enzyme


An enzyme , formerly known as ferment , is a substance that consists of giant biological molecules and that acts as a catalyst to accelerate a chemical reaction. Most enzymes are proteins , with the exception of catalytically active RNA ( ribozyme ), such as. B. snRNA or (naturally non-occurring, artificially produced) catalytically active DNA ( deoxyribozyme ). As with other proteins, they are formed in the cell via protein biosynthesis on the ribosomes . Enzymes have important functions in the metabolism of organisms : They control the majority of biochemical reactions - from digestion to transcription ( RNA polymerase ) and replication ( DNA polymerase ) of genetic information .
Origin of the word and history of enzyme research
Humans have used enzymes such as yeast and bacteria for several thousand years ; it is known that the Sumerians as early as 3000 BC Brewing beer , baking bread and making cheese . For the use of beer or baker's yeast , such as mashing or in yeast dough , and the processes of fermentation initiated by it , the term "fermentation" was created without knowing the existence of bacteria (or microbial yeasts) and their effect through enzymes.
The words fermentation and ferment found their way into the German language in the 15th century, they go back to the Latin word fermentum . Columella used this expression around 60 AD for loosening and swelling of the soil, while Seneca used it around the same time in his epistulae to describe a fermentation process that he considered necessary for the formation of honey . With this meaning as "fermentation agent" or " sourdough ", the word ferment was borrowed from Latin , and fermentation , fermentation and fermenter derived from it.
The first fermentation processes were described by Paracelsus and Andreas Libavius . The first attempts to explain this came from Johann Baptist van Helmont and Georg Ernst Stahl . After René Réaumur had examined the digestion of birds in 1752 and found that birds of prey do not have gizzards that chop up grains, but rather secrete fluid in the stomach, Lazzaro Spallanzani was able to prove in 1783 that their gastric juice alone is sufficient to liquefy meat . This refuted the theory of a purely mechanical digestive process .
The first direct use of enzymes without the involvement of microorganisms was made by the German pharmacist Constantin Kirchhoff in 1811, when he discovered that larger amounts of sugar can be produced by heating starch with the addition of sulfuric acid . The French chemist Anselme Payen refined the process in 1833; since it was assumed at that time that the sugar was only separated from the starch, this process was called "diastase" (Greek for separate); today the term “diastase” is used synonymously with amylase . This was followed by the discovery of Erhard Friedrich Leuchs in 1831 that the human saliva apparently saccharifies starch. In 1833 Eilhard Mitscherlich used the term “ferment” in connection with a substance that is not transformed during a reaction, but is necessary for a reaction to come into contact. In 1835, the Swedish chemist Jöns Jakob Berzelius suspected diastase to be a chemical process with the action of catalytic forces.
It was not until 1837 that three scientists (C. Cagniard de la Tour, T. Swann and F. Kuetzing) discovered independently of one another that yeast consists of microorganisms. Louis Pasteur showed in 1862 that microorganisms are responsible for fermentation; He concluded that the fermentation takes place through a vital force that is present in the mold cell, which he called "ferments", which do not lose their effectiveness with the death of the mold cell.
In 1878 Wilhelm Friedrich Kühne introduced today's neoclassical made-up word enzyme ( ancient Greek ἔνζυμον énzymon ), derived from ἐν- en- , “in-”, and ζύμη zýmē , which also means “the leaven” or “the yeast ”, so the sense is there "That contained in sourdough / yeast" (namely the substance that triggers or influences fermentation ). This term then found its way into international science and is now also part of the modern Greek language .
Kühne differentiated the term enzymes , which are used to describe biocatalysts effective outside of living cells, from ferments , which, according to Pasteur, can only develop their effect within living cells.
Another milestone are the studies on the enzyme specificity of Emil Fischer . He postulated in 1890 that enzymes and its substrate like a lock and key the matching behavior. In 1897 Eduard Buchner discovered on the basis of alcoholic fermentation that enzymes can also have a catalytic effect without the living cell; In 1907 he received the Nobel Prize for demonstrating cell-free fermentation. In 1903 Eduard Buchner and Jakob Meisenheimer managed to kill microorganisms that triggered lactic and acetic acid fermentation without affecting their enzyme action. The German chemist Otto Röhm isolated enzymes for the first time in 1908 and developed processes for enzymatic leather tanning , fruit juice cleaning and a number of diagnostic applications.
