Spent nuclear fuel
Spent or spent nuclear fuel (Spent Nuclear Fuel, SNF) is nuclear fuel from a nuclear reactor (usually a nuclear power plant ). It is no longer useful for maintaining the chain reaction (neutron-induced nuclear fission) in an ordinary thermal reactor and, depending on the point in the nuclear fuel cycle , it can contain significantly different isotopic constituents.
Type of fuel used
See: John H Large: Radioactive Decay Characteristics of Irradiated Nuclear Fuels, January 2006 .
Nanomaterial properties
There are intense temperature gradients in the oxide fuel , which cause fission products to migrate. The zirconium tends to move to the center of the fuel, pellets where the temperature is highest, while the lower boiling fission products move to the edge of the pellet. The pellet likely contains many small bubble- like pores that formed during use; the fission product xenon migrates into these gaps. Some of this xenon then breaks down to cesium . Hence, many of these bubbles contain a large concentration of 137 Cs.
In MOX fuel assemblies , when xenon escapes from the fuel, it diffuses out of the plutonium-rich areas of the fuel and is then trapped in the surrounding uranium dioxide, neodymium tends not to be mobile.
Metallic particles of an alloy of Mo-Tc-Ru-Pd also tend to form in the fuel. Other solids form at the boundary between the uranium dioxide grains, but the majority of the fission products remain dissolved in the uranium dioxide as mixed crystals . There is a paper that describes a method for separating non- radioactive uranium from active uranium from spent fuel elements.
Fission products
3% of the mass consists of fission products of 235 U and 239 Pu (also indirect products in the decay chain ); these are taken into account in radioactive waste or can be further separated for various industrial and medical purposes. The fission products contain every element from zinc to lanthanoids ; A large part of the fission yield is concentrated on two peaks, one in the second transition row ( zirconium , molybdenum , technetium , ruthenium , rhodium , palladium , silver ) and the other at the back of the periodic table ( iodine , xenon , cesium , barium , lanthanum , cerium , Neodymium). Many of the fission products are either non-radioactive or only short-lived radionuclides . A significant number, however, are medium to long-lived radioisotopes such as 90 Sr, 137 Cs, 99 Tc and 129 I. Several countries have conducted studies to separate the rare isotopes in fission waste, including the "fission platinoids" (Ru, Rh, Pd) and silver (Ag) to offset remanufacturing costs. This is currently not operated commercially.
The fission products can change the thermal properties of the uranium dioxide; Lanthanoid oxides tend to lower the thermal conductivity of the nuclear fuel, while the metallic nanoparticles increase the thermal conductivity of the nuclear fuel slightly.
Chemical data table
element | gas | Metal | oxide | Solid solution |
---|---|---|---|---|
Br Kr | Yes | - | - | - |
Rb | Yes | - | Yes | - |
Sr | - | - | Yes | Yes |
Y | - | - | - | Yes |
Zr | - | - | Yes | Yes |
Nb | - | - | Yes | - |
Mon | - | Yes | Yes | - |
Tc Ru Rh Pd Ag Cd In Sb | - | Yes | - | - |
Te | Yes | Yes | Yes | Yes |
I Xe | Yes | - | - | - |
Cs | Yes | - | Yes | - |
Ba | - | - | Yes | Yes |
La Ce Pr Nd Pm Sm Eu | - | - | - | Yes |
plutonium
About 1% of the mass is 239 Pu and 240 Pu , resulting from the conversion of 238 U, which can be considered either a useful by-product or a hazardous and undesirable waste. One of the main concerns is to prevent the proliferation of nuclear weapons , as this plutonium must not come into the possession of states that fall under the Nuclear Non-Proliferation Treaty . If the reactor was operated normally, only non-weapons grade plutonium is produced, it contains more than 19% 240 Pu and less than 80% 239 Pu, which makes it not ideal for making atomic bombs . If the irradiation time was kept short, the plutonium reached weapon quality by more than 93%.
uranium
96% of the mass is the remaining uranium: most of the original 238 U and a small amount of 235 U. As a rule, the 235 U is less than 0.83% of the mass together with 0.4% 236 U.
Recycled uranium will contain 236 U, which does not occur naturally; this is an isotope that is used as a fingerprint for recycled reactor fuel.
If thorium is used to make fissile 233 U, the 233 U in the spent nuclear fuel will have a half-life of 159,200 years (unless this uranium is removed from the spent fuel by a chemical process). The presence of 233 U has a long-term effect on the radioactive decay of the spent fuel. Compared to MOX fuel elements , the activity in the cycles with thorium is one million years higher because the not completely decayed 233 U is present.
About the natural uranium fuel:
The fossil component starts at 0.71% 235 U concentration in natural uranium. On discharge, the total fissile component is still 0.50% (0.23%). 235 U 0.27% fissile 239 Pu, 241 Pu Nuclear fuel is not given off because the fissile material has been completely consumed, but because neutron-absorbing fission products have accumulated and the fuel will be significantly less able to maintain a nuclear chain reaction.
For some naturally occurring uranium fuels, chemically active casings such as Magnox are used and must be processed because long-term storage and disposal are difficult.
