Wittig reaction
The Wittig reaction is an organic chemical reaction that serves to create C = C bonds and is named after its discoverer Georg Wittig . It can be used to olefinate carbonyl compounds ( aldehydes or ketones ) with phosphorylides to alkenes by substituting the carbonyl oxygen :
As an olefin formation reaction, it is of great importance in laboratories and industry. The Wittig reaction is a widely applicable method for the synthesis of olefins and tolerates a wide range of functional groups . Usually, only very acidic functional groups such as carboxylic acids or 1,3-dicarbonyl compounds prove to be disruptive to the Wittig reaction .
General
Both inter- and intramolecular variants are known in the Wittig reaction. The reaction is regioselective , that is, the newly formed carbon-carbon double bond is found in the place of the earlier carbon-oxygen double bonds. Aldehydes and ketones come into consideration as carbonyl components, aldehydes being more reactive and sterically less hindered ketones being able to be selectively olefinated compared to sterically hindered ketones. Carboxylic acid esters are almost inert in a Wittig reaction.
A disadvantage is the in principle little pronounced ( E , Z ) selectivity, which can, however, be controlled by suitable reaction conditions. Due to its high tolerance of functional groups, the Wittig reaction can also bring a large number of functional groups in the Wittig reagent itself.
The Wittig reaction is used in the synthesis of olefinic natural substances such as vitamins A and D, carotenoids ( β-carotene see below), squalene, unsaturated pheromones , insect hormones, odoriferous substances and prostaglandins .
history
discovery
In his experiments on the quaternization of the main group elements, Wittig obtained a zwitterion which exhibited remarkable properties.
In the presence of carbonyl compounds, they reacted smoothly with the carbonyl carbon to form a carbon-carbon double bond and triphenylphosphine oxide .
Wittig published this novel reaction, discovered in 1947 at the University of Tübingen , under the title About triphenyl-phosphine-methylene as olefin-forming reagents and henceforth called this reaction carbonyl-olefination . The name Wittig reaction later came into use. Today the triphenylalkylphosphonium salt is usually used as Wittig salt for this reaction and triphenylphosphine oxide is obtained as a by-product .
The Wittig reaction quickly proved to be very versatile and universally applicable. Georg Wittig was awarded the Nobel Prize for Chemistry in 1979 .
First industrial applications
BASF took note of the new method with enthusiasm. It made it possible to combine an already existing synthetic building block [derivative of (2 E , 4 E ) -3-methyl-5- (2,6,6-trimethylcyclohex-1-en-1-yl) pentane] with the easily accessible (2 E , 4 E , 6 E ) -2,7-dimethylocta-2,4,6-trienedial to link to β- carotene . A patent was filed (BASF) just three years after Wittig's publication.

Making the ylids
Trisubstituted phosphorus alkyls / aryls can be quaternized in an S N 2 reaction. For example, triphenylphosphine ( 1 ) reacts with ethyl bromide ( 2 ) to form a phosphonium salt via ethyltriphenylphosphonium bromide ( 3 ) to form an ylide ( 4 ) and a ylene:
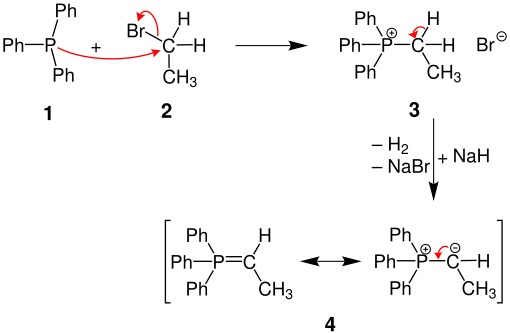
Triphenylphosphine ( 1 ) and ethyl bromide ( 2 ) are heated in benzene in an autoclave to 130 ° C. for 20 hours. The phosphonium salt precipitates in crystalline form on cooling (yield 90%).
Phosphines are good nucleophiles, but bad bases. For this reason, the E2 elimination competing with the S N 2 reaction is almost completely suppressed. Most primary and secondary alkyl halides can thus be converted into their phosphonium salts in good yields.
