Patch clamp technique

The patch clamp ( English patch clamp technique ) is a measuring method in the electrophysiology at which the current through individual ion channels in the cell membrane of a cell will be represented measured while current levels of a few picoamperes (10 -12 ampere ). This technique was first described in 1976 by Erwin Neher and Bert Sakmann . For their work on the function of individual cellular ion channels , which they carried out with this technique, they were awarded the 1991 Nobel Prize in Physiology or Medicine . The patch-clamp technique revolutionized electrophysiological research with the ability to observe the electrical behavior of membrane proteins on individual molecules .
The term patch refers to the small membrane cutout ( patch : patch) under the patch pipette, which also serves as a measuring electrode. During the measurement, the membrane patch is held at a predetermined potential ( to clamp ). Depending on whether this membrane area is detached from the cell after the patch pipette has been put on or whether it is measured on the intact cell, certain configurations are distinguished in the patch clamp technique.
overview
The patch-clamp technology is based on the technology of the voltage clamp (English voltage clamp ; English to clamp : fasten, festklemmen; English voltage : voltage), which was used in the 1930s by Kenneth Cole and HJ Curtis to measure currents intact nerve cells. In this process, two electrodes are pierced into the cell: one is used to set a holding or command voltage, while another electrode is used to record the currents that occur across the membrane. Using this technology, the sum of all individual currents through the cell membrane is measured; it is not possible to resolve individual components.
All cells contain so-called ion channels in the plasma membrane surrounding them - proteins that can act like pores for the ions of dissolved salts . Ion channels are also found in the membranes inside the cell, in plants for example in the tonoplast - the membrane that separates the central vacuole from the cytoplasm . In living cells, these ions are present in unequal concentrations on the outside and inside of the cell membrane; this uneven distribution leads to an electrical voltage across the membrane, the transmembrane potential . Ion channels play an important role both in maintaining the resting membrane potential of the cell and in possible changes which, when erratic , are called action potentials . Action potentials are used to transmit electrical impulses in the organism (for example, when conducting excitation ). The resting membrane potential typically assumes values of around −70 mV for animal cells and up to −200 mV for plant cells.
Since the carrier substance of biomembranes , the lipid bilayer , is practically impermeable to water and dissolved ions, it was assumed early on that a change in conductivity can only be achieved by opening or closing specific membrane proteins, the ion channels. The two British scientists Alan Hodgkin and Andrew Huxley developed a model that predicted the existence of voltage-dependent gates , which completely open or close according to the all-or-nothing principle , in the cell membrane. In 1969, this gate function was demonstrated for the first time for the bacterial membrane protein gramicidin A.
Using voltage clamp measurements, however, it was not possible to research the molecular mechanisms of these gates , the ion channels. The patch-clamp technique has been significantly enhanced. Here, the electrode is no longer inserted into the cell, but is placed directly on the cell membrane. Due to the very small opening of the pipette (approx. 1 µm ), it is very likely that a single ion channel protein can be detected. At the same time, an electrically tight connection is established between the glass edge of the measuring electrode and the cell membrane, so that leakage currents that occur can be neglected. In this way, very small currents (on the order of 5 pA) can be measured. The electrode is used to set holding or command voltages as well as to measure the ion currents. This double task is made possible by the use of an operational amplifier connected to virtual ground in the measurement electronics; a second electrode with cell contact can be omitted.
Overall, the patch-clamp technology places high demands on the devices used and the measurement electronics (low noise, long-term stability), at the same time it requires the experimenter to be very skilled in handling the device and biological material.
Devices and materials
Measuring station
For work with patch clamp technology, a measuring station is usually set up, which is typically equipped with various devices. A Faraday cage for electrical shielding stands on a vibration-damped measuring table . Inside there is an inverted microscope with a micromanipulator for positioning the patch pipette. The pipette holder is connected to the preamplifier , the sample holder to the bath electrode. The signal from the preamplifier is amplified in the patch clamp amplifier. A monitor is used to observe the measurement object and the patch pipette through the microscope camera. In most cases, a computer and data storage device is located directly at the measuring station for the digital recording to evaluate and record the electrical signals . The behavior of the cell can also be recorded with a video recorder .
Measuring electrode (patch pipette)
The measuring electrode is made from a glass capillary, which is pulled out very thinly when exposed to heat. Borosilicate glass is usually used as the glass material ; pulling out can be automated with suitable devices ( puller ); parameters such as heating temperature and tension can be set. The tip of the pipette is often heat-polished after it has been pulled , which creates the smoothest possible edges at the tip, which promote the formation of an electrically tight connection ( gigaseal ) between the pipette and the membrane.