At the beginning of the 20th century, the chemical composition of enzymes was still unknown. It was suggested that enzymes consist of protein and that their enzymatic activity is associated with their structure. However, other scientists such as Richard Willstätter argued that proteins are only carriers of the “real enzymes” and that they are unable to initiate a catalytic reaction on their own. James B. Sumner showed in 1926 that the enzyme urease is a pure protein and was able to crystallize it. The last doubts about the composition of enzymes were dispelled by John H. Northop and Wendell M. Stanley when they demonstrated in 1930 that pepsin , trypsin and chymotrypsin consist of pure protein. Northrop and Stanley received the Nobel Prize in Chemistry for this in 1946.
The knowledge of how to crystallize enzymes has now enabled the researchers to use crystal structure analysis to clarify the structure and functionality of enzymes at the atomic level. In the years 1930 to 1939 the crystal structures of eleven other enzymes were discovered. The first amino acid sequence that was completely deciphered by an enzyme is that of the ribonuclease . This step was achieved by Stanford Moore and William Howard Stein . In 1969 Robert Bruce Merrifield then synthesized the entire sequence of the ribonuclease using the technique named after him ( Merrifield synthesis ). At the same time, RG Denkewalter and R. Hirschmann also managed to do this .
In the 1980s were catalytic antibodies by Richard Lerner discovered that an enzyme activity had once against the transition state modeled on molecular immunized was. Linus Pauling had already suspected in 1948 that enzymes bind molecules similar to the transition state particularly well. At the end of the 1980s it was discovered that RNA can also develop catalytic (enzymatic) activity in the organism ( ribozyme ). In 1994 the first deoxyribozyme, GR-5 , was developed.
Researchers like Leonor Michaelis and Maud Menten pioneered the study of enzyme kinetics by formulating the Michaelis-Menten theory .
Nomenclature and classification according to IUPAC and IUBMB
nomenclature
The IUPAC and IUBMB have joined a so-called nomenclature developed the enzymes that these homogeneous and numerous containing group of representatives molecules classified . For this purpose, the IUPAC developed principles of nomenclature:
- Enzyme names have the extension (or suffix ) "-ase" when the enzyme in question chemical or organic compounds separates or splits (such as the " hydrol ase n " or " Prote ase n ") or neuverbindet (such as the " oxide ase n "or" Telomer ase ").
- The enzyme name is intended to be explanatory, i.e. to describe the reaction that the enzyme catalyzes. (Example: Cholinesterase : An enzyme which the ester group in the choline molecule hydrolyzed .)
- The enzyme name should contain its classification (see below). (Example: choline esterase )
In addition, a code system, the EC number system, was developed in which the enzymes are classified under a numerical code made up of four numbers. The first number indicates one of the seven enzyme classes. Lists of all detected enzymes ensure that the specified enzyme code can be found more quickly, e.g. B. at BRENDA . Although the codes are based on the properties of the reaction that the enzyme catalyzes, in practice numerical codes prove to be unwieldy. Systematic names based on the above rules are used more frequently. Problems with the nomenclature arise, for example, with enzymes that catalyze several reactions. Therefore there are sometimes several names for them. Some enzymes have trivial names that do not indicate that the substance mentioned is an enzyme. Since the names were traditionally widely used, they were partially retained (examples: the digestive enzymes trypsin and pepsin in humans).
classification
Enzymes are divided into seven enzyme classes according to the reaction they catalyze:
- EC 1: Oxidoreductases that catalyze redox reactions.
- EC 2: Transferases that transfer functional groups from one substrate to another.
- EC 3: hydrolases that cleave bonds using water .
- EC 4: Lyases that catalyze the cleavage or synthesis of more complex products from simple substrates, but without the consumption of adenosine triphosphate (ATP) or another nucleoside triphosphate (NTP).
- EC 5: isomerases that accelerate the conversion of chemical isomers .
- EC 6: ligases or synthetases that catalyze addition reactions with the help of ATP (or another NTP). A reverse reaction (splitting) is usually energetically unfavorable and does not take place.
- EC 7: Translocases , the transport of substances to or through cell membranes.
