neutrino
neutrino |
|
---|---|
classification | |
Elementary particle fermion lepton |
|
properties | |
electric charge | neutral |
Dimensions | <2 · 10 −36 kg |
Resting energy | <1.1 eV |
Spin | 1/2 |
Interactions |
weak gravitation |
Neutrinos are electrically neutral elementary particles with a very low mass . In the standard model of elementary particle physics, there are three types ( generations ) of neutrinos: electron, muon and tau neutrinos. Each neutrino generation consists of the neutrino itself and its anti-neutrino . The name neutrino was suggested by Enrico Fermi for the particle postulated by Wolfgang Pauli and means (according to the Italian diminutive ino ) small neutron .
When the neutrinos interact with matter, unlike the other known elementary particles, only processes of weak interaction take place. In comparison to the electromagnetic and strong interaction , reactions take place very rarely. That is why a beam of neutrinos also goes through great thicknesses of matter - e.g. B. through the whole earth - even if with a certain weakening. The detection of neutrinos in experiments is correspondingly complex.
According to the place of origin of the neutrinos observed in neutrino detectors, a distinction can be made between
- cosmic neutrinos (space)
- solar neutrinos (sun)
- atmospheric neutrinos (earth's atmosphere)
- Geoneutrinos (Earth's interior)
- Reactor neutrinos (nuclear reactors)
- Neutrinos from accelerator experiments
Research history

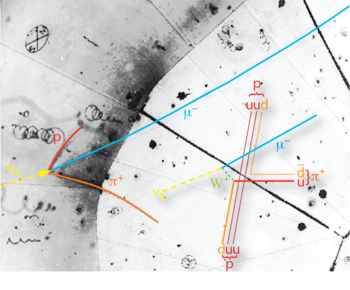
During the radioactive beta-minus decay , only one emitted electron was initially observed. Together with the remaining core, it seemed to be a two-body problem (see also kinematics (particle processes) ). This could only explain the continuous energy spectrum of the beta electrons if one assumed a violation of the law of conservation of energy . This led Wolfgang Pauli to adopt a new elementary particle that - unobserved by the detectors - is emitted from the nucleus at the same time as the electron. This particle carries away part of the energy released during the decay. In this way, the electrons of the beta radiation can receive different amounts of kinetic energy without the conservation of energy being violated.
In a letter dated December 4, 1930, Pauli proposed this hypothetical particle, which he initially called a neutron. Enrico Fermi , who worked out a theory about the basic properties and interactions of this particle, renamed it to neutrino (Italian for "small neutron", "little neutron") in order to avoid a name conflict with the now known neutron . It was not until 1933 that Pauli presented his hypothesis to a wider audience and asked about possible experimental evidence. Since the neutrino did not generate a signal in the usual particle detectors, it was clear that it would be extremely difficult to detect.
In fact, the first observation was only made 23 years later, in 1956, on one of the first large nuclear reactors with the Cowan pure neutrino experiment . On June 14, 1956, the researchers sent Wolfgang Pauli a telegram to Zurich with the message of success. A nuclear reactor emits neutrinos (more precisely: electron antineutrinos) with a much higher flux density than could be achieved with a radioactive preparation due to the beta decay of the fission products . Reines and Cowan used the following particle reaction (so-called inverse beta decay) to detect the antineutrinos:
An antineutrino meets a proton and creates a positron and a neutron. Both of these reaction products are comparatively easy to observe. For this discovery, Reines received the Nobel Prize in Physics in 1995 .
The muon neutrino was discovered in 1962 by Jack Steinberger , Melvin Schwartz and Leon Max Lederman with the first neutrino beam produced at an accelerator. They generated the neutrino beam by letting a high-energy pion beam travel so far that some of the pions (around 10%) had decayed into muons and neutrinos. With the help of a massive, approximately 12 m thick steel shield, which stopped all particles except the neutrinos from the mixed particle beam of pions, muons and neutrinos, they were then able to obtain a pure neutrino beam. For this they received the 1988 Nobel Prize in Physics. With the muon neutrino, a second generation of neutrinos became known, which is the analogue of the electron neutrino for muons . For a short time the term neutretto was used for the muon neutrino ( -etto is also an Italian diminutive ), but it was not widely used. When the tauon was discovered in 1975 , physicists also expected a corresponding generation of neutrinos, the tauon neutrino. The first signs of its existence were given by the continuous spectrum in the Tauon decay, similar to the beta decay. In 2000, the tau neutrino was then directly detected for the first time in the DONUT experiment.