In contrast to the mostly isolated phosphonium halides, the ylides produced from them by deprotonation at the α-C atom are usually further converted directly.
The ethyltriphenylphosphonium bromide ( 3 ) is deprotonated with sodium hydride under inert gas in DMSO .
An externally neutral phosphorus compound is formed, which is called ylide ( 4 ) or phosphorane. These betaines can also be formulated as Ylene . Other common deprotonation reagents are phenyllithium or n - butyllithium .
A modern and simple method for deprotonating the phosphonium ion under lithium-free conditions is the use of potassium tert -butanolate in THF or, in the case of sterically hindered carbonyl groups, in toluene . The Wittig salt can be mixed dry with the base in equimolar proportions and mixed with the solvent, which is an alternative to the instant ylide that is easier to use . Phosphorus ylides can also be obtained by carbene addition to phosphines.
Mechanisms of the Wittig reaction
The mechanism of the Wittig reaction can be viewed in general or from a stereochemical point of view.
Wittig reaction, general
The following mechanism explains the general Wittig reaction, in which phosphorylides react with carbonyl compounds to form alkenes.
The negatively charged carbon atom of the phosphorylid 5 attacks the carbonyl compound of the starting material and a phosphorus betaine 6 is formed , which further reacts to form oxaphosphetane 7 , a four-membered ring. This finally breaks down into alkene 8 and triphenylphosphine oxide 9 due to the strong phosphorus-oxygen double bond.
Wittig reaction, stereochemical aspects
Overall, the mechanism has not yet been precisely clarified, or it proceeds differently depending on the substituent. For the most part, the reaction takes place in several stages; individual interconnections can be isolated. In the case of reactive ylidene / carbonyl compounds, the attack of the carbanion and formation of the oxaphosphetane occurs in concert according to the Woodward-Hoffmann rules . There is also evidence that in the presence of very large substituents (high steric hindrance), the reaction by free-radical levels (SET s ingle e Lectron t ransfer) extends.
When the betaine is formed, it is determined whether the alkene is ( E ) or ( Z ) configured. However, the first step is partially reversible.
- In the case of reversible addition, the thermodynamically more stable addition product is formed in these cases (thermodynamic reaction control).
- In the case of irreversible addition, the product is formed which is formed more quickly (lower activation energy; kinetic reaction control).
Shortly after the discovery, it was recognized that the Wittig reaction is usually quite diastereoselective. Depending on the reactivity of the ylide / carbonyl components used, the diastereoselectivity can be specifically changed by choosing the substituents (both on the ylide and on the carbonyl compound) and the reaction conditions, the yield ratio of the isomers. Since the reactivity of the carbonyl is usually given, one tries to influence the diastereoselectivity by adjusting the reactivity of the ylide. The general rule:
Reactivity of the carbonyl compound | ||||
---|---|---|---|---|
high | medium | low | ||
Reactivity of the ylide |
||||
high | ( Z ) | ( Z ) | ( E , Z ) | |
medium | ( Z ) | ( E , Z ) | ( E , Z ) | |
low | ( E ) | ( E ) | ( E ) |
Possibilities of varying the reactivity of the oxaphosphetane
Destabilization can take place by replacing the substituents on phosphorus with π / σ donors. The ( Z ) -olefins are then usually formed in high yields .
Stabilization can be achieved by replacing the substituents on the phosphorus with π / σ acceptors. The ( E ) -olefins are then usually formed in high yields .
Multi-stage (less reactive components)
The reaction is initiated by the attack of the carbanion on the positively polarized carbonyl carbon atom and the formation of the betaine. The carbonyl compound is in most cases prochiral; H. When betaine is formed, a new chiral center is formed, which is (pro- R or pro- S ) ( R ) or ( S ) -configured depending on the attack side .
The carbanion of Ylides is also prochiral. So there is an intermediate formation of a compound that contains two adjacent chiral carbon atoms. Depending on how the substituents are arranged, there are two different forms that can be traced back to the carbohydrates threose and erythrose . For naming purposes, the substituents are sorted according to size. If a conformation results (through rotation around the CC axis) in which the large, medium and small substituents are opposite each other, one speaks of the erythro form, alternatively of the threo form. The passage through the betaine intermediate could be proven by isolating stable representatives.
