Graphene oxide
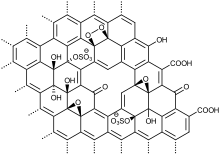
Graphene oxide (GO) is a carbon-based 2D nanomaterial that is usually produced by reacting graphite with a strong oxidizing agent and then processing it in water. It is made up of an extensive hexagonal carbon skeleton, which has a variable amount of point and extensive hole defects within the carbon plane. This carbon lattice is decorated with oxygen-containing functional groups on both sides and the edge. This material is a very promising starting material for the mass production of graphene . The name graphene oxide is the basic term for all monolayer graphene derivatives with oxygen-containing functional groups, while multilayer graphene oxide is referred to as graphite oxide .
Manufacturing
For historical reasons, there is no uniform protocol for the production of graphene oxide from graphite . Although the underlying reactions for the production of " graphitic acid " (Brodie 1859, Staudenmaier 1898, Charpy 1909 and Hofmann 1937) have been known for many decades, the laminar nature and thus the possible exfoliation of graphite oxide to graphene oxide was only discovered much later. The currently most widely used method of representation is based on the method of William S. Hummers and Richard E. Offeman (1958), as this is easy to perform and relatively harmless chemicals are used. Depending on the method, the yield, the degree of functionalization of the sp 2 lattice, the number of hole defects introduced and thus the final quality of the graphene oxide obtained vary greatly.
To obtain it, finely ground graphite with high crystallinity is generally first dispersed in a highly concentrated and oxidizing acid. Usually it is sulfuric acid , nitric acid , or mixtures of these - in rare cases orthophosphoric acid . The selection of the right graphite is important here, as it has a decisive influence on the quality of the graphene oxide obtained in the end. Then, with constant stirring, partly with cooling, another oxidizing agent is carefully added (e.g. KMnO 4 , KClO 3 , (NH 4 ) 2 S 2 O 8 , NaNO 3 ). In the case of permanganate (Hummers and Offeman method), the reactive species is the manganyl cation (MnO 3 + ) or dimanganese heptoxide formed in situ as a result of its dehydration .
The oxidizing agent begins to oxidize the individual graphene layers up to a theoretical charge maximum of C 30 + , with sulfuric acid and hydrogen sulfate anions intercalating between the graphene layers at the same time to balance the charge . An acceptor-type graphite intercalation compound is created as an intermediate (cf. lithium-ion battery, donor-type ). The intercalation process increases the intercalation process from 0.332 nm in graphite to ~ 0.9 nm in the intercalation compound. Subsequent aqueous work-up leads to the formation of graphite oxide . The exact mechanism of functionalization is complex and, despite intensive research, has not yet been finally clarified in detail. The graphite oxide finally obtained can delaminate by itself in graphene oxide even in neutral or slightly alkaline aqueous solutions with a sufficiently high degree of functionalization. However, this slow process can be accelerated by ultrasound , ball mills or jet cavitation . After further purification steps - usually by centrifugation or filtration - a golden yellow dispersion of graphene oxide in water is obtained. A previous addition of hydrogen peroxide serves to transform various insoluble manganese species into water-soluble Mn 2+ ions and thus facilitates purification.
structure
The superordinate structure of graphene oxide is graphite oxide , from which it is delaminated with e.g. B. ultrasound or ball mills is obtained. The particle size of the individual graphene oxide particles in a dispersion is heterogeneously distributed and depends heavily on the graphite initially used. After production, it is usually in the range of a few micrometers, usually in the range of 5-20 μm, and can be reduced afterwards using various post-treatment techniques.
