Hydrogen production
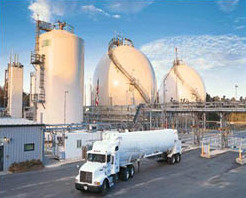
With hydrogen production , the provision of molecular is hydrogen (H 2 hereinafter). Natural gas (especially methane (CH 4 )), other hydrocarbons , biomass , water (H 2 O) and other hydrogen-containing compounds can be used as raw materials . Chemical energy or externally supplied electrical, thermal or solar energy is used as the energy source .
Use of hydrogen
Hydrogen is primarily used in the chemical industry, for example for the production of nitrogen fertilizers or for the cracking of hydrocarbons in petroleum refineries . It is important as an intermediate stage in chemical processes for the production of synthetic fuels such as gas-to-liquid (GtL), coal-to-liquid (CtL) and biomass-to-liquid (BtL), which, among other things, require the production of a hydrogen-rich synthesis gas . In view of the increasing importance of renewable energies , energy storage systems are necessary so that production and demand times can be coordinated. One option for electricity storage could be the electrolysis of water to form the gases hydrogen and oxygen (O 2 ), which can be stored and later converted into electricity. As part of a so-called hydrogen economy, the hydrogen could also be used directly. The same PEM / SPE technology is used in reverse form to produce molecular hydrogen for use in medicine and health care.
Although hydrogen is a colorless gas, it has become common practice in the context of the climate and carbon dioxide debate to cover the hydrogen with colors, depending on the manufacturing process used.
- Green hydrogen: Manufacturing processes based on water splitting can produce hydrogen CO 2 -free if they are operated with climate-neutral electricity. Hydrogen produced in this way is sometimes called "green hydrogen". The large-scale implementation of such processes is often seen as a key technology for combating climate change. On the other hand, it is disputed what energy and cost efficiency can be achieved with them, how great the overall benefit of this technology would be even in a fully developed form, and whether battery technology, for example, would be preferable for most sectors.
- Gray hydrogen: Traditional processes usually split natural gas into hydrogen and CO 2 under heat . The CO 2 enters the atmosphere unused. Approximately 10 tons of CO 2 are produced per ton of hydrogen produced . Hydrogen produced in this way is called "gray hydrogen".
- Blue hydrogen: “Blue” is the name given to hydrogen, which is traditionally produced like gray, but in which the resulting CO 2 is bound and not released into the atmosphere.
- Turquoise hydrogen: The production of hydrogen by thermal splitting of methane does not produce CO 2 , but solid carbon. This hydrogen is called "turquoise hydrogen". However , this process is only CO 2 -neutral if the thermal energy comes from renewable energy sources.
production method
Hydrogen production processes are explained below, some of which are used on an industrial scale, but some of which are still under development. A distinction is made between processes that use hydrocarbons and similar compounds and those that split hydrogen from water using various methods.
Use of hydrocarbons
When using hydrocarbons, but also coal and biomass, the raw material provides the energy required for the process. The hydrogen can also be partially bound in the raw material or added in the form of water. An exception is the Kværner process, in which the required energy is mainly supplied from outside.
Steam reforming
In steam reforming , hydrogen is generated from hydrocarbons in two process steps. The following raw materials can be used: natural gas, biomass, but also longer-chain hydrocarbons from crude oil such as the middle gasoline fraction . This process is established and implemented in plants with capacities of up to 100,000 m³ / h.
In the first step, long-chain hydrocarbons are split into methane, hydrogen, carbon monoxide and carbon dioxide in a pre-reformer with the addition of steam at a temperature of around 450–500 ° C and a pressure of around 25–30 bar . This preliminary stage avoids excessive coking of the reformer catalyst. In the second step, the methane is converted in the reformer at a temperature of 800 to 900 ° C and a pressure of around 25-30 bar over a nickel catalyst with water to form carbon monoxide and hydrogen. The second step is usually preceded by a refining plant for gas processing, as catalysts are extremely sensitive to sulfur and halogen compounds , especially chlorine ( catalyst poisons ).
- general equation:
- Example Methane ;
The intermediate product carbon monoxide produced by incomplete conversion is then converted into carbon dioxide and hydrogen with the aid of the water gas shift reaction on an iron (III) oxide catalyst . In order to obtain high-purity hydrogen in the final step, pressure swing adsorption systems or lye absorption gas scrubbers are often used in practice , which filter out byproducts such as CO, CO 2 and CH 4 down to a few ppm .
