RNA interference
The RNA interference (short RNAi or RNA silencing ) is a natural mechanism in the cells of living organisms with a nucleus ( eukaryotes ), which the targeted shutdown of genes is used. It is a special case of gene silencing . The RNA interference is based on an interaction of short pieces of ribonucleic acid (RNA) with the genetic information -transferring mRNA with the participation of several enzyme complexes . As a result, the mRNA is split into several fragments and the information to be transmitted is destroyed or translation into a protein is prevented.
In the life sciences, RNA interference has established itself as an experimental way to shut down genes (“ gene knockdown ”). New therapies based on RNA interference are in clinical development . The two US scientists Andrew Z. Fire and Craig C. Mello received the 2006 Nobel Prize in Physiology or Medicine for their discovery of the mechanism of RNA interference .
Occurrence

The phenomenon of RNA interference can be observed in all realms of eukaryotic living things, including fungi , plants and animals . It is therefore assumed that RNA interference is an evolutionarily very old mechanism. The mechanisms of RNA interference in the biological model organisms Arabidopsis thaliana (thale cress), Caenorhabditis elegans and Drosophila melanogaster have been studied particularly well . The nematode C. elegans , in particular, serves as a model organism for researching RNA interference, since this relatively simply built organism can introduce particularly easily interfering RNA via the genetically modified E. coli bacteria that serve as food , and robust interference effects can be observed in it are.
Some components of the RNA interference machinery, such as the argonaut proteins essential for the function of RNA interference , are also found in prokaryotes . A process that is largely similar to the RNA interference of eukaryotes is the CRISPR mechanism of the prokaryotes to defend against the intrusion of foreign genetic material by viruses .
function
Different types of interfering RNA occur in nature. Although these different types of interfering RNA use common or related mechanisms, some of their function is very different.
Virus defense
RNA interference plays an important role in defense against foreign RNA, especially in plants. It thus represents a central component of the plant's defense system against RNA viruses . The siRNA ( small interfering RNA ), which is produced in plant cells as a consequence of infection with an RNA virus during the replication of the virus, is responsible for this defensive function. RNA is formed and at the same time serves the cell to recognize and destroy this foreign RNA. Numerous viruses try for their part to evade this defense mechanism by inhibiting the proteins involved in the RNA interference.
Similar mechanisms of RNA interference for defense against infection could also be found in fungi, roundworms and insects. In mammals, the occurrence of an endogenous siRNA-based defense mechanism is not certain. In mammals, this task can be performed by special cell-specific miRNA ( micro RNA ) with a direct inhibitory effect on the replication of viruses.
Regulation of gene expression
With the participation of the cell's own miRNA, RNA interference plays an important role in regulating gene expression in many multicellular organisms . The estimated 1000 different miRNAs in the human organism control the activity of about 30% of human genes. This affects numerous functions of the immune system, among other things. This means that the regulation of gene activity by miRNA is as important as regulation by transcription factors .
A cell-specific variant of the siRNA, the so-called esiRNA ( endogenous siRNA ), is also involved in the regulation of gene expression. In contrast to exogenous siRNA, this interfering RNA, which occurs in plants, fungi and animals, does not serve to defend against viruses.
Control of the transposons
Another task, the control of so-called jumping genes ( transposons ), is performed in particular by another type of interfering RNA molecules, the piRNA ( PIWI interacting RNA ). This function of RNA interference plays a special role in human spermatogenesis and the development of the embryo .
mechanism
Several related mechanisms of RNA interference are known. Components of these mechanisms are small, specialized, mostly double-stranded RNA molecules, an enzyme complex known as an RNA-induced silencing complex (RISC), and the target RNA. RNA interference can generally be divided into three phases. In the first step, larger double-stranded RNA molecules are cut into short double-stranded RNA fragments in the cell with the help of ribonuclease enzymes such as Dicer and Drosha . In the second step, these fragments are split into single strands, and one strand, the so-called 'lead strand' (not to be confused with the lead strand of DNA replication), is incorporated into the RISC enzyme complex. Ultimately, the included lead strand can activate the enzyme complex to cleave an mRNA whose base sequence is complementary to the sequence of the lead strand . In this way, the leading strand determines which mRNA is cleaved in which way. Alternatively, the enzyme complex can block the function of an mRNA complementary to the leading strand as a carrier of information without cleavage. Both ways can lead to a suppression of the conversion of genetic information about a protein into a trait .
Formation of interfering RNA
A first central step in RNA interference is the formation of double-stranded RNA molecules (dsRNA) with a length of around 20 to 30 base pairs . Depending on their origin, a distinction can be made between different types of short double-stranded RNA molecules.