A monolayer graphene oxide consists of a hexagonal carbon structure and is decorated on both sides with oxygen-containing functional groups ( on-plane functional groups ) that were introduced through oxidation of the graphite. Depending on the synthesis protocol, the composition of the functional groups varies dramatically, but can be limited to the following types: hydroxyls (-OH), epoxides (> O), sulfuric acid esters (-OSO 3 H), and endoperoxides (> O 2 ). The long-range order of the hexagonal basic structure is disturbed by isolated point defects ( vacancy defects ), non-hexagonal structural motifs (e.g. Stone-Wales defects ) and extensive holes that were either initially present in the graphite or later arise through overoxidation during synthesis (formation of CO / CO 2 ). Additional carbonyls (-C = O), carboxyls (-CO 2 H) can be present at these points and represent so-called in-plane functional groups . Images with the atomic force microscope show that the carbon layers are deformed by oxygen bonds, as well as a significantly increased roughness have. These defects also appear in the Raman spectrum of graphene oxide. Since the oxidation usually only leads to a degree of functionalization of approx. ~ 60-70%, there are still partially isolated or conjugated double bonds and aromatic areas between the functional groups. Depending on the synthesis route chosen, there is a smooth transition from intact graphene with oxo functionalities to increasingly holey graphene with oxo functionalities to a material with an almost completely destroyed carbon lattice with a humic acid- like structure.
Theoretical calculations and experiments show that the initially homogeneously distributed functional groups of a freshly synthesized graphene oxide rearrange themselves to sp² and sp³ dominated areas due to aging processes. This process is e.g. B. accelerated by acids.
Graphene oxide as a solid can be produced by freeze-drying, but because of its high density of polar functional groups it always has a small amount of strongly bound water molecules (<10 m%).
Applications
Graphene production
In the 2000s, graphite oxide became interesting as a possible precursor for the production of graphene on a large scale. Due to its hydrophilic properties, graphite oxide dissolves easily in water, breaking down into microscopic graphene oxide flakes, which are usually one layer thick. By means of chemical reduction it is possible to obtain a metastable suspension of graphene flakes, which, however, has a strong tendency to aggregate. However, this can largely be prevented by adding surfactants beforehand.
A partial to complete reduction of the graphene oxide can be achieved in many different efficient ways. Sodium borohydride , glutathione , ascorbic acid (vitamin C), hydrazine (24h at 100 ° C) or hydrogen iodide are used for chemical reduction . Further, a reduction is also short-term, lasting a few seconds treatment with plasma of hydrogen gas, a strong light pulse, such as a Xenon - flash possible or UV radiation. The reducing effect of UV light can be increased enormously by mixing acetone and isopropanol in water (1% or 2%). The method of "reduction" often described in the literature by the action of sodium hydroxide or potassium hydroxide solution or treatment at high temperatures is based on a disproportionation reaction and the associated partial destruction of the carbon lattice by CO / CO 2 formation.
Compared to the other conventional methods of graphene production, such as mechanical exfoliation or chemical vapor deposition ( CVD process ), production on a gram or kilogram scale is easily possible and not limited to individual carbon films.
Graphene oxide paper
As in papermaking , graphene oxide flakes can be scooped out of an aqueous dispersion, resulting in extremely tear-resistant graphene oxide paper.
Chemical properties
stability
Graphene oxide is a material that is not very temperature-resistant and already decomposes at ~ 100 ° C to graphene and CO / CO2 with a high number of hole defects. The on-plane functional groups can by means of many reducing substances such. B. sodium borohydride , glutathione , ascorbic acid (vitamin C), hydrazine or hydrogen iodide can be removed. The efficiency with which the oxygen-containing functional groups can be removed differs immensely and is not always quantitative. Many other methods such as B. by hydrogen plasma , strong light pulses or UV radiation are also known. The method of "reduction" by the action of sodium hydroxide or potassium hydroxide solution, which is often described in the literature, leads to a partial destruction of the carbon lattice with formation of CO / CO 2 during treatment at high temperatures (so-called thermally reduced graphene oxides ) however, the other part is rearomatized into graphs. For this reason, it is better to speak of thermal disproportionation than thermal reduction.
The carbon structure of graphene oxide is stable to acids and short-term contact with bases at low temperatures ( HCl , H 2 SO 4 , NaOH , KOH ). A shorter contact time with bases of a few hours at room temperature or several hours at 4 ° C already leads to measurable degradation. The action of dilute acids and bases can change the type and number of functional groups. Sulfuric acid esters , which are often present during the synthesis after the cold work-up of graphite oxide, are split by acids and bases. Concentrated acids with oxidizable anions (e.g. HCl ) lead to a partial reduction, while strong bases lead to an apparent reduction (more precisely: disproportionation of GO to partially reduced GO and: CO / CO 2 ).
