Heliocentric view of the world
The heliocentric worldview ( ancient Greek ἥλιος helios , German “sun” and κέντρον kentron center), also called the Copernican worldview , is a worldview in which the sun is considered the center of the universe at rest. The planets, including the earth, move around the center, while the fixed stars are said to be attached to a stationary outer spherical shell. The earth revolves around itself once a day and the moon moves around the earth once a month.
In its beginnings, the heliocentric worldview goes back to the Greek astronomers Aristarchos of Samos and Seleukos of Seleukia , but it was only worked out in detail in the 16th century by Nicolaus Copernicus and decisively improved in the 17th century by Johannes Kepler and then especially by Isaac Newton .
It thus prevailed against the geocentric worldview that had prevailed since antiquity , in which the earth is not a moving planet, but rests and represents the unmoving center around which the sun, moon, planets and fixed stars rotate. Although this corresponds to the direct perception of the movements of the stars, it makes the explanation of the small irregularities observed in ancient times extremely complicated and has also been refuted by direct measurements since the 18th century.
In the strict sense, the usual designation as a heliocentric system only applies to the level of development reached by Kepler, because with Copernicus (70 years earlier) the planets and even the sun itself revolved around an imaginary point a little outside the sun, called the “middle one Sun ”, and for Newton (60 years later) already around the barycenter of the solar system, which is mostly a little outside the sun . At the same time, the modern idea matured that the universe as a whole has no center at all.
Compared to the geocentric view of the world, the heliocentric view of the world was much simpler and for the first time paved the way for a much more precise description and prediction of the positions of the sun, stars and planets. Even when it was first created, it was in conflict with many religious ideas about the role of man and his place in the universe . For a long time, it seemed unacceptable that the earth was not in the center and that it was itself in motion. The heliocentric view of the world met with fierce opposition from the Christian churches (see e.g. Galileo Trial ). The emergence and spread of the heliocentric worldview are closely connected with the emergence of modern natural sciences and are therefore also referred to as the Copernican turn .
Forerunner of the heliocentric worldview
Ancient Greece

Little is known about what was thought in ancient Greece about a worldview that did not focus on the earth. For the Pythagorean school from the 6th century BC In BC fire was the most important element. For example, Philolaus (5th century BC) assumed that the sun, earth and the other celestial bodies orbit an underground and therefore invisible central fire . Aristotle (4th century BC) reports about it: “ In the center, they (the Pythagoreans) say , there is fire and the earth is one of the stars and creates night and day by moving in a circle around the center. “But he rejected this worldview, gave reasons for a geocentric worldview and thus remained decisive until the 17th century AD.
Aristarchus of Samos (3rd century BC), of whom a book with a geocentric view of the world has survived, is said to have also written a book with a heliocentric view of the world. The earth's orbital motion is said to have appeared for the first time as a natural explanation of the planet's temporarily retrograde motion. Aristarchus also knew that the stars would then have to show a parallax . However, this was not observed at the time, which he explained by assuming a very large distance between the stars. Aristarchus also estimated the size of the moon and the distance from the earth to the moon and the sun. The calculations for the moon were acceptable, but for the sun he misjudged himself by many orders of magnitude. At the same time, his contemporary Eratosthenes was able to calculate the circumference of the earth almost exactly.
Seleucus of Seleukia (2nd century BC) is said to have represented a heliocentric view of the world. When he was alive, the consequences of a heliocentric view of the world were probably already calculable, so that the view of the world could be checked ("proven") on the basis of the observations. More details are not known about Seleucus.
In the second century AD, Claudius Ptolemy developed a system that included an epicyclic theory on a geocentric basis to reconcile astronomical observations with the Aristotelian principle of uniform motion. This theory provides for such a complicated construction of up to 80 multi-stage circular motions of fictitious points in space for the movable celestial bodies that it hardly seemed compatible with the Aristotelian commandments and still left a lot to be desired in terms of accuracy. Nevertheless, it was used almost unchanged for centuries to calculate the movements of the sun, moon and planets.
India
The Indian astronomer and mathematician Aryabhata (476-550) assumed that the earth rotated on its own axis and discovered that the moon and planets reflect the light of the sun. It is assumed that he represented a heliocentric view of the world, because in his model for calculating the planetary positions he specified the orbital times around the sun for Venus and Mercury, not around the earth.