The LSND experiment in Los Alamos, which ran from 1993 to 1998 , was interpreted as an indication of the existence of sterile neutrinos , but it was controversial. After the KA rlsruhe- R utherford- M ittel- E nergie- N eutrino- ( KARMEN ) experiment under the leadership of the Karlsruhe Research Center at the British Rutherford Laboratory could not reproduce the results, this interpretation has been valid since 2007 through the first results of MiniBooNE ( miniature booster neutrino experiment at the Fermi National Accelerator Laboratory ) as open.
In neutrino research of the 21st century, four scientists have been awarded the Nobel Prize in Physics (2002 and 2015) and five teams of scientists have been awarded the Breakthrough Prize in Fundamental Physics 2016.
properties
Three generations of neutrinos and antineutrinos
Three generations of leptons are known. Each of them consists of an electrically charged particle - electron , muon or tauon - and an electrically neutral neutrino, electron neutrino ( ), muon neutrino ( ) or tau or tauon neutrino ( ). Then there are the corresponding six antiparticles . All leptons have a spin ½.
According to more recent findings, neutrinos can transform into one another. This leads to a description of the neutrino species as three different states , and , each with a different, sharply defined (but still unknown) mass. The observable electron, muon and tau neutrinos - named after the charged lepton with which they occur together - are quantum mechanical superpositions of these three masses of their own . The relationship between the flavor eigenstates ( , , ), and the mass eigenstates ( , , ) is represented by a mix matrix, the PMNS matrix .
The number of types of neutrinos with a mass less than half the mass of the Z boson was determined in precision experiments and the like. a. determined to be exactly three at the L3 detector at CERN .
There is currently no evidence of neutrino-free double beta decay . Earlier work suggesting this has been refuted by more accurate measurements. A neutrino-free double beta decay would mean that either the conservation of the lepton number is violated or the neutrino is its own antiparticle . In the description of quantum field theory this would mean (in contradiction to the current standard model ) that the neutrino field would not be a Dirac spinor , but a Majorana spinor .
The physicists Lee and Yang initiated an experiment to study the spins of neutrinos and antineutrinos. This was carried out in 1956 by Chien-Shiung Wu and brought the result that parity maintenance does not apply without exception:
The neutrino turned out to be "left-handed", its spin is opposite to its direction of movement (antiparallel; see handedness ). This enables an objective explanation from left and right . In the area of weak interaction, not only the electrical charge but also the parity, i.e. the spin, must be swapped when moving from a particle to its antiparticle . The weak interaction differs from the electromagnetic interaction in that the weak isospin is linked to the right- or left-handedness of a particle:
- in the case of leptons and quarks , only the left-handed particles and their right-handed antiparticles have a weak isospin other than zero.
- In contrast, the right-handed particles and their left-handed antiparticles are inert to weak interactions with W bosons ; this phenomenon is called maximum parity violation .
This also makes it understandable that neutrinos could be their own antiparticles, although neutrinos and antineutrinos behave differently in the experiment: The particles known as antineutrinos from the experiment would simply be neutrinos whose spin is parallel to the direction of movement. The direction of movement of the neutrinos cannot simply be reversed experimentally; In addition, it is currently not possible to carry out experiments in which a neutrino is overtaken by a faster particle and interacts with it, so that the direction of movement in the reference system of the center of interaction is opposite to the direction of movement in the reference system of the laboratory.
Neutrino mass
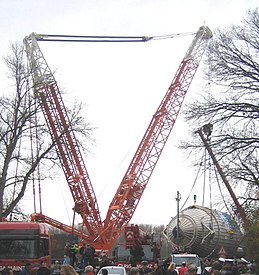
In the standard model of particle physics , neutrinos have no mass. There are extensions to the Standard Model and also some Great Unified Theories that predict non-zero mass.
Methods for determining the neutrino mass fall into four groups:
- direct determination of the mass from the missing energy during beta decay
- the observation of neutrino oscillations , i.e. conversions of one type of neutrino into another
- the search for neutrino-free double beta decays
- indirect conclusions from other observations, especially from observational cosmology
All published results are evaluated by the Particle Data Group and are incorporated into the annual Review of Particle Physics .