Some enzymes are able to catalyze several, sometimes very different, reactions. If this is the case, they are assigned to several enzyme classes.
construction
Enzymes can be differentiated based on their structure. While many enzymes consist of only one polypeptide chain , so-called monomers , other enzymes, the oligomers , consist of several subunits / protein chains. Some enzymes combine with other enzymes to form so-called multi- enzyme complexes and cooperate or regulate each other. Conversely, there are also individual protein chains that can exert several different enzyme activities ( multifunctional enzymes ). Another possible classification with regard to their structure takes into account the presence of cofactors :
- Pure protein enzymes are made up entirely of proteins. The active center is formed only from amino acid residues and the peptide backbone. This group includes, for example, the digestive enzyme chymotrypsin and the triose phosphate isomerase (TIM) of glycolysis .
- Holoenzymes ( old Gr . Ὅλος holos “whole”, “complete” and enzyme) consist of a protein component, the apoenzyme , and a cofactor, a low molecular weight molecule (not a protein). Both together are important for the function of the enzyme. Organic molecules as cofactors are called coenzymes . If they are covalently bound to the apoenzyme, they are called prosthetic groups , otherwise they are also called cosubstrate , since they are reacted with the substrate in equivalent amounts during the enzymatic reaction. Examples of cosubstrates are adenosine triphosphate (ATP) and nicotinamide adenine dinucleotide (NAD). ATP is often used as an energy source for protein kinases to react . NAD is used as an electron acceptor by enzymes such as alcohol dehydrogenase . Requires an enzyme metal ions ( iron -, zinc - or copper ion), one speaks of a metalloenzyme . The lipoxygenase , for example, iron and the carbonic anhydrase contains zinc.
The protein-RNA complexes or protein-ribozyme complexes form a special group, examples of which are the telomerases . The ribosomes are also such complexes.
function
Enzymes are biocatalysts . They accelerate biochemical reactions by reducing the activation energy that has to be overcome in order for a substance to be converted. This increases the reaction rate (see transition state theory ). Theoretically, an enzymatic conversion is reversible; This means that the products can be converted back into the starting materials. The starting materials (educts) of an enzyme reaction, the substrates , are bound in the so-called active center of the enzyme, an enzyme-substrate complex is formed . The enzyme now enables the substrates to be converted into the reaction products, which are then released from the complex. Like all catalysts, the enzyme is back in its original form after the reaction. Enzymes are characterized by high substrate and reaction specificity ; from numerous substances they only select the appropriate substrates and catalyze exactly one of many conceivable reactions.
Energetic foundations of catalysis
Most biochemical reactions would only take place with negligible speed without enzymes in living things. As with any spontaneous reaction, the free enthalpy of reaction ( ) must be negative. The enzyme accelerates the establishment of the chemical equilibrium - without changing it. The catalytic effectiveness of an enzyme is based solely on its ability to lower the activation energy in a chemical reaction : this is the amount of energy that must first be invested in order to set the reaction going. During this, the substrate is increasingly changed, it adopts an energetically unfavorable transition state . The activation energy is now the amount of energy that is needed to force the substrate into the transition state. This is where the catalytic effect of the enzyme comes in: through non-covalent interactions with the transition state, it stabilizes it, so that less energy is required to bring the substrate into the transition state. The substrate can be converted into the reaction product much more quickly, as a way is “paved” for it.
The active center - structural basis for catalysis and specificity
The active center (catalytic center) is responsible for the catalytic effectiveness of an enzyme . At this point it binds the substrate and is then "actively" converted. The active center consists of folded parts of the polypeptide chain or reactive non-protein parts (cofactors, prosthetic groups) of the enzyme molecule and determines the specificity of the enzymatic catalysis. This specificity is based on the complementarity of the spatial structure and the possible superficial interactions between enzyme and substrate. An enzyme-substrate complex is formed .
The spatial structure of the active center means that only a structurally suitable substrate can be bound. Illustratively, a certain substrate fits the corresponding enzyme like a key in the appropriate lock ( key-lock principle ). This is the reason for the high substrate specificity of enzymes. In addition to the lock-and-key model, there is also the non-rigid induced fit model : Since enzymes are flexible structures, the active center can be reshaped through interaction with the substrate.