The siRNA, a double-stranded small RNA comprising about 19 to 23 base pairs, each with two 3'-terminal protruding nucleotides , is formed by cleaving a large double-stranded RNA molecule. This precursor RNA can be several hundreds to thousands of base pairs long and is obtained, for example, from the replication of viral RNA. dsRNA can be of not only exogenous but also endogenous origin. The enzyme Dicer, a so-called RNase III, is particularly involved in the cleavage . This mechanism is used in particular to defend against RNA viruses.
In contrast, the miRNA is encoded by the genomic DNA . In a multi-stage process, the miRNA is formed from non-protein-coding regions of the DNA. Primarily pri-miRNA ( primary miRNA ) is generated with the help of RNA polymerase II by transcription as an RNA single strand, which folds into a characteristic secondary structure due to considerable palindromic areas . In animal cells, the pri-miRNA is split into the hairpin-shaped pre-miRNA ( precursor miRNA ) with a length of 65 to 70 nucleotides with the help of an enzyme complex, the microprocessor complex , with the participation of the RNase Drosha in the cell nucleus . The pre-miRNA formed is finally cut in the cytosol with the aid of Dicer into a miRNA double strand each comprising around 21 to 25 base pairs. In plant cells, on the other hand, a miRNA double strand is formed directly from the pri-miRNA with the participation of the RNase DCL1 located in the cell nucleus .
A variant of RNA interference found in animal sex cells uses single-stranded piRNA ( PIWI- interacting RNA). The formation of these interfering RNA molecules comprising about 24 to 31 nucleotides differs significantly from that of siRNA and miRNA. Neither double-stranded RNA precursor molecules nor type III RNAses are involved in the as yet not fully understood biogenesis of piRNA. It is assumed, among other things, that the piRNA is formed after the formation of a primary transcript by transcription of a piRNA cluster in a circulatory process called the ping-pong mechanism with the participation of PIWI proteins .
In addition to these interfering RNAs, other RNA types capable of RNA interference, such as 21U-RNA and esiRNA, are known.
Formation of the RNA-induced silencing complex
The central link in RNA interference is an enzyme complex known as the RNA-induced silencing complex (RISC). The double-stranded siRNA or miRNA molecules formed by Dicer are transferred to the argonaut proteins of the RNA-induced silencing complex . This complex loaded with double-stranded RNA is also known as pre-RISC. The double strands of the bound siRNA or miRNA are cleaved within the pre-RISC. The RNA single strand known as the guide strand remains in the RNA-induced silencing complex , which in this state is called Holo-RISC, while the other strand leaves the complex and is broken down. Finally, an mRNA that is complementary to the lead strand is incorporated into the RNA-induced silencing complex .
Function of the RNA-induced silencing complex
Cleavage of the target RNA
The best researched consequence from the activation of the RNA-induced silencing complex is the cleavage of an mRNA bound in the complex and complementary to the lead strand. This mechanism, which can be observed in particular with siRNA, requires an argonaut protein with endonuclease activity and the most perfect complementarity possible between the lead strand and the target mRNA. Of the four human argonaut proteins involved in siRNA- or miRNA-mediated RNA interference, only AGO2 has endonuclease activity. Cleavage of the target RNA can also be observed as a consequence of the piRNA-mediated RNA interference. This cleavage is due to the endonuclease activity of the PIWI proteins involved in the ping-pong cycle. The cleaved target mRNA can be broken down further in the P-bodies.
Inhibition of translation
The most important function of the RNA-induced silencing complex (especially in the case of miRNA-mediated RNA interference) is to inhibit the translation of the information from an mRNA into a protein on the ribosomes (translation). Neither endonuclease activity nor a high degree of complementarity between the leading strand and the target mRNA is required for this function. Only nucleotides 2 to 7 of the lead strand have to be complementary to those of the target mRNA. The underlying molecular mechanisms of translation inhibition are less well understood. At least two possible mechanisms, which could already be observed in the cells of the fruit fly Drosophila melanogaster , are held responsible for the inhibition of the conversion of the mRNA information into a protein. On the one hand can RNA-induced silencing complex via protein-protein interactions with Translationsinitialisierungsfaktoren block. On the other hand, a degradation of the polyadenylation signal of the mRNA can be observed by the activated RNA-induced silencing complex . Both mechanisms result in an inhibition of translation.