Steam reforming is the most economical and widely used (~ 90%) method of producing hydrogen. Due to the use of fossil fuels, just as much of the greenhouse gas carbon dioxide CO 2 is released as when it is burned. The use of biomass can improve the climate balance, as only the carbon dioxide is released that the plants previously absorbed from the atmosphere while growing.
Partial oxidation
In the case of partial oxidation , the raw material, such as natural gas or a heavy hydrocarbon ( heating oil ), is converted substoichiometrically - i.e. under a lack of oxygen - in an exothermic process. Reaction products are mainly hydrogen and carbon monoxide:
- general reaction equation:
- Example: typical component of heating oil :
- Example: typical composition of coal :
Usually water is added in order to get both the extreme temperatures and the soot formation under control, so that one would have to speak of an autothermal reforming with little water. The partial oxidation is considered technically mature.
In coal-rich countries such as China or South Africa , coal can also be used as a starting material for this process, which is ground beforehand and mixed with water to form a suspension.
Autothermal reforming
The autothermal reforming is a combination of steam reforming and partial oxidation, by which the efficiency is optimized. For example, methanol, like any other hydrocarbon or any hydrocarbon mixture (natural gas, gasoline, diesel, etc.) can be used. The two processes are combined in such a way that the advantage of oxidation (provision of thermal energy) and the advantage of steam reforming (higher hydrogen yield) complement each other in an optimizing manner. This is done by precisely metering the air and water vapor supply. Particularly high demands are made of the catalysts used here, as they must promote both steam reforming with the water-gas shift reaction and partial oxidation.
Gas processing
If the product contains proportions of CO, the hydrogen yield can still be increased. After the reforming, the synthesis gas is processed further. The next step is the CO conversion by means of the water gas shift reaction . Followed by any necessary CO fine purification by preferential oxidation or selective methanation is CO chemically reacted or physically by pressure swing adsorption or a hydrogen permeable membrane made of a palladium - silver - alloy separated (PdAg). On an industrial scale, it is also possible to wash out CO 2 and H 2 S ( hydrogen sulfide ) with methanol ( Rectisol process ).
Kværner method
The process developed by the Norwegian company Kværner completely separates hydrocarbons in a plasma burner at 1600 ° C into activated carbon (pure carbon) and hydrogen.
- general reaction equation:
- Reaction equation for methane:
A pilot plant built in Canada in 1992 achieved an efficiency of almost 100% - but only under the condition that the waste heat generated is fully used. The energy content of the reaction products of this process is distributed around 48% on hydrogen, around 40% on activated carbon and around 10% on superheated steam.
Biomass
Biomass mostly consists mainly of carbohydrates and can be converted in processes similar to hydrocarbons. Another option could be anaerobic fermentation with the help of microorganisms.
Steam reforming
Biomass consists mainly of carbohydrates and other organic compounds containing hydrogen and carbon. These can be converted into molecular hydrogen by means of allothermal or autothermal steam reforming. Since biomass consists of about 40% oxygen, it almost gasifies by itself with only a little additional oxygen to carry out the endothermic reaction . It is therefore possible to achieve significantly higher levels of efficiency than, for example, when gasifying coal.
Pyrolysis and steam reforming
Another process combines pyrolysis and steam reforming . The first stage is pyrolysis, which produces primary gases, coke and methanol as end products. In a second sub-process, these are mixed with water vapor, which in turn creates a mixture of hydrogen, methane, carbon monoxide and carbon dioxide. In this second step, too, energy has to be supplied, and hydrogen is then obtained by steam reforming. This two-stage variant is mainly used for smaller systems.
According to the dena project GermanHy , large-scale biomass gasification may in future become an option for generating hydrogen from renewable energies. The efficiencies of the most important processes for converting biomass to hydrogen are in the range of 30 to 40 percent. There are as yet no known commercial plants for the production of hydrogen. However, the production of hydrogen from biomass is in competition with biomass liquefaction in addition to the direct energetic use of biomass (e.g. through wood chips ) . As an energy source, the liquid fuels obtained in this way have a higher energy density than hydrogen and are easier to handle.
fermentation
Under laboratory conditions, hydrogen can be obtained directly from biomass using anaerobic microorganisms. If mixed cultures are used for this , hydrogen production must be decoupled from the last link in the anaerobic food chain, methane production (methanogenesis). For reasons of reaction kinetics, the release of molecular hydrogen by microorganisms is only favored at very low hydrogen partial pressure. This pressure must therefore be kept low despite the absence of methanogenic microorganisms or sulfate-reducing or hydrogen-utilizing bacteria through the construction and operation of the bioreactor .
