Cell wall
Parent |
Cell periphery |
Subordinate |
Cell wall in bacteria / fungi / plants / spores Microfibrils Lignin / suberin / hemicellulose network Endosporal envelope Exosporium |
Gene Ontology |
---|
QuickGO |
A cell wall is a shell made of polymers that surrounds the cells of plants , bacteria , fungi , algae and some archaea . Animals and protozoa do not have cell walls. The cell wall lies outside the cell membrane , which in turn contains the cell interior. It is formed as a product of the separation of living cells. The cell wall provides structure and protection and also acts as a filter. A main function of the cell wall is to act as a pressure vessel; it prevents the cell from bursting if water should penetrate due to the osmotic gradient .
properties
The cell walls of different organisms serve similar purposes. The wall gives the cells rigidity and strength, which provides protection against mechanical stress. It enables multicellular organisms to develop and maintain a form ( morphogenesis ). The cell wall also limits the entry of large, potentially toxic molecules. It also allows an osmotically stable milieu to be created, since excessive water absorption from the environment is prevented, which would result in the cell bursting, and the cell wall can store water. The composition, properties and shape of the cell wall can change during the cell cycle and are dependent on growth conditions.
Strength of the cell walls
The strength of the cell walls is often overestimated. In most cells, the cell wall is flexible, it is more likely to bend than to hold a certain shape, but it has considerable tensile strength. The stability arises from the interplay between turgor and tensile strength of the cell wall. As soon as the turgor subsides due to a lack of water (wither), leaves and stems begin to hang in unwooded (non-lignified) plants. According to John Howland, a good comparison for the cell wall is a wicker basket in which a balloon is inflated (the plasma membrane), which exerts pressure from within (the turgor). Such a basket is very strong and resistant to mechanical damage.
Plant cell walls
Plant cell walls have two main functions: On the one hand, they serve as a shaping element for the cell and ensure stability. In addition, the cell wall withstands the turgor pressure, which presses the protoplast against the cell wall at around 0.5–1 MPa . They completely enclose the cell and protect it. Plant cell walls consist of cellulose fibrils that are bound into a matrix of pectins , hemicelluloses , proteins and, in some cases, lignin . The individual cells are connected by plasmodesmata through the cell walls .
The entirety of all cell walls and the spaces between cells is called apoplast and is created by the release of substances from the living part of the cell.
Structure of the plant cell wall
from the outside to the inside:
- Middle lamella
- Primary wall
- Secondary wall
- Tertiary wall
Middle lamella
The middle lamella consists largely of pectins, which are stored in the still liquid cell plate in the area of the phragmoplast . It has a gel-like character and is of low expansion.
Primary wall
The primary wall consists of pectins, cellulose, hemicellulose and proteins (especially glycoproteins such as extensins ). After cell division, cellulose is deposited in the form of microfibrils on the middle lamella and a primary wall is formed. The fibrils do not form a structure. The primary wall is therefore elastic, which means that the plant cell is not restricted in its growth. In Kollenchymgewebe it comes to partial thickening of the primary wall. However, the cell remains viable.
Cellulose is represented in the primary (and still growing) cell wall with a proportion of 8-14%. It is in the form of microfibrils that are arranged in a tangled mess (as a scattering texture). These fibrils are embedded in a matrix that mainly consists of hemicellulose and pectin. The most common hemicellulose in the primary cell wall is xyloglucan. The hemicellulose strands are connected to several cellulose fibrils, and the cellulose fibrils are connected to one another, both via hydrogen bonds. This creates a network. In this, the remaining components are integrated as a sub-structure via Ca 2+ - and Mg 2+ - ion crosslinked pectins and isodityrosine bridges crosslinked extensins. The gel-like matrix polymers and the framework-forming cellulose fibrils embedded therein lead to a very tear-resistant and nevertheless plastically deformable composite material. The network can also be converted due to the reversibility of the crosslinking hydrogen bridge bonds.
The structure of the cell walls of the grasses ( Poales ) deviates massively from the one described here, xyloglucan and pectin occur less frequently and are partly replaced by glucuronarabinoxylan, a hemicellulose. As a type II cell wall, they are in contrast to the type I cell wall that occurs in typical dicotyledons and most monocots and gymnosperms.