Islamic astronomy in the Middle Ages
The Islamic astronomers stuck to the geocentric view of the world in the Middle Ages, but noticed the lack of agreement with the observations. As a problematic weak point they recognized the introduction of the equant , a fictitious point away from the world center, with the help of which Ptolemy's non-uniform movements appear uniform to the earthly observer in the epicyclical theory ; this hypothesis also contradicted the principle of uniform circular motion.
The Persian scientist Nasir ad-Din at-Tusi (1201–1274) solved this and other problems of the Ptolemaic system with the help of the Tusi pairs , which are two circular movements with one circle rolling on the inside of the other. At-Tusi showed that linear movements can also arise from this, which at the same time refuted the Aristotelian doctrine of the unbridgeable difference between linear and circular movements.
The scientist Mu'ayyad ad-Din al-Urdi (approx. 1250) developed the Urdi lemma , with the help of which a circular motion through an epicyclic can be turned into an eccentric circular motion. Urdi lemma and Tusi pair were later used in the Copernican model, but without any reference to their discoverer.
Ibn al-Shatir (1304-1375) resolved in his treatise Kitab Nihayat as-Sulfi Tashih al-Usul the need for an equant by introducing an additional epicyclic into the Ptolemaic system. In the same way, Copernicus later succeeded in constructing his model without quanta. Otherwise Ibn al-Shatir stayed with the geocentric system.
Establishment of the heliocentric worldview
The inadequacies of the Ptolemaic system were also increasingly recognized in Europe. Georg von Peuerbach and Regiomontanus expressed cautious doubts about its correctness in the 15th century and found some improvements.
The breakthrough to the heliocentric worldview in its present form took place in a multitude of steps. The three most important of them each represent a paradigm shift in that they make a thought that was previously excluded or not even considered as a new starting point:
- At the beginning of the 16th century, Nicolaus Copernicus gave up the idea of the earth as the center of the universe. He attributed a movement to it and the other planets in the form of orbits around the sun. He determined the sun as the common center of movement of all planetary orbits.
- At the beginning of the 17th century, Johannes Kepler disregarded the doctrine of the uniform movement of the heavenly bodies on combinations of predetermined circular paths. He assumed an elliptical orbit in each case , in which the sun is in a focal point and the orbital speed is constantly changed by the direct influence of the sun.
- At the end of the 17th century, Isaac Newton put an end to the separation between heavenly and earthly mechanics, thus establishing today's classical mechanics .
New paradigm through Nicolaus Copernicus
In search of simplification or improvement of the Ptolemaic system, Copernicus came across the heliocentric model, which he knew from ancient Greek sources. He first sketched it in his Commentariolus , written around 1510 , which remained unprinted, but probably became known to astronomers through copies. In his main work De revolutionibus orbium coelestium (English: About the revolutions of the heavenly circles ), which appeared in print in 1543 , he then elaborated on it in detail. Copernicus was the first to work out the essential advantage of the heliocentric over the geocentric system: The heliocentric system explains the variable speed of the planets and in particular their sometimes even retrograde motion simply by the fact that this only appears from the earth, because it is itself around the sun run.
In the way of posing the problems and the method of solving them, Copernicus remained in the traditional style of Greek astronomy. Like Aristotle, he advocated the proposition that there can only be the most perfect motion in the sky, the uniform circular motion. In order to achieve an approximate agreement with the observational data, he had to assume, just like Ptolemy, that the movements of the planets occur on epicycles ; H. on circles whose centers move on other circles. Already the center of the earth's orbit - the "middle sun", which with Copernicus also became the center of all other planetary orbits - the true sun should revolve on two compound circular movements. Contrary to popular representations, Copernicus required no fewer circular movements than Ptolemy while maintaining the same level of accuracy. In addition to the conceptual simplification of the system, he himself saw his greatest success in having fulfilled the Aristotelian theorem better than Ptolemy, in which he had made the equant superfluous. Both systems resulted in position errors of up to 10 arc minutes (1/3 moon diameter). This was not insignificant in those days, which were still very much influenced by astrology , because it can mean an error of a few days in the prediction of the time of certain astronomical events. So z. B. When two planets meet or a planet with a fixed star (p. 58) .