However, fermentative hydrogen production is relatively unfavorable in terms of energy. According to Thauer (1976), a maximum of 33% of the combustion heat from glucose can be stored in hydrogen in the way described . In comparison, methane fermentation can convert 85% of the energy from glucose into the fermentation product.
Water splitting
When water is split, water serves as a hydrogen supplier.
With the help of electricity, the energy carriers hydrogen and oxygen are generated from the water in an electrolyser . Various chemical processes that use electrolysis to generate other compounds can produce hydrogen as a by-product.
When using hydrocarbons and in electrolysis, solar energy is primarily used indirectly, as this is, for example, a prerequisite for the production of coal, crude oil and natural gas. But a more or less direct use of solar energy is also possible. In thermochemical processes for splitting water into hydrogen, very high temperatures are necessary, which are made possible, for example, by concentrating solar radiation. Biological processes are also being developed in which the splitting of water during photosynthesis can be used to generate hydrogen.
The photons of sunlight are used directly in the photocatalytic splitting of water . The photons generate electron-hole pairs, the energy of which can be used directly to break water down into its components.
Electrolysis of water
This form of conversion of water into hydrogen was first demonstrated by the German chemist Johann Wilhelm Ritter around 1800 . The reaction takes place in a vessel filled with conductive electrolytes ( salts , acids , bases ), in which there are two electrodes that are operated with direct current. Under alkaline conditions, the manufacturing process takes place in two partial reactions:
- Cathode:
- Anode:
In principle, electrons are given off at the anode, which are then taken up again by the cathode. In the overall reaction, water is converted into molecular hydrogen and molecular oxygen:
- Overall reaction:
The process only makes sense in terms of energy efficiency if the pure oxygen produced can also be used and is not simply released into the air. The energetic efficiency of the electrolysis of water is over 70%. Several plant manufacturers (e.g. Electrolyser Corp., Brown Boveri, Lurgi, De Nora, Epoch Energy Technology Corp.) offer large electrolyser devices with an even higher degree of efficiency (over 80%). MIT scientists have developed a catalyst that is said to increase the efficiency of the electrolysis of water to almost 100%.
One type of process is alkaline electrolysis , which is mainly used as an energy supplier in Norway and Iceland because of the low electricity prices of hydropower plants .
In contrast to the use of fossil fuels, no CO 2 is released during electrolysis . However, this only applies if the electricity used was not generated from fossil fuels. For hydrogen production, storage and subsequent reconversion, the efficiency in 2013 was a maximum of 43%. Sterner et al. indicate efficiency ranges between 34 and 44%. It is assumed that overall electrical efficiencies of a maximum of 49 to 55% can be achieved in perspective.
Chlor-alkali electrolysis
In chlor-alkali electrolysis , hydrogen and chlorine are produced as by-products. However, it is primarily used to obtain sodium hydroxide and potassium hydroxide from solutions of chlorides (e.g. table salt (NaCl)). These reactions take place at the two electrodes :
The process has been used on an industrial scale for decades. It makes economic sense where there is a need for alkalis (and possibly chlorine), but is not worthwhile for the purpose of hydrogen production alone.
Thermochemical processes
Thermal dissociation
Thermal dissociation describes the disintegration of molecules into their individual atoms through the action of heat. The direct splitting of water vapor into hydrogen and oxygen takes place above a temperature of 1,700 ° C. This happens, for example, in solar ovens . The resulting gases can be separated from one another with ceramic membranes . These membranes are permeable to hydrogen but not to oxygen. The problem with this is that very high temperatures occur and only expensive, heat-resistant materials can be used. For this reason, this process is not yet competitive.
Thermochemical process
A lowering of the temperature during thermal water splitting to below 900 ° C can be achieved through coupled chemical reactions. As early as the 1970s, various thermochemical cycle processes were proposed for coupling the heat from high-temperature reactors , some of which are also suitable for the use of concentrated solar radiation. From today's perspective, the highest system efficiencies and the greatest potential for improvements are found in an improved sulfuric acid-iodine process : iodine (I) and sulfur dioxide (SO 2 ) react with water at 120 ° C to form hydrogen iodide (HI) and sulfuric acid (H 2 SO) 4 ). After the reaction products have been separated, sulfuric acid is split into oxygen and sulfur dioxide at 850 ° C, hydrogen iodide is formed at 300 ° C and the starting product iodine. However, the high thermal efficiencies of the thermochemical cycle processes (up to 50%) must be compared with the still largely unsolved material and process engineering difficulties.