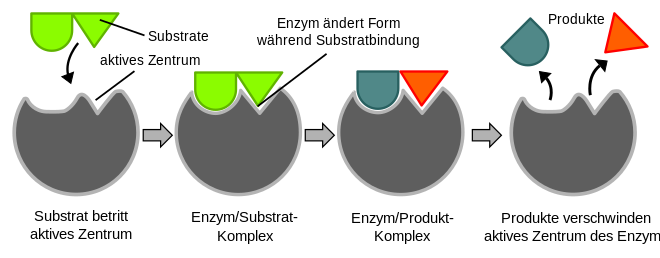
Even small structural differences in the spatial structure or charge distribution of the enzyme can lead to a substance similar to the substrate no longer being recognized as a substrate. Glucokinase, for example, accepts glucose as a substrate, but its stereoisomer, galactose , does not. Enzymes can have different substrate specificities, for example alcohol dehydrogenases break down other alcohols in addition to ethanol and hexokinase IV accepts not only glucose but also other hexoses as substrates.
The substrate is recognized and bound by non-covalent interactions ( hydrogen bonds , electrostatic interaction or hydrophobic effects ) between parts of the enzyme and the substrate. The binding of the enzyme must be strong enough to bind the often poorly concentrated substrate (micro- to millimolar concentrations), but it must not be too strong since the reaction does not end with the binding of the substrate. It is important that the transition state of the reaction is bound even more strongly and thus its stabilization. It is not uncommon for two substrates to take part in a reaction; the enzyme then has to guarantee the correct orientation of the reaction partners to one another. These latter mechanistic properties of an enzymatic reaction are the basis of the specificity of an enzyme's action . It only ever catalyzes one of many possible reactions of the substrates. The activity of enzymes is partially regulated by pseudoenzymes (variants of enzymes without enzyme activity).
Catalytic Mechanisms
Although the detailed mechanisms of enzymatic reactions are varied, enzymes usually use one or more of the following catalytic mechanisms.
- Preferential binding of the transition state
- The binding of the transition state is stronger than the binding of the substrates and products, which results in a stabilization of the transition state.
- Orientation and approach of substrates
- The binding of two substrates in the appropriate orientation and conformation can significantly increase the reaction rate, since the reactive groups of the molecules come into the right position to one another and the conformations of the molecules that are favorable for the reaction are stabilized.
- General acid-base catalysis
- Amino acid residues, for example from histidine, react as acid or base by taking up or releasing protons ( H + ions ) during a reaction .
- Covalent catalysis
- Amino acid residues or coenzymes form covalent bonds with a substrate and form a short-lived intermediate . Usually nucleophilic amino acid side chains (for example lysine side chains with an amino group ) or coenzymes such as pyridoxal phosphate are involved in such reactions .
- Metal ion catalysis
- Metal ions can support the catalysis as structure-stabilizing coordination centers , redox partners (often iron or copper ions) or as Lewis acids (often zinc ions). They can stabilize or shield negative charges or activate water molecules.
Enzyme kinetics
Enzyme kinetics deals with the course of enzymatic reactions over time. A key factor here is the speed of reaction . It is a measure of the change in the substrate concentration over time, i.e. the amount of substrate that is converted in a certain reaction volume per unit of time (unit: mol / (l · s)). In addition to the reaction conditions such as temperature , salt concentration and pH value of the solution, it depends on the concentrations of the enzyme, the substrates and products as well as effectors (activators or inhibitors).
The enzyme activity is related to the reaction rate. It indicates how much active enzyme there is in an enzyme preparation. The units of enzyme activity are unit (U) and katal (kat), where 1 U is defined as the amount of enzyme which converts one micromole of substrate per minute under the specified conditions: 1 U = 1 μmol / min. Katal is rarely used, but is the SI unit of enzyme activity: 1 kat = 1 mol / s. Another important measurand for enzymes is the specific activity (activity per unit mass, U / mg). This shows how much of the total protein in the solution is really the enzyme you are looking for.
The measured enzyme activity is proportional to the reaction rate and therefore strongly dependent on the reaction conditions. It rises with the temperature in accordance with the RGT rule : an increase in temperature by approx. 5–10 ° C leads to a doubling of the reaction rate and thus also the activity. However, this only applies to a limited temperature range. When an optimal temperature is exceeded, there is a steep drop in activity due to denaturation of the enzyme. Changes in the pH of the solution often have dramatic effects on enzyme activity, as this can affect the charge of individual amino acids in the enzyme that are important for catalysis. Beyond the pH optimum, the enzyme activity decreases and eventually comes to a standstill. The same applies to the salt concentration or the ionic strength in the environment.