Although Copernicus gave his world model no physical foundation, he opposed the ancient philosophy of nature represented up to that point in three essential points .
- While Aristotle saw the circular motion of the heavenly bodies as a natural, separate motion of a heavenly matter ( ether or quinta essentia ), according to Copernicus the circular motion was the direct consequence of the spherical shape of the celestial bodies, so that he neither postulated a special kind of heavenly matter as a reason - like Aristotle nor - as in pre-Aristotelian times - had to draw on a divine cause.
- While in the Aristotelian-Ptolemaic way of thinking bodies fall on earth because they strive for the world center, which is thought to be in the earth center, in Copernicus bodies fall on earth in order to reunite with their matter; thus it becomes a matter of indifference whether the earth is at the center of the world or not.
- While Ptolemy rejected a daily rotation of the earth with the argument that a stone thrown vertically up must land further west and that birds and clouds would have to drift to the west because the earth rotates under them, Copernicus assumed that the atmosphere and that contained in it would rotate with it Objects out.
With the last two points, Copernicus opened a way towards the later Newtonian concepts of gravity and inertia.
The first edition of De revolutionibus orbium coelestium was accompanied by an introduction by Andreas Osiander , in which Copernicus' approach was presented as a purely mathematical hypothesis that does not correspond to reality. At that time, “hypothesis” was understood to be a mere calculation method. There was as yet no natural science with the current claim to explanation. The view of the world was essentially interpreted and justified philosophically and theologically, and there were also recipe-like instructions for the practical calculation of the positions of the stars and planets, in which it was mainly a matter of the accuracy achieved and less of whether they correspond to the philosophically and theologically justified views fit.
The first observations that directly contradicted the geocentric view of the world were made by Galileo Galilei in 1609/1610. With his (still very simple) telescope, he discovered the moons of Jupiter , i.e. stars that do not orbit the earth, and the phases of Venus , which run differently than would have been compatible with an orbit around the earth.
The Copernican system was a significant simplification of the Ptolemaic system, but could not improve its inadequate accuracy noticeably. But due to his paradigm shift , no longer seeing the earth as the center of the world, Copernicus is considered to be the trigger of the Copernican turn and an important pioneer of the transition from medieval to modern thinking.
Mathematical specification by Johannes Kepler
Copernicus had adapted his heliocentric model almost exclusively to the same old observational material that Ptolemy had used for his geocentric model, because he wanted to prove the equivalence of his system, and there was no other material to speak of. In order to be able to make a decision between the two models, more precise measurements were required. Towards the end of the 16th century, Tycho Brahe was able to determine the position of the planet and almost 1000 stars over two decades, with an accuracy of 1 to 2 arc minutes that for the first time exceeded that of the old data.
Johannes Kepler , to whom Tycho had given his data, discovered in years of unsuccessful efforts that they could not be explained with either of the two systems. The maximum deviation of the calculated position of Mars from the observed orbit could not be reduced to less than eight arc minutes. Kepler examined the changing orbital velocity of the planets more closely and found that it cannot be represented in the epicyclic model with uniform circular motions. He found out that the speed depends on the current distance of the planet from the (true) sun instead of the distance to the central sun. He therefore saw the sun as the physically effective center of the planetary system and reinterpreted all observation data in relation to the true instead of the mean sun. In particular, he was looking for a mathematically accurate description of the motion of Mars and eventually realized the need to improve the calculated values through a better modeled Earth's orbit . Therefore, he first had to grasp this more precisely. He succeeded in doing this with the help of selected observation data in which Mars was at the same point in its orbit, but the Earth was at different. The effect is the same as if he had held Mars and measured the movement of the earth from there. The idea for this procedure could only arise on the basis of the Copernican model. On the basis of the Earth's orbit, which is now more precisely known, he reevaluated the Mars observations and found that an elliptical orbit fits by far best ( Astronomia Nova , 1609, with the 1st and 2nd of Kepler's three laws ). He checked this on the other planets, including the earth itself, and the moon (where the earth is at the focal point of the ellipse). He discovered the connection between the size of the orbit and the period of revolution of the celestial body as the 3rd Kepler's law ( Harmonices mundi libri V , 1619). With this, Kepler was able to summarize the comprehensive description of the solar system in three Kepler's laws of planetary motion, with which, compared to Copernicus and Ptolemy, the accuracy for calculating the planetary positions was about ten times better.