Inhibition of transcription
In addition to the RNA interference, small double-stranded RNA molecules can lead to an inhibition of transcription. A variant of the RNA-induced silencing complex called RNA-induced transcriptional silencing complex (RITS) is involved in this so-called transcriptional gene silencing that takes place in the cell nucleus . The activated RNA-induced transcriptional silencing complex leads to the formation of heterochromatin areas of the genome via histone modifications . These areas are no longer accessible to the transcription enzymes.
RNA activation
Another phenomenon that can be traced back to small RNA molecules in addition to RNA interference is so-called RNA activation (RNAa). The underlying mechanisms discussed are activation of transcription by promoter- specific interfering RNA, activation of translation involving Argonaut proteins, interaction with the cell's own miRNA and antisense mechanisms.
application
Basic research
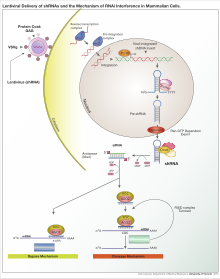
In basic research, RNA interference is used to elucidate the still unknown function of a known gene to be investigated and its encoded protein. The RNA interference allows the targeted deactivation of any gene. The function of the protein encoded by gene can be derived from this deactivation. Only the nucleotide sequence of the gene to be examined needs to be known in order to develop potentially interfering RNA molecules. This means that the application of RNA interference, also known as “gene knockdown”, to investigate the function of a gene and its encoded protein is significantly less complex than conventional gene knockout . In addition, the use of RNA interference is more time-saving and more promising than the development of a ligand as an inhibitor of protein function. This is particularly the case when a pharmacological differentiation between closely related proteins is practically impossible.
The experimental use of RNA interference is also suitable for the reverse question, the search for the genes or proteins responsible for a known function or a certain characteristic. For this purpose, RNA interference libraries with interfering RNAs against each individual gene are used, which are used with the help of high throughput screening . These so-called genome-wide RNA interference screenings are also used in pharmaceutical research in the search for new target molecules for new active ingredients ( target discovery ).
For both areas of application of RNA interference in basic research, synthetic siRNA or shRNA molecules ( short hairpin RNA ) are used in particular . They are either smuggled directly into the cells with the aid of various transfection techniques or indirectly formed in the cells after the introduction of a vector such as a shRNA-coding plasmid or a virus , using cellular transcription. To avoid misinterpretation due to the unspecific effects that occur again and again in practice, suitable control experiments are carried out or the specificity is confirmed with the help of several interfering RNAs against one and the same gene.
therapy
Although RNA interference is a recently discovered biological mechanism, the first RNA interference-based therapeutics are in the late stages of clinical development. This rapid development can be attributed on the one hand to the potential of RNA interference as a therapeutic method and on the other hand to years of experience with antisense oligonucleotides and ribozymes in clinical development. The furthest advanced was the development of bevasiranib , an anti- vascular endothelial growth factor (VEGF) siRNA that was intended to be used in the treatment of age-related macular degeneration , but which failed in a phase III clinical trial. Shortly after this setback, several large pharmaceutical companies , including Hoffmann-La Roche , discontinued their siRNA-based development programs. Regardless of this, there are other therapeutic agents based on RNA interference, such as Sirna-027 , which is directed against the VEGF receptor -1, and PF-655, which is directed against the RTP801 gene, for the treatment of age-related macular degeneration and ALN-RSV01, which is directed against a viral nucleocapsid for the treatment of respiratory syncytial virus infections, currently at least in phase II of clinical trials.
Cell-own interfering RNAs also represent potential targets for pharmaceutical development. So-called antagomires , which block cell-specific miRNAs via antisense mechanisms, are in preclinical development.
Story of discovery

Around 1990, the research group led by Joseph Mol and Richard Jorgensen tried to intensify the flower color of petunias . They intended to introduce additional copies of the gene dihydroflavonol reductase into the plants and hoped to stimulate the production of the flower pigments (from the group of flavonoids ). However, the opposite was true. To everyone's surprise, most of the genetically modified plants were less colored than untreated plants, some even snow-white. The term “cosuppression” was initially coined for this phenomenon, since not only the genes introduced into the plants, but also the corresponding dihydroflavonol reductase gene, which occurs naturally in the plants, provided little or no functional protein.
A few years later, further work showed that the genes were not only switched off at the level of transcription, but that the mRNA they produced was also rapidly broken down in the cells - a process known as Post-Transcriptional Gene Silencing (PTGS). At about the same time, similar phenomena were described from fungi ( Neurospora crassa ) under the name Quelling , from algae and from the nematode C. elegans .
