Electrophilic aromatic substitution
An electrophilic aromatic substitution - abbreviated as S E Ar - is an electrophilic substitution reaction on an aromatic compound. While substitutions are often nucleophilic in aliphatics , aromatics, due to their electron-rich π system, are preferentially attacked by electrophiles . As a rule, a hydrogen atom bound to the reacting aromatic is replaced by the electrophile. Electrophilic aromatic substitution is a multi-step reaction.
Reaction mechanism
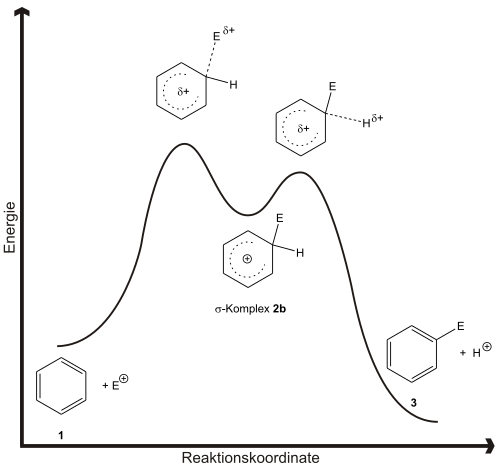
The reaction mechanism in Figure 1 describes the general course of an electrophilic aromatic substitution. The aromatic 1 interacts with the electrophile E + . One speaks of the formation of the π-complex 2a . From this, or directly from the starting materials, the mesomerism-stabilized σ-complex 2b , which is also known as the arenium ion or Wheland complex , is formed with the removal of the aromaticity of 1 . With deprotonation of this complex, a rearomatization of the system takes place and the end product 3 is released. The formation of a π complex is not absolutely necessary to explain the reaction.
Electrophilic
A wide spectrum of compounds can be used as the electrophile E + , which are often reactive products of a reaction preceding the electrophilic aromatic substitution. In the context of electrophilic substitution, the following electrophiles are important:
- the proton
- polarized halogens , e.g. B. bromination or iodination :
- Br 2 → Br + + Br - with the action of a Lewis acid as a catalyst
- Hypobromous acid HBrO (structure HO-Br)
- Iodine chloride I-Cl
- Carbocations
- Carbonyl compounds (C = O → C δ + = O δ− ) under the action of a Lewis acid as a catalyst, an acyl cation is formed
- Sulfur trioxide in sulfuric acid forms HSO 3 +
- the nitrosyl cation
- the nitryl or nitronium cation ( nitration ) from [NO 2 ] + [BF 4 ] - or by protolysis of nitric acid
Some of the electrophiles are positively charged and can directly replace the leaving group. For others, the positive charge has to be generated through bond cleavage. The coordination of a Lewis acid to the negative end of the electrophile increases the polarization of the bond and accelerates the electrophilic substitution. This happens e.g. B. in the bromination with bromine , Br 2 , in the presence of iron (III) bromide , FeBr 3 .
The leaving group
As a rule, the leaving group is a proton. Examples of carbocations, sulfonyls and silyl groups are also known.
Kinetics of reaction
Fig. 2 shows the reaction coordinate of the reaction. There is a lot of evidence for the two-stage approach. On the one hand, it is possible to isolate and characterize the arenium ions 2b occurring as an intermediate product in pure substance. The σ-complex 4 of mesitylene and ethyl fluoride can be prepared in the presence of boron trifluoride in ether at −80 ° C. On the other hand, by examining isotope effects, one can determine the kinetics of the reaction, which suggests a two-step mechanism. Then the formation of the σ-complex is the rate-determining step.
Substituent Effects
Substituents already present on the aromatic have a great influence both on the reactivity of the aromatic and thus on the speed of the electrophilic substitution, as well as on the position of a second substitution (directing effects). If the electron density of the aromatic ring is increased, its reactivity towards electrophiles also increases. This increase can be caused by mesomeric and / or inductive effects - one speaks of the M or I effect . An attached sign indicates whether the substituent increases the electron density of the ring (+ M / + I effect) or decreases it (−M / −I effect).
The inductive effect is based on the fact that electron-withdrawing substituents destabilize the protonated ring. The mesomeric effect is based on the fact that the substituent has free electron pairs via which it can increase or decrease the electron density through mesomerism in the ring.