Metal oxide process
Many metal oxides split oxygen at very high temperatures and the resulting metal reacts with water at lower temperatures, recovering the oxide to produce hydrogen. More than 300 variants of these thermochemical processes are known. Some of them, for example the zinc-zinc oxide process or the cerium (IV) oxide-cerium (III) oxide process , are being examined as technologically promising (see also the Hydrosol project ):
Example (M: metal; M (II): metal of oxidation state II; O: oxygen; H: hydrogen):
- Cleavage step: M (II) O reduces + H 2 O → M (IV) O 2 oxidizes + H 2
- Regeneration step: M (IV) O 2 oxidizes → M (II) O reduces + 1/2 O 2
Photocatalytic water splitting
Photocatalysis uses sunlight and a catalyst to produce hydrogen. The advantage of photocatalysis over other techniques such as electrolysis is that the charge separation and splitting of the water from a material can be carried out at the same interface, which minimizes transmission losses and enables material savings to be achieved.
Photobiological production
In the photobiological production of hydrogen, sunlight can also be used as an energy source. The corresponding catalysts are produced by living organisms. To do this, bacteria must be cultivated in hydrogen bioreactors . By influencing the photosynthesis they carry out , energy is not stored in biomass , but used to split water into hydrogen and oxygen. This process could be CO 2 -neutral or almost CO 2 -neutral. So far, however, no economic implementation is possible.
See also
- Hydrosol project
- Hydrogen Challenger
- List of hydrogen technologies
- Solar chemistry # Solar thermal hydrogen production
- Hydrogen storage
- Ortho and para hydrogen
Web links
- Fuel gas generation for fuel cells ( Memento from October 16, 2006 in the Internet Archive ) at Forschungszentrum Jülich
- Hydrogen production using an artificial bacteria-algae symbiosis (ArBAS) , project website of the TU Berlin
- Hydrogeit - hydrogen production
- Where will hydrogen come from in Germany by 2050? , Study as part of the GermanHy project ofthe German Energy Agency (dena), status August 2009, as a 62-page PDF
Individual evidence
- ↑ Introduction to Hydrogen. (No longer available online.) Archived from the original on March 1, 2017 ; accessed on February 28, 2017 . Info: The archive link was inserted automatically and has not yet been checked. Please check the original and archive link according to the instructions and then remove this notice. Link broken on October 21, 2018.
- ↑ H2 -Aqua Cell: Experience and knowledge about water and H2. Retrieved October 21, 2018 .
- ↑ Molecular Hydrogen (H2): A molecule with high therapeutic potential. Retrieved October 21, 2018 .
- ↑ a b Federal Ministry of Education and Research: A Little Hydrogen Color Theory. Retrieved June 15, 2020 .
- ↑ Green hydrogen as a climate protector: Der Sauberstoff on spiegel.de accessed on January 2, 2020
- ↑ Hydrogen: The Energy of the Future? Retrieved from br.de on January 2, 2020
- ↑ Where will hydrogen come from in 2050? Retrieved June 19, 2020 .
- ↑ Ulf Bossel, Theory and Practice, April 2006: Hydrogen does not solve energy problems , accessed on September 24, 2014
- ↑ Jan Rössler, Sven Messerer: Fuel cell technology - production of hydrogen. (PDF) 2005, p. 5 , archived from the original on October 4, 2012 ; accessed on January 16, 2015 (Darmstadt University of Applied Sciences, group work (?)): "In principle, electrons are given off at the anode and picked up again by the cathode."
- ↑ MIT claims 24/7 solar power , July 31, 2008, accessed October 19, 2011
- ↑ Volker Quaschning , Regenerative Energy Systems. Technology - calculation - simulation . 8th updated edition. Munich 2013, p. 373.
- ↑ Energy-economic and ecological evaluation of a wind gas offer p. 18 ( Memento of the original from December 24, 2014 in the Internet Archive ) Info: The archive link was inserted automatically and has not yet been checked. Please check the original and archive link according to the instructions and then remove this notice. . Fraunhofer IWES . Retrieved November 14, 2014.
- ^ Dan Gao, Dongfang Jiang, Pei Liu, Zheng Li, Sangao Hu, Hong Xu, An integrated energy storage system based on hydrogen storage: Process configuration and case studies with wind power . Energy 66 (2014) 332-341 doi : 10.1016 / j.energy.2014.01.095 .
- ^ Report of the Federal Environment Agency on the use of microalgae ( Memento of September 30, 2007 in the Internet Archive ), last update on March 16, 2009, accessed on March 30, 2010