimerization improves low-copy-number amplifications. In: Nucleic Acids Res. (1992), Vol. 20 (7), pp. 1717-23. PMID 1579465 ; PMC 312262 (free full text).
- ↑ S. Tabor, CC Richardson: A single residue in DNA polymerases of the Escherichia coli DNA polymerase I family is critical for distinguishing between deoxy- and dideoxyribonucleotides. In: Proc Natl Acad Sci U.S.A. (1995), Vol. 92 (14), pp. 6339-43. PMID 7603992 ; PMC 41513 (free full text).
- ↑ JM Prober, GL Trainor, RJ Dam, FW Hobbs, CW Robertson, RJ Zagursky, AJ Cocuzza, MA Jensen, K. Baumeister: A system for rapid DNA sequencing with fluorescent chain-terminating dideoxynucleotides. In: Science (1987), vol. 238 (4825), pp. 336-41. PMID 2443975 .
- ↑ B. Fuchs, K. Zhang, MG Rock, ME Bolander, G. Sarkar: High temperature cDNA synthesis by AMV reverse transcriptase improves the specificity of PCR. In: Molecular biotechnology. Volume 12, Number 3, October 1999, pp. 237-240, doi : 10.1385 / MB: 12: 3: 237 , PMID 10631680 .
- ↑ AM Carothers, G. Urlaub, J. Mucha, D. Grunberger, LA Chasin: Point mutation analysis in a mammalian gene: rapid preparation of total RNA, PCR amplification of cDNA, and Taq sequencing by a novel method. In: Biotechniques (1989), Volume 7 (5), pp. 494-6, 498-9. PMID 2483818 .
- ↑ TW Myers, DH Gelfand: Reverse transcription and DNA amplification by a Thermus thermophilus DNA polymerase. In: Biochemistry (1991), Vol. 30 (31), pp. 7661-6. PMID 1714296 .
- ↑ MJ Moser, RA DiFrancesco, K. Gowda, AJ Klingele, DR Sugar, S. Stocki, DA Mead, TW Schoenfeld: Thermostable DNA polymerase from a viral metagenome is a potent RT-PCR enzyme. In: PLoS One (2012), Volume 7 (6), p. E38371. doi: 10.1371 / journal.pone.0038371 . PMID 22675552 ; PMC 3366922 (free full text).