Conducting effect
In addition to influencing the reactivity of the aromatic, the first substituent has a directing effect on the entry position of the second substituent: Electrophilic aromatic substitution reactions with + I / + M substituents give predominantly ortho and para substituted, –I / –M substituents, on the other hand, meta - substituted products.
To justify this effect, consider the mesomeric boundary structures of either the aromatic reactant or the σ-complex. The height of the first transition state between the starting material and the σ-complex is actually decisive for the preferred second substitution. When considering the mesomeric boundary structures of the educt, one argues that the electrophile attacks the electron-rich atoms of the ring more easily and that the transition state is lower. When considering the σ-complex, it is assumed that a more stable, i.e. H. low-energy σ-complex was also reached via a lower transition state. In general terms, one can say that electron donating groups - that is, electron donor groups - such as -CH 3 or -OCH 3 ortho / para -directing, while electron-attracting groups - so-called electron acceptors - such as -NO 2 or -CO 2 H meta -directing.
First substitute | M-effect | I effect | Conducting effect | Activating effect |
---|---|---|---|---|
–O - | + | + | ortho / para | activating strong |
-OH / -NH 2 / -NR 2 | + | - | ortho / para | activating strong |
-OCH 3 / -OR / -NHCOR | + | - | ortho / para | activating medium |
Alkyl radical | n / A | + | ortho / para | activating weak |
-F / -Cl / -Br / -I | + | - | ortho / para | deactivating weak |
-CN / -COOH / -COOR / -COH / -COR | - | - | meta | deactivating medium |
-NO 2 / -NR 3 + / -CF 3 / -CCl 3 | - (only -NO 2 ) | - | meta | deactivating strong |
Second substitution
The following examples show various options for secondary substitution:
Nitrotoluene
In the nitration of toluene with nitric acid , the + I effect of the methyl group is decisive for controlling the second substituent, so that the main products are o -nitrotoluene with 65% and p -nitrotoluene with 30%, whereas m -nitrotoluene is only 5%.
Dinitrobenzene
Here, the −I effect and the −M effect of the nitro group of nitrobenzene cause 93% of the control into the meta position. The ortho and para positions occur only 6 and 1% respectively.
Polysubstitution
In the case of an electrophilic aromatic substitution with two or more already present substituents, the reactivity and the location of the substitution can usually be derived from the combined effects of the substituents. This is often very easy if the effects of the substituents are intensified with one another or all free positions are equivalent. If directing effects of different substituents resulted in substitution at different sites, it is usually the most activating substituent that determines where the substitution occurs. If two positions are similarly preferred by + I effects of alkyl substituents, steric effects gain in importance and the substitution takes place at the more accessible position.
The figure shows the nitration of nitrotoluene to dinitrotoluene . First forming a π-complex between the nitronium ion NO 2 + and the aromatics, then they react to a as a σ-complex called carbonium ion , whose resonance structures are shown in square brackets. This intermediate product is less stable in the lower reaction path than in the unsubstituted aromatic, since the positive charge is close to the electron-withdrawing nitro group. The above intermediate is stabilized because the positive charge is close to the electron donating methyl group. The top product is formed preferentially.
Electrophilic substitution reactions on aromatics (selection)
- Aminomethylation
- Azo coupling
- Blanc reaction (chloromethylation)
- Friedel-Crafts acylation
- Friedel-Crafts alkylation
- Halogenation
- Houben-Hoesch synthesis
- Hydroxymethylation
- Gattermann synthesis
- Gattermann-Koch synthesis
- Kolbe-Schmitt reaction
- nitration
- Sulfonation
- Vilsmeier-Haack reaction
- Diazotization
Individual evidence
- ^ Hans Beyer and Wolfgang Walter : Textbook of Organic Chemistry , 19th edition, S. Hirzel Verlag, Stuttgart 1981, ISBN 3-7776-0356-2 , p. 456.
- ↑ Joachim Buddrus: Fundamentals of Organic Chemistry , 3rd edition, Walter de Gruyter, 2003, ISBN 3-11-014683-5 , p. 360.
- ^ FA Carey, Organic Chemistry 4th Edition, McGraw-Hill, 2000, ISBN 0-07-117499-0 .