The betaine reacts via the conformer, which enables a thermal [2 + 2] cycloaddition to form the cyclic oxaphosphetane. The existence of the four-ring intermediate could be demonstrated by 31 P-NMR spectroscopy. The oxaphosphetane formed at −80 ° C is stable at these temperatures. It decomposes when heated to 0 ° C.
A retro - [2 + 2] cycloaddition results in the formation of triphenylphosphine oxide and the ( E ) -alkene.
Concerted (more reactive components)
In the case of unstabilized ylides, the reaction is rapid. Due to Coulomb attraction, the reactants approach each other orthogonally (the positively polarized atoms attach to each negatively charged one). At the same time, the “large” methyl groups are positioned as far away from each other as possible for steric reasons. The bond is formed by torsion of the transition complex and concerted [2 s +2 a ] cycloaddition (disrotatory ring closure). The betaine intermediate stage is practically skipped.
The Z -oxaphosphetane is finally formed via a “twisted” four-ring .
Side reactions
Unstabilized ylides are unstable to oxygen and water. Hydrolysis turns an ylide into a phosphine oxide and a hydrocarbon.
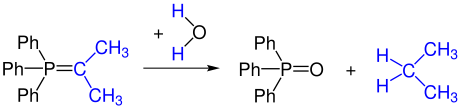
Part of the ylide can be reoxidized to form the carbonyl compound by partial reaction with oxygen (or by adding oxidizing agents). This carbonyl compound reacts with another ylide to form an alkene.

These side reactions are usually undesirable and reduce the yield, which is why one works under inert gas and with exclusion of moisture. However, some of these reactions are also used preparatively.
Enantioselective Wittig Reactions
By using chiral phosphorus ligands, Trost and Curran achieved an enantiomeric excess ( ee ) of 30–40% in an intramolecular Wittig reaction . Look there.
Wittig-like reactions
With other carbonyl and heterocarbonyl compounds
Phosphorus ylides also react in a similar way with other carbonyl compounds than aldehydes or ketones, for example with ketenes , with isocyanates , with various anhydrides and imines .

Arsenic ylids
Like this, the homologue of phosphorus forms quaternary arsonium salts, which can also be deprotonated with bases in the α position. These arsenic ylides behave like nitrogen ylides in carbonyl olefination.
Examples
The versatility of the Wittig reaction is shown in the following figure using a few examples:
Limits of the Wittig reaction
A disadvantage of the Wittig reaction is its restriction to aldehydes and ketones (see above for exceptions). Carboxylic acid derivatives are more or less inert to ylides. The Wittig reaction also requires basic conditions that can initiate side reactions such as eliminations or racemizations. Alternative olefination reagents are, for example, in titanocene compounds (see Tebbe reaction ).
Alternatives to the Wittig reaction
Peterson olefination , the Julia olefination, and the Tebbe reaction . Furthermore, the metathesis .
variants
- Horner-Wadsworth-Emmons reaction
- Schlosser's variant of the Wittig reaction
- Takai olefination
literature
- Thomas Laue, Andreas Plagens: Name and keyword reactions in organic chemistry. 5th edition. Vieweg + Teubner-Verlag, 2006, ISBN 3-8351-0091-2 .
- Marcel Hoffmann: Development of catalytic Wittig reactions. Berlin, Mensch - & - Buch-Verlag, 2014, ISBN 978-3-86387-476-6 .
Individual evidence
- ↑ G. Wittig, G. Geissler: To the reaction of pentaphenyl-phosphorus and some derivatives . In: Liebigs Ann. Chem. Band 580 , no. 1 , 1953, p. 44-57 , doi : 10.1002 / jlac.19535800107 .
- ↑ G. Wittig, U. Schöllkopf: About triphenyl-phosphine-methylene as olefin-forming reagents . In: Chemical Reports . tape 87 , no. 9 , 1954, pp. 1318-1330 , doi : 10.1002 / cber.19540870919 .
- ↑ G. Wittig, H. Pommer: DBP 954247 , 1956.
- ↑ G. Wittig, H. Pommer: In: Chem. Abstr. 53, 1959, p. 2279.