Michaelis-Menten theory
A model for the kinetic description of simple enzyme reactions is the Michaelis-Menten theory (MM theory). It provides a relationship between the reaction rate v of an enzyme reaction and the enzyme and substrate concentration [E 0 ] and [S] . It is based on the assumption that an enzyme forms an enzyme-substrate complex with a substrate molecule and this either breaks down into enzyme and product or into its starting components. What happens faster depends on the respective rate constants k .
The model states that the higher the substrate concentration, the faster the reaction. This happens linearly at the beginning and then flattens out until a further increase in the substrate concentration no longer has any influence on the speed of the enzyme, since it is already working at maximum speed V max . The MM equation is as follows:
The parameters K m ( Michaelis constant ) and k cat ( turnover number ) are suitable for characterizing enzymes kinetically, i. That is, to make statements about their catalytic efficiency . If K m is very low, for example, this means that the enzyme reaches its maximum speed even at a low substrate concentration and thus works very efficiently. At low substrate concentrations, the specificity constant k cat / K m is a more suitable measure of the catalytic efficiency. If it reaches values of more than 10 8 to 10 9 M −1 s −1 , the reaction speed is only limited by the diffusion of the substrate and enzyme molecules. Any chance contact between the enzyme and the substrate leads to a reaction. Enzymes that achieve this level of efficiency are called “catalytically perfect”.
Cooperativity and Allostery
Some enzymes do not show the hyperbolic saturation curve as predicted by the Michaelis-Menten theory, but rather a sigmoid saturation behavior. Such a thing was first with binding proteins such as hemoglobin described and as positive cooperativity multiple binding sites interpreted: the binding of a ligand (substrate molecule) influenced more binding sites in the same enzyme (often but in other subunits ) in their affinity . With positive cooperativity, a binding protein with many free binding sites has a weaker affinity than a largely occupied protein. If the same ligand binds to all binding centers, one speaks of a homotropic effect . In the case of enzymes, cooperativity is closely linked to allostery . Allostery is understood to mean the presence of further binding sites (allosteric centers) in an enzyme, apart from the active center. If effectors (not substrate molecules) bind to allosteric centers, a heterotropic effect is present. Allostery can be conceptually differentiated from cooperativity, but they often occur together.
Multi-substrate reactions
The previous considerations only apply to reactions in which a substrate is converted into a product. However, many enzymes catalyze the reaction of two or more substrates or co-substrates. Several products can also be created. In the case of reversible reactions, the distinction between substrate and product is relative anyway. The Michaelis-Menten theory only applies to one of several substrates if the enzyme is saturated with the other substrates.
The following mechanisms are conceivable for multi-substrate reactions:
- Sequential Mechanism
- The substrates sequentially bind to the enzyme. If all substrates have bound, there is a central complex . This is where the substrates are converted into products, which are then released from the complex one after the other. A distinction is made between:
- Random mechanism (Engl. Random ): The order of substrate binding is random.
- Parent mechanism (engl. Ordered ): The order of binding is fixed.
- Ping pong mechanism
- The binding of the substrate and the release of the product take place alternately. First substrate A binds to the enzyme and the first product P is split off. The enzyme is modified in the process. The second substrate B is then taken up and reacts to form a second product Q. The enzyme has its original shape again.
Enzyme inhibition


As the enzyme inhibition (inhibition) is defined as the reduction in the catalytic activity of an enzyme by a specific inhibitor ( inhibitor ). A fundamental distinction is made between irreversible inhibition , in which an inhibitor enters into a bond with the enzyme that is irreversible under physiological conditions (such as penicillin with D-alanine transpeptidase ), and reversible inhibition , in which the enzyme-inhibitor complex formed is restored can break down into its components. In the case of reversible inhibition, one differentiates between
- competitive inhibition - the substrate competes with the inhibitor for binding to the active site of the enzyme. However, the inhibitor cannot be converted enzymatically and thus stops the enzyme work by blocking the active center;
- allosteric inhibition (also non-competitive inhibition ) - the inhibitor binds to the allosteric center and thereby changes the conformation of the active center so that the substrate can no longer bind there;
- uncompetitive inhibition - the inhibitor binds to the enzyme-substrate complex and thus prevents the catalytic conversion of the substrate into the product.