Kepler owed the breakthrough to his important new guiding principle: Since the planets do not, due to their heavenly nature, follow their predetermined orbits unaffected and with uniform speed, a constantly effective cause is required for the deviations, not in a mere mathematical point, but only in the true one Sun can lie. In Kepler's heliocentric system, the sun is no longer just the most central body in the planetary system, but also the one that is the only one that has an effect on all others. Although Kepler had wrong ideas about this "force" and its mode of action, he added a decisive element to the heliocentric worldview and prepared the development of the later celestial mechanics.
Physical justification by Isaac Newton
In his major work Philosophiae Naturalis Principia Mathematica , published in 1687, Isaac Newton found a formulation of mechanics that became the basis of today's classical mechanics . Guided by Kepler's three laws and the idea of being able to apply laws of earthly mechanics to events in the cosmos, he discovered the general law of gravitation and was able to derive Kepler's laws from it. This was the first time that an astronomical view of the world was placed on a solid physical basis.
In the context of Newtonian physics, however, it is not the sun itself that is in a common focal point of all the elliptical orbits of the planets, but the center of gravity of the entire solar system. The sun also performs its own orbital movement around this barycentre , with a varying distance of up to two solar radii (measured from the sun's center).
In addition, Newton recognized that Kepler's ellipses are only approximations to the actual planetary orbits. They are only correct if you neglect the forces of attraction between the planets. These cause small deviations, which are called path disruptions . In the course of the 18th century, the mathematical methods were developed with which the orbital disturbances could be calculated. This increased the accuracy of the predictions again by about fifty times.
With more precise astronomical instruments than in Galileo's time, the correctness of the heliocentric system, in particular the annual and daily movement of the earth, could be verified by measurements. The orbital motion of the earth was demonstrated in 1725 by James Bradley through the discovery of aberration and in 1838 by Friedrich Wilhelm Bessel through the discovery of the annual parallax of the stars. Trap experiments to prove the earth's rotation were carried out from 1800 onwards. The direct proof of the earth's rotation came about in 1851 with the help of Foucault's pendulum .
reception
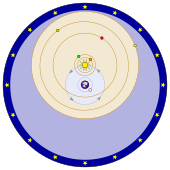
Already with Aristarchus in the 3rd century BC The heliocentric view of the world was classified as "anti-religious". This was also true in Christian Europe, which is why this view of the world remained insignificant until the 16th century AD.
After the 11th century, Arabic texts were increasingly translated into Latin in the Toledo translation school. The Franciscan Bonaventure of Bagnoregio gave a lecture on a heliocentric view of the world contained therein in his Collationes in Hexaemeron 1273 and interpreted it theologically as Christ as the center of creation. He was followed in the discussion by Nikolaus von Oresme and Nicolaus Cusanus . Most scholars, however, saw in the world view with an earth rotating or even orbiting the sun, the problem that people and objects should fall obliquely or even fly out into space; an object falling from the tower should not hit the ground exactly vertically due to the eastward rotation of the earth, but should be deflected to the west. The Bible also seemed to contradict the heliocentric worldview by reporting that in the battle of the Israelites against the Amorites, God once commanded the moon and the sun to stand still Jos 10: 12-13 LUT , not the earth.
Against the heliocentric view of the world, the Catholic monastic order of the Dominicans demanded a teaching ban, which was not initially implemented. Protestants also expressed themselves decisively against the Copernican view of the world in the 16th century. It is often shown that Martin Luther himself spoke out against it in a table conversation (1539) with clear words: "This fool would like to twist the entire art of astronomy. " However, this is probably a subsequent tightening, because the most original source for this quote only says: "As does one who wants to turn all of astronomy around". Furthermore, not a single statement on the heliocentric worldview is known from Luther.
As a compromise, Tycho developed a system in which the earth stands still and is orbited by the sun and moon, while the other planets - as in the Copernican system - orbit the sun. The Jesuit astronomers in Rome were initially skeptical of this system, such as Christophorus Clavius , who commented that Tycho Brahe "confused all astronomy because he wanted Mars closer than the sun." Ideas went ahead, the Jesuits switched to Brahe's system. From 1633 the use of this system was mandatory.