Direct measurements of the end point of the beta spectrum of tritium could by 2006 the possible mass of the neutrino electron with 2 eV / c ² restrict upward. It is hoped that a better upper limit will be achieved through more precise measurements of the KATRIN experiment at the Karlsruhe Institute of Technology , which is supposed to reach an upper limit of 0.2 eV / c² . The previous measurements could not rule out that the lightest neutrino is massless, and this is not expected without an improvement in the measurement accuracy by several orders of magnitude. In 2019 the upper limit was improved to 1.1 eV.
The observation of neutrino oscillations is an indirect measurement of mass differences between different neutrinos. They prove that neutrinos actually have a very small mass different from zero (compared to the associated charged leptons). The very small mass differences obtained in this way also mean that the above mass limit for electron neutrinos is also the limit for all types of neutrinos.
The hypothetical neutrino-less double beta decay is only possible if the neutrinos are their own antiparticles. Then, with the simultaneous beta decay of 2 neutrons in an atomic nucleus, sometimes 2 virtual neutrinos are annihilated instead of 2 (real) neutrinos being emitted. Since the neutrinos themselves are hardly measurable, the total energy of the 2 electrons produced in the process is measured: If neutrino-free decays occur, the total electron energy spectrum has a local maximum close to the decay energy, because almost all of the decay energy is now dissipated by the electrons (a small residue is converted into kinetic energy of the atomic nucleus).
The cosmological approach to the determination of the neutrino masses is based on the observation of the anisotropy of the cosmic background radiation by WMAP and other observations that determine the parameters of the lambda CDM model , today's standard model of cosmology. Due to the influence that neutrinos have on structure formation in the universe and on primordial nucleosynthesis , the upper limit for the sum of the three neutrino masses can be assumed to be 0.2 eV / c² (as of 2007) .
For their discovery of neutrino oscillations, Takaaki Kajita and Arthur B. McDonald received the 2015 Nobel Prize in Physics .
speed
Due to their low mass, it is expected that neutrinos generated in particle-physical processes move at almost the speed of light in a vacuum . The speed of neutrinos was measured in several experiments and a correspondence within the measurement accuracy with the speed of light was observed.
The measurement of the neutrino mass, neutrino velocity and neutrino oscillations also represent possibilities to check the validity of the Lorentz invariance of the special theory of relativity . Measurement results of the OPERA experiment in 2011, according to which neutrinos should have moved faster than light , could be traced back to measurement errors. A new measurement by ICARUS and a new analysis of the OPERA data have shown that they correspond to the speed of light.
Penetration ability
The ability to penetrate depends on the energy of the neutrinos. With increasing energy, the cross-section of the neutrinos increases and the mean free path decreases accordingly.
Example:
The mean free path of neutrinos with an energy of 10 3 TeV when interacting with the earth is in the range of the earth's diameter. This means that almost two thirds of these neutrinos interact when flying across the earth, while a good third flies through the earth. At 11 MeV, the mean free path in lead is already 350 billion kilometers, and on average around three out of a billion neutrinos would interact in the earth, while the rest would fly through unhindered.
For comparison:
The largest particle accelerator in the world, the Large Hadron Collider , generates particles with an energy of 6.5 TeV per nucleon , the sun mainly produces neutrinos with energies below 10 MeV.
An overview of the cross-section of neutrinos for different reactions and energies, published in 2013, is available on the Internet.
Decays and reactions

Processes with neutrinos take place via the weak interaction . Neutrinos are also subject to gravity; but this is so weak that it has practically no meaning. Like any weak interaction, neutrino processes can be divided into two categories:
- Charged stream
- An elementary particle couples to a neutrino via an electrically charged W boson . Here the particles involved transform into others. The exchange boson is positively or negatively charged depending on the reaction, so the charge is retained. Elastic scattering can also proceed in this way. Because the particles are the same at the beginning and the end, they can usually be described simply as a classic scattering.
- Neutral electricity
- An elementary particle couples to a neutrino via an electrically neutral Z boson . The particle flavors involved are retained and the reaction is like an elastic collision that can take place with any leptons or quarks. If the energy transfer is large enough, particle conversions can then take place at the atomic nuclei that are hit.
Decays
The first known processes in which neutrinos participate were the radioactive beta decays . During the β - (beta minus) decay, a neutron changes into a proton and an electron and an electron antineutrino are sent out. One of the two down quarks of the neutron emits the intermediate vector boson W - and thus turns into an up quark. The W - boson then breaks down into an electron and an electron antineutrino. So it is the “charged current”. This decay occurs, for example, with free neutrons, but also with atomic nuclei with a large excess of neutrons .