During growth, the primary cell wall expands after acidification by auxin through a turgor-driven movement of the solid cellulose microfibrils within the weaker hemicellulose / pectin matrix, catalyzed by expansins. The final stable state of the primary cell wall is known as the saccoderm .
Secondary wall
The secondary wall is only formed when the cell has finished growing. It consists largely of tightly packed cellulose microfibrils and hemicelluloses, which are packed in mineral substances and especially in lignins . The microfibrils are placed parallel to one another. Several layers cross each other. In the secondary wall there are recesses ( pits ) that allow the connection between individual cells.
If the secondary wall becomes lignified , the cell dies (formation of sclerenchyma ). Secondary cell walls are typical of the xylem . There are also secondary sealing wall layers, e.g. B. in epidermal cells, the cuticula , which prevent the plant from drying out. They contain cutin , waxes or, in the case of cork , suberin .
Secondary cell walls contain a wide range of additional compounds that change their mechanical properties and permeability. The walls of the cork cells in the bark of trees are impregnated with suberin, and the outer part of the primary cell wall of the plant epidermis is usually impregnated with cutin and wax and forms a permeability barrier . Suberine also forms the permeability barrier in primary roots known as the Casparian streak. Secondary walls - especially in grasses - can also contain microscopic silicon dioxide crystals that strengthen the wall and protect it from herbivores.
The cell walls of some plant tissues also function as stores for carbohydrates, which can be monomerized and absorbed again to support metabolism and growth. For example, the cell walls of the endosperm in the seeds of cereals and grasses are rich in glucans and other polysaccharides , which can be easily broken down by enzymes into simple sugars during the germination of the seeds to nourish the growing embryo. Cellulose microfibrils, on the other hand, cannot easily be digested by plants.
The main polymers that make up wood (predominantly secondary cell wall) are:
- Cellulose, 35-50%
- Xylan, 20-35%, a hemicellulose
- Lignin, 10–25%, a complex phenolic polymer that fills gaps in the cell wall between cellulose, hemicellulose and pectin, making them more hydrophobic and stronger
Tertiary wall
The innermost layer is also called the "tertiary cell wall". It is thin but particularly resistant and covers the inside of the cell wall; it has a warty surface and is rich in pectins and hemicelluloses. It differs from the secondary wall in composition and texture.
Development of the plant cell wall
New cell walls are only formed during cell division. The phragmoplast is first created in the equatorial plane and then the cell wall plate. This is created by the confluence of many Golgi vesicles and remains as a central lamella after the cell wall has been completed. Microfibrils are now randomly deposited on both sides in a scattering texture and thus form the primary walls. The individual fibrils are connected to one another via hydrogen bonds . Since the cell is still growing, the cell wall grows in area. The ability of the cell wall to stretch is due to the structure of the fibrils. The stretching results in the wall thinning, which is compensated for by the application of further fibrils. The growth of the primary wall ends with the maximum expansion of the cell. After the surface growth of the cell wall, the growth in thickness now begins. Microfibrils are applied in parallel and in layers, with the fibrils of adjacent layers usually crossing each other (parallel texture). The resulting secondary wall makes up the majority of the cell wall and gives it stability. However, like the primary wall, it is not expandable. Towards the end of the growth of the cell wall, a final layer, the final lamella or tertiary wall, is applied. This consists of hemicellulose and protopectin.
Chemical composition
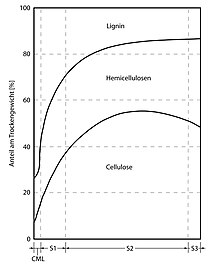
The carbohydrates cellulose, hemicellulose and pectin are most involved in the structure of the plant cell wall. Their proportions differ greatly in some cases. These three substances are fractions , so they include many different polysaccharides. Essentially, they only consist of seven different, glycosidically linked monosaccharides: D- glucose, D- galactose, D- galacturonic acid, L- rhamnose, L- fucose, D- xylose, L -arabinose. Other substances such as lignin and suberin can be added, and polypeptides also make up a small proportion (5–10%).