Tycho also reinforced doubts about the prevailing view of the world, because he could not determine a measurable parallax either in the supernova of 1572 or on the comet of 1577 and concluded that both must be far outside the lunar orbit. According to the doctrine of the time, which was shaped by Aristotle, there should be heavenly perfection, so that there should be no processes of arising and fading.
At this time, the physical conceptions of Aristotle and thus the worldview represented by the Church were questioned or even refuted by the first results of the incipient natural science in today's sense. Particularly noteworthy is Galileo Galilei with his experiments on free fall and crooked throw and his discoveries of the phases of Venus and the moons of Jupiter . The Catholic Church began to strictly defend the geocentric worldview. Pope Urban VIII had approved the publication of Galileo's work Dialogue Regarding the Two Main World Systems (i.e. the geocentric and the heliocentric view of the world), but now opposed it.
In a well-received inquisition trial , Galileo was accused of "... a false doctrine taught by many, namely that the sun is immovable in the center of the world and that the earth moves". The final verdict was that he was guilty of "heresy". With the fall of Galileo, the conflict between the church's claim to authority and free science was raised into social consciousness for the first time beyond the church.
Triggered by the general recognition that Newton found with his results in the scientific world, Pope Benedict XIV lifted the ban on April 17, 1757 against works that represented the heliocentric worldview. On September 11, 1822, the Congregation of the Roman and General Inquisition ruled that the printing and publication of works depicting the movement of the planets and the sun in accordance with the conception of modern astronomers was permitted. In 2018, a Foucault pendulum was hung in the former Catholic Dominican church of the city of Münster , in order to make "the earth's rotation, which was first demonstrated in a pendulum test in 1851 by the French physicist Léon Foucault, visible".
After the idea of an unlimited universe was already expressed in antiquity ( Leukippus , Demokrit , Lucretius ), Nikolaus von Kues showed in the 15th century that the earth cannot be a center in an infinite universe, just as little as any other celestial body, with which he excluded both a geocentric and a heliocentric worldview. These views were later also represented by Thomas Digges and Giordano Bruno and prevailed as a doctrine in the course of the 18th and 19th centuries, even before galaxies outside the Milky Way were discovered in the 20th century . The heliocentric worldview was gradually replaced by the cosmological principle from around 1930 .
The cosmological principle says that in principle there is no place that is distinguished from another, thus also no center. Worldviews that emphasize a certain place in the universe are considered outdated. Together with the isotropy of the universe, i.e. that no direction is marked, the cosmological principle forms the cornerstone of the standard model of cosmology , which is supported by observation of the observable universe .
However, the cosmological principle is only applicable to large scales spanning millions of light years. In smaller systems such as B. a galaxy (typically a few 100,000 light years in size) or our solar system (less than 1/1000 light years in size), excellent points can be specified. Thus, although the sun is not the center of the universe, it forms the center of the solar system, since its center of gravity is never further than about a solar radius from the solar surface and is often even within it.
Likewise, from a physical point of view, according to the general theory of relativity, every free falling system has equal rights and a change from the geocentric to the heliocentric worldview is only a coordinate transformation. Since the sun comes closest to the center of gravity of the solar system, the heliocentric reference system is almost identical to the center of gravity system and therefore often serves as a simple reference system for the representation of processes in the solar system.
literature
- Eduard Jan Dijksterhuis : The mechanization of the worldview. Springer, Berlin / Heidelberg / New York 1956. (Reprint 1983 (with a foreword by Heinz Maier-Leibnitz ), ISBN 3-540-02003-9 )
- Jürgen Hamel : History of Astronomy in Source Texts. Spektrum Akademischer Verlag, Heidelberg 1996, ISBN 3-8274-0072-4 .
- Dieter B. Herrmann : discoverer of the sky. Pahl-Rugenstein Verlag, Cologne 1979, ISBN 3-7609-0454-8 .
- Jürgen Teichmann : Change of the worldview (= cultural history of the natural sciences and technology ). 2nd edition, Rowohlt, Reinbek bei Hamburg 1985, ISBN 3-499-17721-8 .