- ↑ a b Heinz G. Becker et al. (Ed.): Organikum . Organic-chemical basic internship . Wiley-VCH, Weinheim 2004, ISBN 3-527-31148-3 .
- ↑ L. Fitjer, U. Quabeck: In Chem. Commun. 15, 1985, pp. 855-864.
- ↑ D. Spitzner, K. Oesterreich: Anionically Induced Domino Reactions - Synthesis of a Norpatchoulenol-Type Terpene . In: European Journal of Organic Chemistry . tape 2001 , no. 10 , 2001, p. 1883-1886 , doi : 10.1002 / 1099-0690 (200105) 2001: 10 <1883 :: AID-EJOC1883> 3.0.CO; 2-M .
- ↑ M. Schlosser, B. Schaub: In Chimia 36, 1982, pp. 396-397.
- ↑ Jerry March: Advanced Organic Chemistry . McGraw-Hill, Kogakusha 1977, ISBN 0-07-040247-7 .
- ↑ KPC Vollhardt , NE Schore: Organic Chemistry. 4th edition. Wiley-VCH, Weinheim 2005, ISBN 3-527-31380-X , p. 891.
- ↑ Robert B. Woodward, Roald Hoffmann: The preservation of orbital symmetry . Verlag Chemie, Weinheim 1970, ISBN 3-527-25323-8 , pp. 1-178.
- ↑ Kaim, W .: One-electron transfer: Farewell to electron pair mechanisms? In: Nachr. Chem. Tech. Lab. 32, 1984, pp. 436-439.
- ↑ Peter Sykes: reaction mechanisms of organic chemistry. 7th edition. Verlag Chemie, 1979, ISBN 3-527-21047-4 .
- ↑ M. Schlosser, KF Christmann In: Liebigs Ann. Chem. 708, 1967, p. 1.
- ↑ Thomas Laue, Andreas Plagens: Name and catchword reactions of organic chemistry. 5th edition. Vieweg + Teubner-Verlag, 2006, ISBN 3-8351-0091-2 .
- ↑ BE Maryanoff, AB Reitz, MS Mother, RR Whittle, RA Olofson: Stereo Chemistry and mechanism of the Wittig reaction. Diasteromeric reaction intermediates and analysis of the reaction course . In: J. Am. Chem. Soc. tape 108 , no. 24 , 1986, pp. 7664-7678 , doi : 10.1021 / ja00284a034 .
- ↑ E. Vedejs include: Topics in Stereochemistry. Volume 21, 1994, ISBN 0-471-52120-5 .
- ^ A. Streitwieser, CH Heathcock: Organic Chemistry. Verlag-Chemie, 1980, ISBN 3-527-25810-8 .
- ^ Nguyên Trong Anh: The Woodward-Hoffmann rules and their application . Verlag Chemie, 1970, ISBN 3-527-25430-7 .
- ↑ H.-J. Bestmann, R. Armsen, H. Wagner, Chem. Ber. 102, 1969, pp. 2259-2269.
- ↑ BM Trost, DP Curran: An enantiodirected cyclopentenone annulation. Synthesis of a useful building block for condensed cyclopentanoid natural products . In: J. Am. Chem. Soc. tape 102 , no. 17 , 1980, pp. 5699-5700 , doi : 10.1021 / ja00537a059 .
- ↑ Asknes and Frøyen, Acta Chem. Scand. , 22, 1968, p. 2347.
- ↑ Frøyen: Acta Chem. Scand., Ser. B . 28, 1974, p. 568.
- ↑ Chopard, Hudson, Searle: Tetrahedron Lett. , 1965, p. 2357.
- ↑ Flitsch, Peters: Tetrahedron Lett. , 1969, p. 1161.
- ↑ Gara, Massy-Westropp and Reynolds: Tetrahedron Lett. , 1969, p. 4171.
- ↑ Bestmann, Seng: Tetrahedron . 21, 1965, p. 1373.
- ^ AF Holleman , E. Wiberg , N. Wiberg : Textbook of Inorganic Chemistry . 81–90. Edition. Walter de Gruyter, Berlin 1976, ISBN 3-11-005962-2 .