The cell wall consists mainly of the following groups of substances:
pectin
Pectins can be removed from the cell wall with relatively mild media, e.g. B. with hot water and complexing agents or with potassium chlorate and nitric acid (Schulze's mixture) or after the action of specific enzymes (pectinases). The cell walls containing cellulose resist this treatment, but not the middle lamella containing pectin, so that the cells also separate from each other (maceration). From a chemical point of view, pectin is a heterogeneous fraction, after an extraction one can distinguish between: homogalacturonans (1,4-α- D- galacturonans, polygalacturonic acid), rhamnogalacturonans (branched mixed polymers of galacturonic acid and rhamnose with various additional sugar residues), arabinans (1,5-α- L -arabinosyl chains) and galactans (1,4-β- D -galactosyl chains). The carboxy groups of pectin are linked by Ca 2+ and Mg 2+ via salt bridges. However, some carboxy groups exist as esters with methanol and can therefore no longer form salt bridges. These salt bridges are relatively reversible as a bond, so that pectin is elastic and easily changeable. A distinction is made between protopectin, which makes up the largest part of the middle lamella and mainly consists of galacturonic acid and rhamnose, and pectin, which consists of highly methylated galacturonan and occurs in large quantities in the cell walls of many fruits.
Hemicellulose
Hemicelluloses can be removed from the cell wall by an alkaline treatment. Its name comes from the fact that it was previously mistaken for an intermediate product in cellulose synthesis. Hemicellulose makes up the majority of the matrix in the primary wall. Like pectin, hemicelluloses are a heterogeneous mixture of different polysaccharides, the composition of which can vary greatly. The main components are usually xyloglucans, but arabinogalactans and glucomannans are also found. They are composed of pentoses such as D -xylose and L -arabinose and hexoses such as D -glucose, D- mannose and D- galactose.
Cellulose
Cellulose is a linear, unbranched polymer made from β- D -glucose monomers. The cellulose content is approx. 10% in primary walls and can be over 90% in secondary walls. Cellulose molecules exist in parallel bundles, the elementary fibrils (or micellar strands), which are organized into microfibrils, which in turn can be bundled into macrofibrils. The fibrils are held tightly together by hydrogen bonds and can have a high proportion of paracrystalline regions. The paracrystallinity gives the fibrils a tear strength that is comparable to that of steel. Also due to the paracrystallinity, unlike matrix material, they are hardly hydrated. In the primary wall, the cellulose fibrils are randomly arranged in the plane, this is called scattering texture. In the secondary wall, on the other hand, the fibrils are applied in layers, parallel and slightly rotated in alignment with the next layer. This is called a parallel texture.
Cellulose is insoluble in water and thus cannot be synthesized in the Golgi apparatus and transported into the cell wall through vesicles, such as pectins, hemicelluloses and cell wall proteins. Instead, cellulose is synthesized directly as elementary fibrils in the cell wall by the enzyme complex cellulose synthase. Cellulose synthase is a transmembrane protein that occurs as a hexameric rosette complex, mostly in rosette fields. The glucose required for synthesis is supplied in the form of uridine diphosphoglucose (UDPG) in the cytoplasm. The alignment of the cellulose fibrils, important for cell growth and differentiation, is carried out by the cytoskeleton, on which the cellulose synthase moves as if on rails. Plants themselves cannot break down cellulose, but herbivores and some fungi have the necessary enzyme cellulase . Technically, cellulose is broken down into glucose again by sulfuric acid (wood saccharification).
Cell wall proteins
In addition to numerous enzymes, such as B. hydrolases , esterases , peroxidases and transglycosylases, which are involved in the construction and remodeling of the cell wall (especially in primary walls), make structural proteins (1–5%) the main part of cell wall proteins. A distinction is made between glycine-rich proteins (GRP), proline-rich proteins (PRP), arabinogalactan proteins (AGP) and hydroxyproline-rich glycoproteins (HRGP). The hydroxyproline-rich glycoproteins (HRGP) are arguably the most widely used and best studied. Each class of glycoproteins is defined by a characteristic, highly repetitive protein sequence. Most are glycosylated , contain hydroxyproline (Hyp) and are networked in the cell wall. [5] The relative proportions of carbohydrates, secondary compounds and proteins varies between different plants, cell types and ages.