- Ernst Zinner : Origin and Spread of the Copernican Teaching. 2nd edition, reviewed and supplemented by Heribert M. Nobis and Felix Schmeidler . CH Beck, Munich 1988, ISBN 3-406-32049-X .
Web links
- Alan H. Batten: Article on the development of the heliocentric worldview from Aristarchus to Kepler
- The Kepler Ellipse - Martin Holder (University of Siegen) - a detailed report on Kepler's approach to finding the planetary laws
Individual evidence
- ^ Eduard Jan Dijksterhuis : The mechanization of the world view . Springer, Berlin / Heidelberg / New York 1956, ISBN 3-540-02003-9 .
- ↑ Aristotle: De Caelo , Book 2, Chapter 13
- ↑ Jeffrey O. Bennett, Harald Lesch : Astronomy: the cosmic perspective . Addison-Wesley in Pearson Education Germany, 2010, ISBN 978-3-8273-7360-1 , pp. 68 .
- ↑ a b Bartel Leendert van der Waerden : The Heliocentric System in Greek, Hindu and Persian Astronomy. In: Annals of the New York Academy of Sciences . tape 500 , 1987, pp. 525-545 .
- ^ Hugh Thurston: Early Astronomy . Springer-Verlag, New York 1993, ISBN 0-387-94107-X .
- ↑ Dijksterhuis 1988, pp. 67, 73.
- ↑ Herrmann 1979, p. 54.
- ↑ Jürgen Hamel: History of Astronomy. 2nd Edition. Kosmos-Verlag, Stuttgart 2002, ISBN 3-440-09168-6 , pp. 123, 128.
- ↑ Dijksterhuis 1988, p. 320.
- ↑ Arthur Koestler : Die Nachtwandler - The history of our knowledge of the world . 3rd Edition. Suhrkamp Taschenbuch, Volume 579, Frankfurt am Main 1988, ISBN 3-518-37079-0 , pp. 190 ff . The exact number of circular motions is given by Copernicus once as 34 (in his announcement of the new model in Commentariolus of approx. 1510), but according to Koestler's count, it is 48 in the main work.On the other hand, the Ptolemaic system did not need 80 epicycles, as Copernicus claims, but in the version last updated by Peurbach in 1543, only 40.
- ↑ Jürgen Hamel: History of Astronomy in Source Texts . Spektrum Akad. Verlag, Heidelberg 1996, ISBN 3-8274-0072-4 , p. 30th ff .
- ↑ Dijksterhuis 1988, p. 321.
- ^ Robert Wilson : Astronomy through the Ages . Taylor and Francis, London 1997, ISBN 0-7484-0748-0 .
- ↑ Dijksterhuis 1983, pp. 36, 322.
- ↑ Dijksterhuis 1983, p. 323.
- ↑ Dijksterhuis 1983, pp. 72, 322.
- ^ Eduard Jan Dijksterhuis: The mechanization of the world view . Springer, Heidelberg 1966, p. 330 ff .
- ^ Hugh Thurston: Early Astronomy . Springer Verlag, New York [u. a.] 1994, ISBN 0-387-94107-X , pp. 220 ff .
- ↑ Bruce Stephenson: Kepler's Physical Astronomy . Springer, New York 1987, ISBN 978-1-4613-8739-8 , doi : 10.1007 / 978-1-4613-8737-4 .
- ↑ Martin Holder: The Kepler Ellipse . Universitätsverlag Siegen, Siegen 2015, ISBN 978-3-936533-64-4 ( online [accessed December 18, 2017]).
- ^ Jean Meeus : Mathematical astronomy morsels . Richmond, Va. 2009, ISBN 978-0-943396-92-7 , pp. 165 .
- ^ Nicolaus Copernicus Edition , Volume VI, 2: Documenta Copernicana. Documents, files and messages. Texts and translations. Edited by Andreas Kühne and Stefan Kirschner. Akademie Verlag, Berlin 1996, ISBN 3-05-003009-7 , p. 372.
- ↑ Andreas Kleinert: A tangible historical lie. How Martin Luther was made an opponent of the Copernican world system. In: Reports on the history of science. 26/2003, pp. 101-111.
- ^ Münster town hall: Dominican church opened with Gerhard Richter artwork
- ↑ Herrmann 1979, pp. 36, 55.