Changes to the material can largely be prevented when stored below 10 ° C. Nevertheless, theoretical computer calculations and experimental results show that even under these conditions functional groups are not necessarily stably bound to the carbon lattice, but can nevertheless rearrange themselves. In the presence of multivalent cations , graphene oxide tends to agglomerate through complex formation between the individual graphene oxide flakes.
Functionalization
A covalent functionalization of the carbon lattice is possible via aryl radicals via diazonium salts . Modification of groups introduced during the synthesis is possible via reactions with ammonia / amines , sodium azide or isocyanate .
A non-covalent functionalization is possible with alkylamines via electrostatic attraction of negatively charged sulfuric acid esters with the positively charged alkylammonium ions, as well as with surface active surfactants ( sodium dodecyl sulfate , sodium dodecylbenzenesulfonate , sodium cholate , ...). Partially reduced graphene oxide can be stabilized against agglomeration with the help of common surfactants ( SDS , SDBS , sodium cholate ...).
Physical Properties
Particle size
The size of the individual graphene oxide particles can be controlled by post-processing using ultrasound , ball mill , jet cavitation, etc. Depending on the graphite source and delamination technique used, particle sizes from 50 nm to below 20 nm can be produced easily and relatively quickly. Since particle breaking follows the law of power, smaller particles break more slowly than larger ones. It was also shown that the breakage rate depends on the degree and type of functionalization: On the one hand, a graphene oxide with a higher density of functional groups breaks more easily under external stress than a derivative with fewer functional groups. If the graphene oxide contains heavy sulfuric acid esters as functional groups, the fracture rate is increased compared to a graphene xod with exclusively hydroxyl and peroxide groups with the same degree of functionalization.
Dispersibility
Depending on the synthesis and the type and amount of functional groups, graphene oxide is dispersible in many polar solvents such as ethanol , methanol , DMF , NMP , CHP and water. In some cases, an aggregation into multilayers is visible in pure organic solvents, but can be prevented by adding small amounts of water. Via non-covalent functionalization - e.g. B. with alkylamines - a certain dispersibility in less polar solvents such as THF is possible. As the reduction progresses - for example with hydrazine or sodium borohydride - graphene oxide becomes increasingly difficult to disperse in water. This can be completely prevented by adding surfactants.
conductivity
Due to its lower amount of sp 2 -hybridized carbon atoms, graphene oxide is a poorer conductor than graphene, which only has sp 2 carbon atoms . Hole defects, like functional defects, have a great influence on the conductivity, as this disrupts the π-system that mediates conductivity. Graphite oxide or graphene oxide - produced according to the method of Hummers and Offeman - generally behaves like an insulator and has a differential electrical conductivity of 1–5 · 10 −3 S · cm −1 . However, if you apply a bias voltage of 10 V, it is almost a semiconductor . The conductivity of graphene , which is obtained after the reduction of graphene oxide with hydrazine , is often more than 3 orders of magnitude higher and is in the range of 10 S · cm −1 . The mobilities of the electron-hole pairs are 0.5–30 cm 2 · V −1 · s −1 for electrons and 2–200 cm 2 · V −1 · s −1 for the holes . These relatively poor values compared to CVD graphs (~ 7,000 cm 2 · V −1 · s −1 ) and mechanically foiled graphene (~ 10,000 cm 2 · V −1 · s −1 ) are based on the fact that the hexagonal carbon structure is through the oxidation of graphite to graphite oxide, the subsequent exfoliation and reduction of which to graphene is interspersed with a great number of vacancy defects and extensive holes and, if applicable, has not been completely defunctionalized.
In the course of time, wet-chemical methods of representing graphene made of graphene oxide have been optimized so that they have significantly higher values for conductivity (~ 250-2,000 cm 2 / Vs).