- A nuclide passes over
into the daughter nucleus with an atomic number higher by 1 , sending out an electron and an electron antineutrino .
Conversely, in the β + - (beta-plus) decay, a proton is converted into a neutron, and in the decay of the W + boson, a positron and an electron neutrino are emitted. The process occurs when there is an excess of protons in the nucleus. Since the reaction products are heavier than the original proton, the mass difference must be applied from the binding energy of the nucleus.
With the emission of a positron and an electron neutrino , a nuclide changes into a daughter nucleus with an ordinal number lower by 1.
Reactions
Important sources of neutrinos are also cosmic nuclear fusion processes , for example in the sun . One example is the proton-proton reaction , which is particularly important for small stars. Two hydrogen nuclei fuse at extremely high temperatures to form a deuterium nucleus; as a result of the conversion of a proton into a neutron, a positron and an electron neutrino are released.
In terms of particle physics, this reaction is equivalent to β + decay. But it is far more important for neutrino research because a lot of neutrinos are generated in the sun. Electron neutrinos are also formed in another fusion process, the Bethe-Weizsäcker cycle , in the sun and heavier stars. The observation of the so-called solar neutrinos is important to understand their properties, details of the processes in the sun and the fundamental interactions of physics .
Reactions with a neutrino as the triggering collision partner are important as "reverse beta decay" for the detection of neutrinos, for example in the historical Cowan-Reines neutrino experiment :
- .
Neutrino research
Although the low reactivity of neutrinos makes it difficult to detect them, the penetration ability of neutrinos can also be used in research: neutrinos from cosmic events reach the earth , while electromagnetic radiation or other particles in interstellar matter are shielded.
astrophysics
First, neutrinos were used to study the interior of the sun . Direct optical observation of the core is not possible due to the diffusion of electromagnetic radiation in the surrounding plasma layers. The neutrinos, however, which are produced in large numbers in the fusion reactions in the sun's interior, only interact weakly and can penetrate the plasma practically unhindered. A photon typically takes a few thousand years to diffuse to the sun's surface; a neutrino only needs a few seconds for this.
Later neutrinos were also used to observe cosmic objects and events beyond our solar system. They are the only known particles that are not significantly affected by interstellar matter. Electromagnetic signals can be shielded from dust and gas clouds or covered by cosmic radiation when detected on earth . The cosmic radiation, in the form of super-fast protons and atomic nuclei, can not spread further than 100 megaparsecs due to the GZK cutoff (interaction with background radiation) . The center of our galaxy is also excluded from direct observation because of dense gas and countless bright stars. However, it is likely that neutrinos from the galactic center will be able to be measured on Earth in the near future.
Neutrinos also play an important role in the observation of supernovae , which release around 99% of their energy in a neutrino flash. The resulting neutrinos can be detected on earth and give information about the processes during the supernova. In 1987, neutrinos were detected by the 1987A supernova from the Large Magellanic Cloud : eleven in the Kamiokande , eight in the Irvine Michigan Brookhaven Experiment , five in the Mont Blanc Underground Neutrino Observatory, and possibly five in the Baksan detector. These were the first neutrinos to be detected that certainly came from a supernova, as this was observed with telescopes a few hours later.
Experiments such as IceCube , Amanda , Antares and Nestor aim to detect cosmogenic neutrinos. IceCube is currently (2018) the largest neutrino observatory .
Neutrino detectors
The IceCube experiment mentioned in the previous section on Astrophysics is a high-energy neutrino observatory with around 260 employees. It was completed in 2010 in the ice of the South Pole and has a volume of 1 km³. The reaction of the high-energy neutrinos with the elementary particles of the ice is observed and evaluated with this detector.
Well-known neutrino detectors are still or on the one hand the radiochemical detectors (e.g. the chlorine experiment in the Homestake gold mine , USA or the GALLEX detector in the Gran Sasso tunnel in Italy), on the other hand the detectors based on the Cherenkov effect , here especially the Sudbury Neutrino Observatory (SNO) and Super-Kamiokande . They detect solar and atmospheric neutrinos and allow u. a. the measurement of neutrino oscillations and thus inferences about the differences of the neutrino masses, since the reactions taking place inside the sun and thus the neutrino emission of the sun are well known. Experiments such as the Double Chooz experiment or the KamLAND detector, which has been working since 2002 at the Kamioka Neutrino Observatory, are able to detect geoneutrinos and reactor neutrinos via inverse beta decay and provide complementary information from a range that is not covered by solar neutrino detectors .