The pipette becomes an electrode by filling it with a conductive solution into which a silver wire coated with silver chloride is immersed. The filled patch pipette is clamped in the holder and connected to the preamplifier attached there, together with another silver wire electrode that is in the bath solution. The short distance between the measuring electrode and the amplifier is necessary to reduce the superimposition of the very small measuring currents caused by external interference signals to a minimum. The resistance of the measuring electrode filled with solution is typically between 1 and 5 MΩ . An additional coating of the patch pipette with silicone elastomers reduces the noise of the measuring arrangement and prevents its electrical capacity from increasing due to wetting with the bath solution. The tip remains free up to about 50 µm.
A gigaseal usually only forms when freshly (a few hours old) manufactured patch pipettes are used for the measurement.
Prepare the cells
The outer cell membrane of cells is rarely fully accessible; for a patch clamp measurement, the cells often have to be prepared first.
Animal cells
Animal cells can be enzymatically detached from the basal lamina and freed from remnants of connective tissue . This step can sometimes be omitted for cells that have grown in cell culture .
Plant cells
Plant cells are surrounded by a cell wall, which is usually removed by enzymatic digestion with cellulases and hemicellulases . As soon as the components of the cell wall have been sufficiently broken down, the cells can be removed from the remaining residues by simply shaking them gently or by transferring them to a hypoosmotic solution. Such protoplasts (cells without a cell wall) often start again with the formation of new cell wall material immediately after they have been removed from the enzyme bath. The measurement should therefore be made as soon as possible after protoplasting .
Performing the measurement
The filled patch pipette is clamped in a micromanipulator, connected to the patch clamp amplifier and then carefully pressed onto an intact cell under optical control (observation in the microscope or on a monitor connected to it) . Below the pipette, within the diameter of the tip, there is a piece of membrane - the patch or membrane patch . A strong connection (seal) is then created between the membrane and the pipette by applying a slight negative pressure to the rear end of the pipette. This creates an electrical resistance in the order of magnitude of several gigaohms (10 9 ohms) between the inside of the pipette and the external solution , the so-called “gigaseal” ( to seal ). With the manufacture of the Gigaseal, the so-called cell-attached configuration of the patch-clamp technology has been achieved ( to attach to, to attach to, to attach to). Due to the high resistance of the Gigaseal, a current that flows through an ion channel within the patch must also flow through the contents of the pipette. An electrode connected to a sensitive amplifier is immersed in the pipette solution. This makes it possible to measure the activity of a single ion channel in the membrane of the patch. Both the cell membrane, of which the patch is a component, and the interior of the cell remain intact in this configuration.
The patch can be opened by further applying negative pressure to the end of the pipette or short pulses of electrical voltage on the electrode in the pipette, while the Gigaseal remains intact. There is now continuity between the inside of the pipette and the inside of the cell, while both are isolated from the external solution by the high resistance of the Gigaseal. This configuration of the patch clamp technique is called whole-cell configuration designated (engl. Whole cell - whole cell). In this configuration, it is derived from the entire cell membrane. Since the pipette solution fills the interior of the cell, its composition must be similar to the cytosol . At the same time, this configuration offers the possibility of manipulating the cell from the inside using the pipette solution.
If, after reaching the cell-attached configuration, the patch is not opened, but the pipette is gently removed from the cell, the part of the membrane located under the pipette tip detaches from the cell and remains on the pipette. The previously inner side of this membrane piece now points outwards into the bath solution, while the previously outer side of the membrane piece is located inside the pipette. This is the so-called inside-out configuration. Similar to the cell-attached configuration, it enables the measurement of individual ion channels in the membrane piece at the pipette tip. In contrast to this, however, in the inside-out configuration the environment on the inside of the membrane can be manipulated. If the pipette is filled with a solution that simulates the extracellular environment, the behavior of the ion channels depending on the composition of the cytosol can be examined. There is also the option of examining single ion channels using the outside-out configuration. The patch bulges out of the pipette opening and the outer side of the membrane is now in the bath solution. In this case, the milieu of the extracellular space can be modulated while the cytosolic milieu is retained.
With this method it is even possible to measure the function of cells in their natural environment in the living organism ( in vivo ).
Planar patch clamp
Planar Patch Clamp is a new method that was developed to increase the throughput in electrophysiology , among other things to meet the growing need for patch clamp measurements in pharmaceutical research .

Instead of positioning a pipette on an adherent cell , the cell suspension is pipetted onto a chip into which a microstructured opening (aperture) has been made.