Optical properties

The absorption spectrum of a graphene oxide suspension essentially shows three variable areas that change with different degrees of functionalization: a maximum at ~ 235 nm (π → π * transition), a shoulder at ~ 300 nm (n → π * transition) and a tapering one Range at higher wavelengths. The position of the maximum can primarily be used to quickly determine the degree of functionalization (sp³ defects). A bathochromic shift of the maximum as well as the disappearance of the shoulder and the increase in the extinction are an indication of a reduction in the starting material.
Using Raman spectroscopy , various techniques can be used to make functional and vacancy defects visible. The so-called D (Defect), G (Graphite) and 2D bands are compared and evaluated. Samples of the material are usually deposited on SiO 2 / Si surfaces. Then they are bombarded with a monochromatic laser beam and the Stokes or Anti-Stokes scatter are measured. Ideally, perfect graphs have no D band, a thin G band and a 2D band with ~ 1.5 times the intensity. With an increasing number of structural defects, the intensity of the D band increases up to a maximum I D / I G ratio and then decreases again as the functionalization progresses. The maximum value depends on the excitation wavelength of the laser used. The ratio I 2D / I G also decreases sharply with increasing defect density . In addition, the general rule is that the half-width of each band increases with the number of defects. Graphene oxide with a high degree of functionalization (30% -70%) has an I D / I G ratio of ~ 1.0-1.2 (laser: 532 nm), which increases with a decreasing number of functional groups (partial reduction) up to a maximum value.
Individual evidence
- ↑ BC Brodie: Note sur un nouveau procédé pour la purification et la désagrégation du Graphite . Ed .: Ann. Chim. Phys. tape 45 , 1855, p. 351-353 .
- ↑ a b Georges Charpy: Lur Sar Formation de L'oxyde Graphitique et la Définition du Graphite . Ed .: Comptes rendus hebdomadaires des séances de l'Académie des sciences. No. 5 , 1909, pp. 920-923 .
- ^ Ulrich Hofmann, Alfred Frenzel: Swelling of graphite and the formation of graphitic acid . In: Reports of the German Chemical Society (A and B Series) . tape 63 , no. 5 , May 7, 1930, p. 1248-1262 , doi : 10.1002 / cber.19300630543 .
- ^ A b c William S. Hummers, Richard E. Offeman: Preparation of Graphitic Oxide . In: Journal of the American Chemical Society . tape 80 , no. 6 , March 1958, p. 1339-1339 , doi : 10.1021 / ja01539a017 .
- ↑ Hanns Peter Boehm, Werner Scholz: Comparison of the representation process for graphite oxide . tape 691 . Justus Liebig's Annalen der Chemie, 1965, p. 1-8 .
- ^ A b c d Daniel R. Dreyer, Sungjin Park, Christopher W. Bielawski, Rodney S. Ruoff: The chemistry of graphene oxide . In: Chemical Society Reviews . tape 39 , no. 1 , December 14, 2009, p. 228-240 , doi : 10.1039 / b917103g .
- ^ A b c d Siegfried Eigler, Andreas Hirsch: Chemistry with Graphene and Graphene Oxide — Challenges for Synthetic Chemists . In: Angewandte Chemie International Edition . tape 53 , no. 30 , July 21, 2014, p. 7720-7738 , doi : 10.1002 / anie.201402780 .
- ↑ a b c d e f Siegfried Eigler, Stefan Grimm, Michael Enzelberger-Heim, Paul Müller, Andreas Hirsch: Graphene oxide: efficiency of reducing agents . In: Chemical Communications . tape 49 , no. 67 , July 26, 2013, doi : 10.1039 / c3cc43612h .
- ^ BC Brodie: On the Atomic Weight of Graphite. Ed .: Proceedings Proceedings of the Royal Society of London. 1859, p. 11-12 .
- ↑ Ulrich Hofmann Ernst König: Investigations on graphite oxide . Ed .: Journal for Inorganic and General Chemistry. tape 234 , no. 4 , 1937, pp. 311-336 .
- ↑ L. Staudenmaier: Process for the representation of graphitic acid . In: Reports of the German Chemical Society . tape 31 , no. 2 , 1898, p. 1481–1487 , doi : 10.1002 / cber.18980310237 .