One of the currently largest neutrino detectors, called MINOS, is located underground in an iron mine in the USA, 750 kilometers from the Fermilab research center. A neutrino beam is emitted from this research center in the direction of the detector, where it is then counted how many of the neutrinos transform during the underground flight.
The CNGS experiment (CERN Neutrinos to Gran Sasso ) has been investigating the physics of neutrinos since 2007. For this purpose, a neutrino beam is sent from CERN over a distance of 732 km through the earth's crust to the Gran Sasso Laboratory in Italy , where it is detected. Some of the muon neutrinos transform into other types of neutrinos (almost exclusively tau neutrinos), which are detected by the OPERA detector (Oscillation Project with Emulsion-tRacking Apparatus). For the related speed measurements see the section Speed .
application
Researchers at Sandia National Laboratories want to use the evidence of antineutrinos to measure the production of plutonium in nuclear reactors , so that the IAEA no longer has to rely on estimates and no one can divert anything for the construction of nuclear weapons . Because of the high production rate of antineutrinos in nuclear reactors, a detector with 1 m³ of detector liquid in front of the nuclear power plant would be sufficient.
In 2012, researchers at the University of Rochester and North Carolina State University succeeded for the first time in sending a message through solid matter using neutrinos. A proton accelerator generated a neutrino beam, which was detected by a neutrino detector 100 meters below the earth.
literature
- Kai Zuber: Neutrino Physics. Institute of Physics Publishing, Bristol / Philadelphia 2004, ISBN 0-7503-0750-1 .
- Konrad Kleinknecht : Detectors for particle radiation. 4th edition. Teubner Verlag, Wiesbaden 2005, ISBN 3-8351-0058-0 .
- Heinrich Päs: The perfect wave. With neutrinos to the limits of space and time or why particle physics is like surfing. Piper, Munich 2011, ISBN 978-3-492-05412-6 .
- Norbert Schmitz : Neutrino Physics. Teubner Verlag, Stuttgart 1997, ISBN 3-519-03236-8 , doi: 10.1007 / 978-3-322-80114-2 .
- Y. Suzuki, M. Nakahata, S. Moriyama (Eds.): The Fifth International Workshop on Neutrino Oscillations and Their Origin: Proceedings of the Fifth International Workshop. World Scientific Publishing, 2005, ISBN 978-981-256-362-0 .
- Jennifer A. Thomas: Neutrino oscillations - present status and future plans. World Scientific, Singapore 2008, ISBN 978-981-277-196-4 .
- Carlo Giunti et al: Fundamentals of neutrino physics and astrophysics. Oxford University Press, Oxford 2007, ISBN 978-0-19-850871-7 .
- Frank Close : Neutrino. Spektrum Akademischer Verlag, Heidelberg 2012, ISBN 3-8274-2940-4 .
- Pasquale Migliozzi: Measurement of the neutrino velocity with the OPERA detector in the CNGS beam. February 2, 2012 (PDF; 5.2 MB).
Web links
- Neutrino Unbound (most extensive online archive, all important papers)
- The Ultimate Neutrino Page (extensive online archive)
- Various neutrino experiments (links, English)
- DESY - The Solar Neutrino Problem (English)
- Neutrinos: Generation and Detection. Fundamentals of particle physics.
Videos
- Neutrinos - secret writing of the cosmos. Lecture by Christian Spiering ( German Electron Synchrotron , Zeuthen) in April 2010
- What are neutrinos? from the alpha-Centauri television series(approx. 15 minutes). First broadcast on Oct 13, 2002.
- Where are the neutrinos? from the alpha-Centauri television series(approx. 15 minutes). First broadcast on Jan 19, 2003.
Individual evidence
- ↑ a b KATRIN Collaboration : An improved upper limit on the neutrino mass from a direct kinematic method by KATRIN . September 13, 2019, arxiv : 1909.06048 .
- ↑ Measurement of the multi-TeV neutrino interaction cross-section with IceCube using Earth absorption . In: Nature . tape 551 , no. 7682 , 2017, p. 596–600 , doi : 10.1038 / nature24459 .
- ↑ Recent happenings in high-energy physics . In: New Scientist . Reed Business Information, January 21, 1971, p. 106 (English, books.google.com ).