- End product inhibition - the end product of a series of enzymatic conversions blocks enzyme 1 and thus terminates the conversion of the starting substrate into the product. This negative feedback limits the amount of production in some metabolic processes.
Regulation and control of enzyme activity in the organism
In the living organism, enzymes work together in a complex network of metabolic pathways. In order to be able to optimally adapt to fluctuating internal and external conditions, a fine regulation and control of the metabolism and the underlying enzymes is necessary. Under regulation refers to processes which both maintain a stable internal conditions in changing environmental conditions ( homeostasis serve). As a control is referred to changes that take place on the basis of external signals (e.g., by hormones). There are fast / short-term, medium-term and slow / long-term regulatory and control processes in the metabolism:
Short term adjustment
Rapid changes in enzyme activity occur as a direct response of the enzymes to changed concentrations of metabolic products, such as substrates, products or effectors (activators and inhibitors). Enzyme reactions that are close to equilibrium are sensitive to changes in substrate and product concentrations. Accumulation of substrate accelerates the forward reaction , accumulation of product inhibits the forward reaction and promotes the reverse reaction (competitive product inhibition) . In general, however, the irreversible enzyme reactions are assigned a greater role in metabolic regulation and control.
Allosteric modulation is of great importance . Substrate or effector molecules that arise in the metabolism bind to the allosteric centers of the enzyme and change its catalytic activity. Allosteric enzymes consist of several subunits (either from the same or from different protein molecules). The binding of substrate or inhibitor molecules to a subunit leads to conformational changes in the entire enzyme, which change the affinity of the other binding sites for the substrate. A final product inhibition ( feedback inhibition ) arises when the product operates a chain of reactions to the enzyme at the beginning of this chain allosteric retardant. This automatically creates a control loop .
Medium-term adjustment
A common form of metabolic control is the covalent modification of enzymes, especially phosphorylation . As with a molecular switch, the enzyme can be switched on or off for example after a hormonal signal by phosphate-transferring enzymes ( kinases ). The introduction of a negatively charged phosphate group leads to structural changes in the enzyme and can in principle favor both active and inactive conformations. The cleavage of the phosphate group by phosphatases reverses this process, so that a flexible adaptation of the metabolism to changing physiological requirements is possible.
Long-term adjustment
As a long-term reaction to changed demands on the metabolism, enzymes are specifically degraded or newly formed. The formation of new enzymes is controlled by the expression of their genes. Such a type of genetic regulation in bacteria describes the operon model by Jacob and Monod . The controlled breakdown of enzymes in eukaryotic cells can be realized by ubiquitination . The attachment of polyubiquitin chains to enzymes, catalyzed by specific ubiquitin ligases, marks them for degradation in the proteasome , a “garbage chute” of the cell.
Biological importance
Enzymes are not to be underestimated biological significance, they play the central role in the metabolism of all living organisms. Almost every biochemical reaction is brought about and controlled by enzymes. Well-known examples are glycolysis and citrate cycle , respiratory chain and photosynthesis , transcription and translation as well as DNA replication . Enzymes not only act as catalysts, they are also important regulation and control points in metabolic processes.
The importance of the enzymes is not limited to the metabolism, they are also important in the absorption and transmission of stimuli. At the signal transduction , ie the exchange of information within a cell receptors are frequently involved with enzymatic function. Also kinases such as tyrosine kinases and phosphatases play a crucial role in the transmission of signals. The activation and deactivation of the information carriers, i.e. the hormones, are carried out by enzymes.
Furthermore, enzymes are involved in the defense of one's own organism, for example various enzymes such as the serine proteases of the complement system are part of the unspecific immune system of humans.
Defects in enzymes can have fatal consequences. Such enzyme defects reduce the activity of an enzyme or no longer exist. Some enzyme defects are genetically inherited; that is, the gene encoding the amino acid sequence of the corresponding enzyme contains one or more mutations or is absent entirely. Examples of inheritable enzyme defects are phenylketonuria and galactosemia .