- ↑ Patrick Feicht, Renée Siegel, Herbert Thurn, Jens W. Neubauer, Maximilian Seuss: Systematic evaluation of different types of graphene oxide in respect to variations in their in-plane modulus . In: Carbon . tape 114 , April 2017, p. 700-705 , doi : 10.1016 / j.carbon.2016.12.065 .
- ^ A b Siegfried Eigler, Yichen Hu, Yoshitaka Ishii, Andreas Hirsch: Controlled functionalization of graphene oxide with sodium azide . In: Nanoscale . tape 5 , no. 24 , 22nd November 2013, doi : 10.1039 / c3nr04332k , PMID 24162364 , PMC 3880578 (free full text).
- ↑ a b Ayrat M. Dimiev, James M. Tour: Mechanism of Graphene Oxide Formation . In: ACS Nano . tape 8 , no. 3 , March 25, 2014, ISSN 1936-0851 , p. 3060-3068 , doi : 10.1021 / nn500606a .
- ↑ a b W. Rüdorff, U. Hofmann: About graphite salts . In: Journal of Inorganic and General Chemistry . tape 238 , no. 1 , June 24, 1938, p. 1-50 , doi : 10.1002 / zaac.19382380102 .
- ↑ a b Steffen Seiler, Christian E. Halbig, Fabian Grote, Philipp Rietsch, Felix Börrnert: Effect of friction on oxidative graphite intercalation and high-quality graphene formation . In: Nature Communications . tape 9 , no. 1 , December 2018, ISSN 2041-1723 , doi : 10.1038 / s41467-018-03211-1 , PMID 29483555 , PMC 5826935 (free full text).
- ^ A b Siegfried Eigler: Graphite sulphate - a precursor to graphene . In: Chem. Commun. tape 51 , no. 15 , February 5, 2015, p. 3162-3165 , doi : 10.1039 / c4cc09381j .
- ^ A b Siegfried Eigler, Michael Enzelberger-Heim, Stefan Grimm, Philipp Hofmann, Wolfgang Kroener: Wet Chemical Synthesis of Graphene . In: Advanced Materials . tape 25 , no. 26 , July 12, 2013, p. 3583-3587 , doi : 10.1002 / adma.201300155 .
- ↑ a b c Anton Lerf, Heyong Hey, Michael Forster, Jacek Klinowski: Structure of Graphite Oxide Revisited‖ . In: Journal of Physical Chemistry B . tape 102 , no. June 23 , 1998, pp. 4477-4482 , doi : 10.1021 / jp9731821 .
- ↑ a b Patrick Feicht, Siegfried Eigler: Defects in Graphene Oxide as Structural Motifs . In: ChemNanoMat . tape 4 , no. 3 , March 2018, p. 244-252 , doi : 10.1002 / cnma.201700357 .
- ↑ Florian Banhart, Jani Kotakoski, Arkady V. Krasheninnikov: Structural Defects in Graphene . In: ACS Nano . tape 5 , no. 1 , January 25, 2011, ISSN 1936-0851 , p. 26-41 , doi : 10.1021 / nn102598m .
- ↑ Ulrich Hofmann, Alfred Frenzel, E. Csalán: The constitution of graphitic acid and its reactions . In: Justus Liebig's Annals of Chemistry . tape 510 , no. 1 , 1934, p. 1-41 , doi : 10.1002 / jlac.19345100102 .
- ↑ M Mermoux, Y Chabre, A Rousseau: FTIR and 13C NMR study of graphite oxide . In: Carbon . tape 29 , no. 3 , 1991, pp. 469-474 , doi : 10.1016 / 0008-6223 (91) 90216-6 .
- ^ A b Siegfried Eigler, Andreas Hirsch: Chemistry with Graphene and Graphene Oxide — Challenges for Synthetic Chemists . In: Angewandte Chemie International Edition . tape 53 , no. 30 , July 21, 2014, p. 7720-7738 , doi : 10.1002 / anie.201402780 .