Permeability
The primary cell wall of most plant cells is semi-permeable and allows the passage of small molecules and proteins; by gel permeation chromatography the maximum size was estimated to be 30-60 kDa. Above all, water and carbon dioxide are distributed throughout the plant from cell wall to cell wall by apoplastic transport. The pH value is an important factor in the transport of molecules through cell walls.
Bacterial cell walls
The bacterial cell wall separates the actual cell with its surrounding cell membrane from the environment. On the one hand, the cell wall is robust enough to maintain the cell geometry in order to serve as protection against adverse environmental conditions. On the other hand, it is also flexible enough not to hinder cell growth, cell division and transport processes in and out of the cell.
The high concentration of soluble substances in the cytoplasm creates an osmotic pressure of up to 1.5 MPa in the cell , which has to be compensated by the cell wall.
In addition, the cell wall serves to protect against phages and, in the case of pathogenic bacteria, against the immune system of their hosts and has to withstand aggressive metabolites of competing microorganisms.
With the so-called Gram stain, bacteria can be roughly classified according to their cell wall structure. The gentian violet dye cannot be washed out in gram-positive bacteria due to their multi-layered cell wall, therefore these bacteria appear blue, whereas the gram-negative bacteria with very thin cell walls are not colored at all due to the washing out of the dye. These can then be colored red with the fuchsine dye. Gram-positive and gram-negative bacteria differ in the structure of their cell walls. In the case of gram-positive ones, it consists of many layers of the so-called murein ( peptidoglycan ), in which (lipo) teichoic acids and proteins are stored.
In Gram-negative bacteria, the cell membrane (inner membrane) is only covered by a thin peptidoglycan layer on which a second, outer cell membrane is deposited, which differs in chemistry and structure from the inner cell membrane. This outer membrane is traversed by proteins such as porins , and it has lipopolysaccharides (LPS) on the outside , which is why it is also known as the lipopolysaccharide layer. The lipid A of the LPS can act as an endotoxin and is a virulence factor of pathogenic bacteria.
Depending on the species, additional protein layers (see S-layer for Archaea), capsules or mucus layers are formed.
Cell walls in fungi
The cell walls that surround the cells of some fungi are made of chitin , which is also used to build the exoskeleton of insects . Similar to plants, the cell walls also serve to stiffen the cells so that they can keep their shape.
literature
- Andreas Bresinsky , Christian Körner , Joachim W. Kadereit , Gunther Neuhaus , Uwe Sonnewald : Strasburger - textbook of botany. Founded by E. Strasburger. Spektrum Akademischer Verlag, Heidelberg 2008 (36th edition) ISBN 978-3827414557
- Biology, Neil A. Campbell / Jane B. Reece, Spectrum Academic Publisher
Web links
- www.biologie.uni-hamburg.de
- Max Planck Society: Enzymes for cell wall synthesis are conserved across species boundaries , July 11, 2011
Individual evidence
- ^ John L. Howland: The Surprising Archaea: Discovering Another Domain of Life . Oxford University Press, Oxford 2000, ISBN 0-19-511183-4 , pp. 69-71.
- ↑ Strasburger, p. 91.
- ↑ a b c d e Weiler, Elmar; Lutz Nover (2008): General and molecular botany , Thieme Verlag. Pp. 94-98.
- ↑ a b c d e f Peter Schopfer; Axel Brennicke (2006): Plant Physiology , Elsevier, Munich 2006, ISBN 978-3-8274-1561-5 . Pp. 23-30.
- ↑ Weiler, Elmar; Lutz Nover (2008): General and molecular botany , Thieme Verlag. P. 240
- ↑ Peter Schopfer; Axel Brennicke (2006): Plant Physiology , Elsevier, Munich 2006, ISBN 978-3-8274-1561-5 , p. 240
- ↑ Laurence Moire, Alain Schmutz, Antony Buchala, Bin Yan, Ruth E. Stark, and Ulrich Ryser: Glycerol Is a Suberin Monomer. New Experimental Evidence for an Old Hypothesis . In: Plant Physiol . 119, No. 3, 1999, pp. 1137-1146. doi : 10.1104 / pp.119.3.1137 . PMID 10069853 . PMC 32096 (free full text).
- ↑ C. Michael Hogan. 2010. Abiotic factor . Encyclopedia of Earth. eds Emily Monosson and C. Cleveland. National Council for Science and the Environment . Washington DC.