Artificial enzymes (for example in bread dough that are not denatured during the baking process) carry the risk of triggering allergies
Use and occurrence in everyday life
Enzymes are valuable tools in biotechnology . Their possible uses range from cheese production ( rennet ) to enzymatics and genetic engineering . For certain applications, scientists today are specifically developing more powerful enzymes through protein engineering . In addition, a new type of catalytically active protein was constructed, the catalytic antibodies , which were called abzymes because of their similarity to the enzymes . Also ribonucleic acids (RNA) can be catalytically active; these are then called ribozymes .
Enzymes are needed, among other things, in industry. Detergents and dishwashing detergents are used to add lipases (enzymes that break down fat), proteases ( enzymes that break down protein ) and amylases ( enzymes that break down starch ) to increase cleaning performance, because these enzymes break down the stains in clothing or leftovers on dishes.
Enzymes are also used in the manufacture of some medicines and insect repellants. In the cheese-making acts rennet with, an enzyme derived from calves' stomachs.
Many enzymes can now be produced with the help of genetically modified microorganisms .
The enzymes contained in raw pineapples , kiwi fruits and papayas prevent cakes from setting gelatin , an undesirable effect if, for example, a fruit cake that contains raw pieces of these fruits is to be covered with a firm cake gelatin topping. The filling does not remain soft when using canned fruit ; these are pasteurized , whereby the protein-degrading enzymes are deactivated.
When peeling fruit and vegetables, plant cells are damaged and enzymes are released as a result. As a result, the peeled product ( clearly visible in apples and avocados ) can turn brown due to the enzymatic reaction of flavonoids or other sensitive ingredients with atmospheric oxygen. The addition of lemon juice acts as an antidote. The ascorbic acid contained in lemon juice prevents oxidation or reduces already oxidized compounds (addition of ascorbic acid as a food additive ).
In the medical enzymes play an important role. Many drugs block or increase the effects of enzymes to cure a disease. The most prominent representative of such drugs is probably acetylsalicylic acid , which inhibits the enzyme cyclooxygenase and thus has a pain-relieving effect, among other things.
Enzymes in technology
The following table gives an overview of the fields of application of enzymes. See protein for how to make it .
technical process | Enzymes | effect |
---|---|---|
Starch processing | α- amylase , glucoamylase | Starch hydrolysis |
Resolution | L- acylase | Manufacture of amino acids |
laundry detergent | Proteases , lipases | Hydrolysis of proteins , fats |
Cheese production | Proteases | Milk curdling |
Distillery products | α-amylase, glucoamylase | Starch saccharification |
Brewing industry | α-amylase, glucoamylase, proteases | Mashing process |
Fruit juice processing | Pectinases , α-amylase | Hydrolysis of pectins or starch |
Bakery production | α-amylase, proteases, pentosanase | partial hydrolysis of flour and dough ingredients |
Leather processing | Proteases | Softening, depilating leather |
Textile industry | α-amylase | Starch hydrolysis, desizing |
The importance of enzymes in medical diagnostics
The diagnostic uses enzymes to discover diseases. In the test strips for diabetics , for example, there is an enzyme system that, under the action of blood sugar, produces a substance whose content can be measured. This is how the blood sugar level is measured indirectly. This procedure is called an "enzymatic measurement". It is also used in medical laboratories to determine glucose (blood sugar) or alcohol. Enzymatic measurements are relatively easy and inexpensive to use. The substrate specificity of enzymes is used. So an enzyme is added to the body fluid to be analyzed, which can specifically convert the substrate to be measured. From the amount of reaction products produced, one can then read off how much of the substrate was present in the body fluid.
A number of enzymes can also be measured directly in human blood based on their activity. Some of the enzymes circulating in the blood come from specific organs. Conclusions about damage to certain organs can therefore be drawn on the basis of the decrease or increase in enzyme activities in the blood. Inflammation of the pancreas can be recognized by the greatly increased activity of lipase and pancreatic amylase in the blood.
literature
- Jeremy M. Berg, John L. Tymoczko, Lubert Stryer: Biochemistry. 5th edition. Spectrum Academic Publishing House, Heidelberg - Berlin 2003, ISBN 3-8274-1303-6 .
- David Fell: Understanding the Control of Metabolism . Portland Press Ltd, London 1997, 2003, ISBN 1-85578-047-X .
- Alfred Schellenberger (Ed.): Enzyme catalysis. Introduction to the chemistry, biochemistry and technology of enzymes . Gustav Fischer Verlag, Jena 1989, ISBN 3-540-18942-4 .