- ↑ a b c d e f g C. Gomez-Navarro et al. : Electronic Transport Properties of Individual Chemically Reduced Graphene Oxide Sheets . In: Nano Letters. 7, No. 11, 2007, p. 3499, doi: 10.1021 / nl072090c .
- ↑ Patrick Feicht, Daniel A. Kunz, Anton Lerf, Josef Breu: Facile and scalable one-step production of organically modified graphene oxide by a two-phase extraction . In: Carbon . tape 80 , December 2014, p. 229-234 , doi : 10.1016 / j.carbon.2014.08.061 .
- ↑ H. Pieper, CE Halbig, L. Kovbasyuk, MR Filipovic, S. Eigler: Oxo-Functionalized Graphene as a Cell Membrane Carrier of Nucleic Acid Probes Controlled by Aging . In: Chemistry - A European Journal . tape 22 , no. 43 , October 17, 2016, p. 15389-15395 , doi : 10.1002 / chem.201603063 .
- ↑ AV Talyzin et al. : Nanocarbons by High-Temperature Decomposition of Graphite Oxide at Various Pressures. In: J. Phys. Chem. C. 113, No. 26, 2008, pp. 11279-11284, doi: 10.1021 / jp9016272 .
- ↑ a b S. Stankovich et al. : Stable aqueous dispersions of graphitic nanoplatelets via the reduction of exfoliated graphite oxide in the presence of poly (sodium 4-styrenesulfonate). In: J. Material Chemistry. 16, 2006, p. 155, doi: 10.1039 / b512799h .
- ↑ a b c Laura J. Cote, Rodolfo Cruz-Silva, Jiaxing Huang: Flash Reduction and Patterning of Graphite Oxide and Its Polymer Composite . In: Journal of the American Chemical Society. 131, 2009, pp. 11027-11032, doi: 10.1021 / ja902348k .
- ↑ a b Roman Flyunt, Wolfgang Knolle, Axel Kahnt, Christian E. Halbig, Andriy Lotnyk: High quality reduced graphene oxide flakes by almost kinetically controlled and clean indirect UV-induced radical reduction . In: Nanoscale . tape 8 , no. 14 , 2016, ISSN 2040-3364 , p. 7572-7579 , doi : 10.1039 / C6NR00156D .
- ^ A b c Siegfried Eigler, Stefan Grimm, Ferdinand Hof, Andreas Hirsch: Graphene oxide: a stable carbon framework for functionalization . In: Journal of Materials Chemistry A . tape 1 , no. 38 , September 10, 2013, doi : 10.1039 / c3ta12975f .
- ↑ a b c Ayrat M. Dimiev, Lawrence B. Alemany, James M. Tour: Graphene Oxide. Origin of Acidity, Its Instability in Water, and a New Dynamic Structural Model . In: ACS Nano . tape 7 , no. 1 , January 22, 2013, p. 576-588 , doi : 10.1021 / nn3047378 .
- ^ Christian E. Halbig, Philipp Rietsch, Siegfried Eigler: Towards the Synthesis of Graphene Azide from Graphene Oxide . In: Molecules . tape 20 , no. 12 , November 26, 2015, p. 21050-21057 , doi : 10.3390 / molecules201219747 ( mdpi.com [accessed December 12, 2016]).
- ↑ H. Pieper, CE Halbig, L. Kovbasyuk, MR Filipovic, S. Eigler: Oxo-Functionalized Graphene as a Cell Membrane Carrier of Nucleic Acid Probes Controlled by Aging . In: Chemistry - A European Journal . tape 22 , no. 43 , October 17, 2016, p. 15389-15395 , doi : 10.1002 / chem.201603063 .
- ↑ Sasha Stankovich, Richard D. Piner, SonBinh T. Nguyen, Rodney S. Ruoff: Synthesis and exfoliation of isocyanate-treated graphene oxide nanoplatelets . In: Carbon . tape 44 , no. 15 , December 1, 2006, p. 3342-3347 , doi : 10.1016 / j.carbon.2006.06.004 .