In 1998, after a series of studies, it was finally clear that the mRNA itself is significantly involved in the PTGS phenomenon. Almost simultaneously with the complete sequencing of the genome of C. elegans Andrew Fire and Craig Mello 1998. The technique of RNA interference (RNAi), wherein the double-stranded RNA in C. elegans to an efficient and specific gene knockdown leads. For this they were awarded the 2006 Nobel Prize in Physiology or Medicine. How exactly the introduction of double-stranded RNA into the organism leads to the degradation of the target RNA, however, only became clear when, in 1999, Andrew J. Hamilton and David C. Baulcombe were able to isolate short RNA molecules with a length of about 25 nucleotides, which in there is a direct connection to the regulated RNA: the siRNA which gives the RNAi its specificity by binding the target RNA via base pairing.
When trying to transfer the strategy used by Craig Mello and Andrew Fire to vertebrates , however, considerable problems subsequently arose: the cells used did not seem to tolerate the long double-stranded RNAs, and programmed cell death ( apoptosis ) occurred . It was not until 2001 that Sayda Elbashir and Thomas Tuschl published a way how this problem can be circumvented. They used short double-stranded RNA with 21 nucleotides each, which do not lead to apoptosis, but are functionally sufficient for gene silencing.
It has been postulated that interfering RNA could play a role in the defense against RNA viruses and in the regulation of some mobile genetic elements (transposons). Therefore, motivated by the fact that RNA interference is a process that is probably universal in eukaryotes, attempts were made to isolate interfering RNA from untreated cells. Another group of small RNA molecules, the miRNAs, was discovered . Two of these miRNAs, lin-4 and let-7 , had previously been discovered in C. elegans and were initially referred to as stRNAs ( small temporal RNAs ).
Animation videos
- Genes silenced - the fascinating mechanism of RNA interference An animation film on the video channel of the Verlag Spektrum der Wissenschaft , published on June 22, 2012, accessed on October 27, 2016
- More animation videos on the subject on YouTube .
literature
- Jochen Graw: Genetics . Springer, Berlin; 5th, completely revised edition 2010; ISBN 978-3-642-04998-9 ; P. 313ff.
- Jocelyn E. Krebs, Elliott S. Goldstein and Stephen T. Kilpatrick: Lewin's Genes X . Jones & Bartlett Pub (Ma); 10th edition 2010; ISBN 978-0-7637-6632-0 ; P. 861ff.
- Siomi H, Siomi MC: On the road to reading the RNA interference code . In: Nature . 457, No. 7228, January 2009, pp. 396-404. doi : 10.1038 / nature07754 . PMID 19158785 .
- Jinek M , Doudna JA : A three-dimensional view of the molecular machinery of RNA interference . In: Nature . 457, No. 7228, January 2009, pp. 405-412. doi : 10.1038 / nature07755 . PMID 19158786 .
Web links
- miRBase: miRNA registry (English)
- RNA interference (RNAi) - a recent breakthrough discovery
- Technical background information from the Nobel Assembly of the Karolinska Institute (PDF, English; 1.2 MB)
- GenomeRNAi - a database for RNAi phenotypes and reagents
Individual evidence
- ↑ a b Cerutti H, Casas-Mollano JA: On the origin and functions of RNA-mediated silencing: from protists to man . In: Curr. Genet. . 50, No. 2, August 2006, pp. 81-99. doi : 10.1007 / s00294-006-0078-x . PMID 16691418 . PMC 2583075 (free full text).
- ↑ Kamath RS, Ahringer J: Genome-wide RNAi screening in Caenorhabditis elegans . In: Methods . 30, No. 4, August 2003, pp. 313-321. PMID 12828945 .
- ↑ a b Haasnoot J, Westerhout EM, Berkhout B: RNA interference against viruses: strike and counter strike . In: Nat. Biotechnol. . 25, No. 12, December 2007, pp. 1435-1443. doi : 10.1038 / nbt1369 . PMID 18066040 .
- ↑ a b c d Kurreck J: RNA interference: from basic research to therapeutic applications . In: Angew. Chem. Int. Ed. Engl . 48, No. 8, 2009, pp. 1378-1398. doi : 10.1002 / anie.200802092 . PMID 19153977 .
- ↑ a b Sontheimer EJ, Carthew RW: Silence from within: endogenous siRNAs and miRNAs . In: Cell . 122, No. 1, July 2005, pp. 9-12. doi : 10.1016 / j.cell.2005.06.030 . PMID 16009127 .
- ↑ Siomi H, Siomi MC: On the road to reading the RNA-interference code . In: Nature . 457, No. 7228, January 2009, pp. 396-404. doi : 10.1038 / nature07754 . PMID 19158785 .