- Donald Voet, Judith G. Voet: Biochemistry. 3. Edition. John Wiley & Sons Inc., London 2004, ISBN 0-471-39223-5 .
- Maria-Regina Kula: Enzymes in Technology. Chemistry in our time , 14th year 1980, No. 2, pp. 61-70, doi: 10.1002 / ciuz.19800140205
- Brigitte Osterath, Nagaraj Rao, Stephan Lütz, Andreas Liese: Technical application of enzymes: white laundry and green chemistry. Chemistry in our time 41 (4), pp. 324-333 (2007), doi: 10.1002 / ciuz.200700412
- Otto Westphal , Theodor Wieland , Heinrich Huebschmann: life regulator. Of hormones, vitamins, ferments and other active ingredients. Societäts-Verlag, Frankfurt am Main 1941 (= Frankfurter Bücher. Research and Life. Volume 1), especially pp. 57–64 ( History of Ferment Research ).
Web links
- IUBMB - List and nomenclature of enzymes
- BRENDA extensive enzyme database
- ExploreEnz - The Enzyme Database
- Enzyme database with search engine
- KEGG Metabolic Pathway Database (graphic representations of the biochemical reactions with the associated, systematically identified enzymes)
- Selection of enzymes that are added to bread dough
Individual evidence
- ↑ a b c d e f Wolf-Dieter Müller-Jahncke , Christoph Friedrich , Ulrich Meyer: Medicinal history . 2nd, revised and expanded edition. Wissenschaftliche Verlagsgesellschaft mbH, Stuttgart 2005, ISBN 978-3-8047-2113-5 , p. 106 .
- ^ A b Kluge Etymological Dictionary of the German Language, 24th edition.
- ↑ Dictionary at in.gr, entry Enzyme. Retrieved May 29, 2013.
- ^ Wolf-Dieter Müller-Jahncke : Enzymes. In: Werner E. Gerabek , Bernhard D. Haage, Gundolf Keil , Wolfgang Wegner (eds.): Enzyklopädie Medizingeschichte. de Gruyter, Berlin / New York 2005, ISBN 3-11-015714-4 , p. 356 f .; here: p. 356.
- ^ The Nobel Prize in Chemistry 1946. In: nobelprize.org , accessed November 19, 2016.
- ^ A. Tramontano, KD Janda, RA Lerner: Catalytic antibodies. In: Science. Volume 234, Number 4783, December 1986, pp. 1566-1570. PMID 3787261 .
- ^ RA Lerner, SJ Benkovic, PG Schultz: At the crossroads of chemistry and immunology: catalytic antibodies. In: Science. Volume 252, Number 5006, May 1991, pp. 659-667. PMID 2024118 .
- ^ L. Pauling: Chemical achievement and hope for the future. In: American scientist. Volume 36, Number 1, January 1948, pp. 51-58. PMID 18920436 .
- ↑ Ronald R. Breaker, Gerald F. Joyce: A DNA enzyme that cleaves RNA . In: Chem Biol. Volume 1 , no. 4 , December 1994, pp. 223-229 , doi : 10.1016 / 1074-5521 (94) 90014-0 , PMID 9383394 .
- ↑ -ase - Duden , 2016
- ↑ JCBN / NC-IUB Newsletter 1984: Synthases and Ligases (English).
- ^ Nomenclature Committee of the International Union of Biochemistry and Molecular Biology (NC-IUBMB) Enzyme Nomenclature. Recommendations: EC 7. Translocases (English)
- ↑ Archived copy ( memento of the original dated February 24, 2016 in the Internet Archive ) Info: The archive link was inserted automatically and not yet checked. Please check the original and archive link according to the instructions and then remove this notice.
- ↑ Ulrich Weber (Ed.): Biologie Oberstufe Complete Volume , Cornelsen Verlag Berlin 2001, ISBN 3-464-04279-0 , p. 72.
- ↑ Functional enzymes (in bread dough)
- ↑ Artificial enzymes could trigger allergies
- ↑ Robert Ebermann, Ibrahim Elmadfa: Textbook of food chemistry and nutrition. 2nd Edition. Springer-Verlag Vienna New York, 2008 and 2011, ISBN 978-3-7091-0210-7 , p. 594, page online at Google Books .