- ↑ Pauli's letters. (PDF; 104 kB), evening lecture on the history of neutrino physics, given by Prof. Dr. Mößbauer at the Technical University of Munich.
- ↑ Claus Grupen, Boris Shwartz: Particle Detectors (Cambridge Monographs on Particle Physics, Nuclear Physics and Cosmology). Cambridge University Press 2008, ISBN 978-0-521-84006-4 .
- ↑ CL Cowan, Jr., F. Reines, FB Harrison, HW Kruse, AD McGuire: Detection of the Free Neutrino: A Confirmation . In: Science . 124, 1956, pp. 103-104. doi : 10.1126 / science.124.3212.103 .
- ↑ Frederick Reines, Clyde L. Cowan, Jr .: The Neutrino . In: Nature . 178, No. 4531, 1956, p. 446. bibcode : 1956Natur.178..446R . doi : 10.1038 / 178446a .
- ↑ 1953-1956 The Reines-Cowan Experiments: Detecting the Poltergeist. (PDF; 664 kB), accessed on June 21, 2011.
- ↑ Leon Ledermann, Dick Teresi: The creative particle . 1st edition. C. Bertelsmann Verlag GmbH, Munich 1993, ISBN 3-570-12037-6 , Die Mord-GmbH and the 2-Neutrino-Experiment, p. 391–393 (English: The God Particle . New York 1993. Translated by Heinrich Peitz, first edition: Houghton Mifflin Company).
- ↑ MiniBooNE Collaboration: A Search for Electron Neutrino Appearance. In: Physical Review Letters , Volume 98, 2007, 231801, (PDF; 194 kB).
- ↑ Neutrino Physics: News from the ghost particles. ( Memento of the original from July 23, 2013 in the Internet Archive ) Info: The archive link was inserted automatically and has not yet been checked. Please check the original and archive link according to the instructions and then remove this notice.
- ↑ W.-M. Yao et al: Particle Data Group. In: Journal of Physics. G 33, 1 (2006).
- ↑ Davide Castelvecchi: Physicists close in on elusive neutrino's mass . In: Nature . September 17, 2019, doi : 10.1038 / d41586-019-02786-z .
- ↑ U. Seljak, A. Slosar, P. McDonald: Cosmological parameters from combining the Lyman-alpha forest with CMB, galaxy clustering and SN constraints. In: JCAP. 0610: 014 (2006), online.
- ↑ M. Cirelli and A. Strumia: Cosmology of neutrinos and extra light particles after WMAP3. In: JCAP. 0612: 013 (2006), online.
- ↑ Hirotaka Sugawara, Hiroyuki Hagura, Toshiya Sanami: Destruction of Nuclear Bombs Using Ultra-High Energy Neutrino Beam. (PDF; 285 kB). In: arxiv.org. June 2003, accessed March 15, 2012.
- ↑ From eV to EeV: neutrino cross-sections Across Energy Scales. (PDF; 2.9 MB).
- ↑ K. Hirata et al .: Observation of a Neutrino Burst from the Supernova SN 1987a. In: Physical Review Letters , Volume 58, 1987, pp. 1490-1493. doi: 10.1103 / PhysRevLett.58.1490 .
- ↑ RM Bionta et al .: Observation of a Neutrino Burst in Coincidence with Supernova SN 1987a in the Large Magellanic Cloud. In: Physical Review Letters , Volume 58, 1987, p. 1494. doi: 10.1103 / PhysRevLett.58.1494 .
- ↑ M. Aglietta et al .: On the Event Observed in the Mont Blanc Underground Neutrino Observatory during the Occurrence of Supernova 1987a. In: EPL - A letters journal exploring the frontiers of physics. Les-Ulis, Vol. 3, 1987, pp. 1315-1320. doi: 10.1209 / 0295-5075 / 3/12/011 .
- ↑ EN Alexeyev et al in: Soviet physics. (Sov. JETP Lett.). New York, vol. 45, 1987, p. 461.
- ↑ Kai Zuber: Neutrino Physics. Institute of Physics Publishing, Bristol / Philadelphia 2004, ISBN 0-7503-0750-1 .
- ↑ Homepage of the Kamland detector.
- ↑ Antineutrinos monitor plutonium production.
- ↑ Researchers Send “Wireless” Message Using Elusive Particles. At: rochester.edu. March 14, 2012.