- ↑ Jochen Graw: Genetics . Springer, Berlin; 5th, completely revised edition 2010; ISBN 978-3-642-04998-9 ; P. 317.
- ↑ Aravin AA, Hannon GJ, Brennecke J: The Piwi pathway piRNA Provides on adaptive defense in the transposon arms race . In: Science . 318, No. 5851, November 2007, pp. 761-764. doi : 10.1126 / science.1146484 . PMID 17975059 .
- ^ Ruby JG, Jan C, Player C, et al. : Large-scale sequencing reveals 21U-RNAs and additional microRNAs and endogenous siRNAs in C. elegans . In: Cell . 127, No. 6, December 2006, pp. 1193-1207. doi : 10.1016 / j.cell.2006.10.040 . PMID 17174894 .
- Jump up ↑ Liu J, Carmell MA, Rivas FV, et al. : Argonaute2 is the catalytic engine of mammalian RNAi . In: Science . 305, No. 5689, September 2004, pp. 1437-1441. doi : 10.1126 / science.1102513 . PMID 15284456 .
- ↑ Pratt AJ, MacRae IJ: The RNA-induced silencing complex: a versatile gene-silencing machine . In: J. Biol. Chem. . 284, No. 27, July 2009, pp. 17897-178901. doi : 10.1074 / jbc.R900012200 . PMID 19342379 . PMC 2709356 (free full text).
- ↑ Lewis BP, Burge CB, Bartel DP: Conserved seed pairing, often flanked by adenosines, indicates that thousands of human genes are microRNA targets . In: Cell . 120, No. 1, January 2005, pp. 15-20. doi : 10.1016 / j.cell.2004.12.035 . PMID 15652477 .
- ↑ Iwasaki S, Kawamata T, Tomari Y: Drosophila argonaute1 and argonaute2 employ distinct mechanisms for translational repression . In: Mol. Cell . 34, No. 1, April 2009, pp. 58-67. doi : 10.1016 / j.molcel.2009.02.010 . PMID 19268617 .
- ^ Li LC, Okino ST, Zhao H, et al. : Small dsRNAs induce transcriptional activation in human cells . In: Proc. Natl. Acad. Sci. USA . 103, No. 46, November 2006, pp. 17337-42. doi : 10.1073 / pnas.0607015103 . PMID 17085592 . PMC 1859931 (free full text).
- ↑ Vasudevan S, Tong Y, Steitz JA : Switching from repression to activation: microRNAs can up-regulate translation . In: Science . 318, No. 5858, December 2007, pp. 1931-4. doi : 10.1126 / science.1149460 . PMID 18048652 .
- ^ Krützfeldt J, Rajewsky N, Braich R, et al. : Silencing of microRNAs in vivo with 'antagomirs' . In: Nature . 438, No. 7068, December 2005, pp. 685-689. doi : 10.1038 / nature04303 .
- ↑ Ledford H: Drug giants turn their backs on RNA interference . In: Nature . 468, No. 7323, November 2010, p. 487. doi : 10.1038 / 468487a . PMID 21107398 .
- ^ Tiemann K, Rossi JJ: RNAi-based therapeutics-current status, challenges and prospects . In: EMBO Mol Med . 1, No. 3, June 2009, pp. 142-151. doi : 10.1002 / emmm.200900023 . PMID 20049714 .
- ^ Alderton GK: Therapy: A big step for targeting RNAs . In: Nature Reviews Cancer . 10, 2010, p. 313. doi : 10.1038 / nrc2848 .
- ↑ Napoli C, Lemieux C, Jorgensen R: Introduction of a chalcone synthase gene into Petunia results in reversible co-suppression of homologous genes in trans . In: Plant Cell . 2, No. 4, 1990, pp. 279-289. PMID 12354959 . PMC 159885 (free full text).
- ↑ Fire A., Xu S., Montgomery MK, Kostas SA, Driver SE, Mello CC: Potent and specific genetic interference by double-stranded RNA in Caenorhabditis elegans . In: Nature . 391, 1998, pp. 806-811. PMID 9486653 .
- ↑ Hamilton AJ, Baulcombe DC: A species of small antisense RNA in posttranscriptional gene silencing in plants . In: Science . 286, No. 5441, 1999, pp. 950-952. PMID 10542148 .
- ↑ Elbashir SM, Harborth J., Lendeckel W., Yalcin A., Weber K., Tuschl T .: Duplexes of 21-nucleotide RNAs mediate RNA interference in cultured mammalian cells . In: Nature . 411, 2001, pp. 494-498. PMID 11373684 .