- ↑ Johannes Walter, Thomas J. Nacken, Cornelia Damm, Thaseem Thajudeen, Siegfried Eigler: Determination of the Lateral Dimension of Graphene Oxide Nanosheets Using Analytical Ultracentrifugation . In: Small . tape 11 , no. 7 , February 1, 2015, p. 814-825 , doi : 10.1002 / smll.201401940 .
- ↑ a b Christian E. Halbig, Thomas J. Nacken, Johannes Walter, Cornelia Damm, Siegfried Eigler: Quantitative investigation of the fragmentation process and defect density evolution of oxo-functionalized graphene due to ultrasonication and milling . In: Carbon . tape 96 , January 1, 2016, p. 897-903 , doi : 10.1016 / j.carbon.2015.10.021 .
- ↑ Thomas J. Nacken, Christian E. Halbig, Simon E. Wawra, Cornelia Damm, Stefan Romeis: Structural factors controlling size reduction of graphene oxide in liquid processing . In: Carbon . tape 125 , December 2017, p. 360-369 , doi : 10.1016 / j.carbon.2017.09.066 .
- ↑ Patrick Feicht, Daniel A. Kunz, Anton Lerf, Josef Breu: Facile and scalable one-step production of organically modified graphene oxide by a two-phase extraction . In: Carbon . tape 80 , December 2014, p. 229-234 , doi : 10.1016 / j.carbon.2014.08.061 .
- ↑ a b Zhenxing Wang, Siegfried Eigler, Yoshitaka Ishii, Yichen Hu, Christian Papp: A facile approach to synthesize an oxo-functionalized graphene / polymer composite for low-voltage operating memory devices . In: Journal of Materials Chemistry C . tape 3 , no. 33 , 2015, ISSN 2050-7526 , p. 8595-8604 , doi : 10.1039 / C5TC01861G .
- ↑ Christian E. Halbig, Oliver Martin, Frank Hauke, Siegfried Eigler, Andreas Hirsch: Oxo-Functionalized Graphene: A Versatile Precursor for Alkylated Graphene Sheets by Reductive Functionalization . In: Chemistry - A European Journal . tape 24 , no. 50 , September 6, 2018, p. 13348-13354 , doi : 10.1002 / chem . 201802500 .
- ↑ Morgan A. Brown, Michael S. Crosser, Matthew R. Leyden, Yabing Qi, Ethan D. Minot: Measurement of high carrier mobility in graphene in an aqueous electrolyte environment . In: Applied Physics Letters . tape 109 , no. 9 , 29 August 2016, ISSN 0003-6951 , p. 093104 , doi : 10.1063 / 1.4962141 .
- ^ Benjamin Butz, Christian Dolle, Christian E. Halbig, Erdmann Spiecker, Siegfried Eigler: Highly Intact and Pure Oxo-Functionalized Graphene: Synthesis and Electron-Beam-Induced Reduction . In: Angewandte Chemie International Edition . tape 55 , no. 51 , December 19, 2016, p. 15771–15774 , doi : 10.1002 / anie.201608377 .
- ↑ Goki Eda, Yun-Yue Lin, Cecilia Mattevi, Hisato Yamaguchi, Hsin-An Chen: Blue Photoluminescence from Chemically Derived Graphene Oxide . In: Advanced Materials . tape 22 , no. 4 , January 26, 2010, p. 505-509 , doi : 10.1002 / adma.200901996 .
- ^ Siegfried Eigler, Ferdinand Hof, Michael Enzelberger-Heim, Stefan Grimm, Paul Müller: Statistical Raman Microscopy and Atomic Force Microscopy on Heterogeneous Graphene Obtained after Reduction of Graphene Oxide . In: Journal of Physical Chemistry C . tape 118 , no. 14 , April 10, 2014, p. 7698-7704 , doi : 10.1021 / jp500580g .
- ↑ a b c L. G. Cançado, A. Jorio, EH Martins Ferreira, F. Stavale, CA Achete: Quantifying Defects in Graphene via Raman Spectroscopy at Different Excitation Energies . In: Nano Letters . tape 11 , no. 8 , 10 August 2011, p. 3190-3196 , doi : 10.1021 / nl201432g .