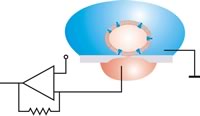
A single cell is then sucked onto the hole with negative pressure and an electrically tight connection is established between the cell and the glass (Gigaseal). The planar geometry offers several advantages compared to the conventional patch clamp method, such as: B. the integration of microfluidic channels allows the automated addition of active ingredients, as is required in pharmaceutical research . The system is accessible for optical and scanning probe microscopy procedures. The perfusion of Intrazellulärseite is much easier to do, which drugs can be better examined that act intracellularly. No special training is required to carry out the measurements. Non-adherent cells (such as red blood cells ), which are classically very difficult to examine, are much easier to patch in planar fashion. The measurement setups can be miniaturized. While a classic setup can easily fill up half a laboratory space, a planar setup can also be implemented as a tabletop device.
The advantages are offset by some disadvantages, such as: B. the adhesion of cells such as neurons or macrophages to the surface on which they were cultivated. In the case of enzymatic detachment, it must be ensured that the proteases do not affect the channel proteins on the cell surface. Adherent cells cannot be patched under strictly physiological conditions. Planar patch systems are therefore most widespread in transfected cell cultures . Since the cells have to be separated, measurements on tissue sections are not possible. It must be ensured that the cell suspension is homogeneous, since the patched cell is selected “blindly” from the ensemble. The cell cannot be selected based on its shape or a fluorescent label . Despite the extensive miniaturization, the acquisition costs per measuring channel are in a similar range as with the conventional patch clamp. Highly parallel systems are therefore only worthwhile with a correspondingly high number of passes.
See also
literature
- E. Neher, B. Sakmann: Research into cell signals with the patch-clamp technique. Spectrum of Science, May 1992, pp. 48-56 .
- Erwin Neher: Ion Channels For Communication Between and Within Cells. Nobel Prize Lecture Dec. 1991. (PDF)
- B. Sakmann, E. Neher: Single-Channel Recording. Springer, 1995, ISBN 1-4615-7858-2 .
- M. Numberger, A. Draguhn: Patch-Clamp Technique. Spectrum Akademischer Verlag, Heidelberg 1996, ISBN 3-8274-0023-6 .
- B. Hille: Ion channels in excitable membranes. , 3rd edition, Sunderland 2001, ISBN 0-87893-321-2 .
Web links
- DC Ogden: Microelectrode techniques, The Plymouth workshop handbook . 2nd edit. Company of Biologists, Cambridge 1994, ISBN 0-948601-49-3 . (English, PDF).
Individual evidence
- ↑ E. Neher, B. Sakmann. Single-channel currents recorded from membrane of denervated frog muscle fibers. In: Nature . 260, 1976, pp. 799-801.
- ↑ Information from the Nobel Foundation on the 1991 award ceremony to Erwin Neher and Bert Sakmann (English)
- ↑ OP Hamill, E. Neher, B. Sakmann, FJ Sigworth: Improved patch-clamp techniques for high-resolution current recordings from cells and cell-free membrane patches. In: Pflügers Arch . 391, 1981, pp. 85-100.
- ↑ KS Cole: Mostly Membranes . In: Annu Rev Physiol . 41, 1979, pp. 1-24.
- ↑ RC Bean, WC Shepherd, H. Chan, JT Eichler: Discrete conductance fluctuations in lipid bilayer protein membranes. In: J. Gen. Physiol. 53, 1969, pp. 741-757, doi : 10.1085 / jgp.53.6.741 .
- ↑ SB Hladky, DA Haydon: Discreteness of conductance change in bimolecular lipid membranes in the presence of certain antibiotics . In: Nature. 225, 1970, pp. 451-453, doi : 10.1038 / 225451a0 .
- ^ Sake Vogelzang: The state of plasma membrane polarization in plant cells. Proefschrift, Rijksuniversiteit Groningen, 1996.
- ^ Christian Schmidt, Michael Mayer, Horst Vogel: A Chip-Based Biosensor for the Functional Analysis of Single Ion Channels13 . In: Angewandte Chemie . tape 112 , no. 17 , 2000, pp. 3267-3270 , doi : 10.1002 / 1521-3757 (20000901) 112: 17 <3267 :: AID-ANGE3267> 3.0.CO; 2-1 .
- ↑ Jan C. Behrends, Niels ready: Planar Patch Clamping. In: Neuromethods. Vol. 38, pp. 411-433 ( PDF ).
- ↑ Small holes, big impact - cell physiology in chip format . List of German Future Prize nominees 2007, accessed on April 18, 2010.
- ↑ Ion channel measurements in high throughput - from a university laboratory to a global player . List of German Future Prize nominees 2014, accessed on March 6, 2015.