Minor actinides
Small traces of actinides ( transuranic waste ) are present in the spent reactor fuel. These are actinides other than uranium and plutonium and include neptunium , americium, and copernicium . The amount formed depends largely on the type of nuclear fuel used and the conditions under which it was used. For example, using MOX elements ( 239 Pu in a 238 U matrix) will likely result in the production of more 241 U. Am and heavier nuclides as fuel based on uranium / thorium ( 233 U in a 232 Th matrix).
For highly enriched nuclear fuels in marine and research reactors , the isotope inventory varies based on the nuclear fuel management and the operating conditions of the reactor.
Spent fuel breaks down into heat
When a nuclear reactor has been shut down and the fission chain reaction has stopped, a significant amount of heat is still generated in the nuclear fuel due to the beta decay of fission products . For this reason, if the reactor had a long and constant power history, the heat of decay at the time the reactor was shut down is about 7% of the previous core power. About 1 hour after the shutdown, the heat dissipation is about 1.5% of the previous core power. After one day the decay heat drops to 0.4% and after a week to 0.2%. The decrease in the rate of heat generation slowly decreases over time.
Spent nuclear fuel withdrawn from a reactor is typically stored in a water-filled cooling pool container for a year or more (10 to 20 years in some locations) to cool it down and shield it from radioactivity. Practical cooling pools are generally not based on passive cooling, but rather require the water to be actively pumped through heat exchangers.
Nuclear fuel composition and long-term radioactivity
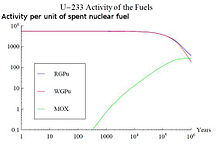
The use of different nuclear fuels in nuclear reactors leads to different SNF compositions with different activity curves.
Long-lived radioactive waste from the rear part of the fuel cycle is particularly relevant for drawing up a complete waste management plan for the SNSF. In the long run, radioactive decay has a significant impact on actinides in the SNSF due to their characteristically long half-lives. The actinide composition in the SNSF varies depending on how a nuclear reactor is fired.
An example of this effect is the use of nuclear fuel with thorium . 232 Th is a fertile material which has a neutron capture and two beta-minus decay can form, leading to the formation of fissile material 233 U . Its radioactive decay will greatly influence the long-term effects and activity in the SNSF by a million years. A comparison of the activities associated with 233 U for three different types of SNSF can be seen in the figure above on the right. The combusted fuels are thorium with reactor plutonium (RGPu), thorium with weapons plutonium (WGPu) and mixed oxide nuclear fuel (currently no thorium). For RGPu and WGPu, the initial amount of 233 U and its decay around a million years can be seen. This affects the overall activity curve of the three nuclear fuel types. The initial absence of 233 U and its daughter products in MOX elements leads to a lower activity in the right region of the figure below right, whereas for RGPu and WGPu the curve is kept higher due to the presence of 233 U has not completely decayed. Nuclear reprocessing can remove the actinides from spent fuel so that they can be used as a long-lived fission product or disposed of.
Corrosion of spent fuel elements
Precious metal nanoparticles and hydrogen
According to the work on corrosion by electrochemist David W. Shoesmith, nanoparticles of Mo-Tc-Pd have a strong influence on the corrosion of uranium dioxide fuel. For example, his work suggests that high hydrogen concentration (due to the anaerobic corrosion of the steel waste container), the oxidation of hydrogen on the nanoparticles will have a protective effect on the uranium dioxide. This effect can be seen as an example of the protection provided by a sacrificial anode , which reacts instead of a metal anode and dissolves the used hydrogen gas.
Storage, treatment and disposal
Spent nuclear fuel is stored either in the spent fuel pool (SFP) or in dry barrels . In the United States, spent fuel and drums reside either directly on nuclear power plant sites or in Independent Spent Fuel Storage Installations (ISFSI). ISFSIs can be adjacent to a nuclear power plant location or in a different location. The vast majority of ISFSIs store spent fuel in dry barrels. Morris Operation is currently the only ISFSI with a spent fuel pool in the United States.
Nuclear reprocessing can separate spent fuel in various combinations from reprocessed uranium, plutonium , minor actinides , fission products , leftover zirconium or steel cladding , activation products , and the reagents or solidifiers that are added during the reprocessing itself. If these components of the spent nuclear fuel were reused and there were limited additional waste as by-products of reprocessing, reprocessing could ultimately reduce the volume of waste to be disposed of.
Alternatively, the intact-spent nuclear fuel can be disposed of directly at the high level radioactive waste . The United States has planned a repository in deep geological formations , such as the Yucca Mountain repository for nuclear waste. There it must be shielded and packaged so that it does not end up in the immediate human environment for millennia. However, on March 5, 2009, Energy Secretary Steven Chu told a Senate hearing that "Yucca-Mountain is no longer viewed as an option for reactor waste storage.
Geological disposal has been approved in Finland using the KBS-3 process.
Risks
It is controversial whether spent fuel elements in the decay basin are susceptible to events such as earthquakes or terrorist attacks. This could likely lead to a release of radiation.
In the rare event of a nuclear fuel leak during normal operation, the primary coolant can penetrate the element. Optical techniques are typically used for post-irradiation testing of fuel assemblies.
Since the terrorist attacks of September 11, 2001 , the Nuclear Regulatory Commission has enacted a series of regulations that stipulate that all cooling tanks and reactors must be designed to be insensitive to natural disasters and terrorist attacks. As a result, used nuclear fuel pools are encased in a steel liner and thick concrete and regularly checked to ensure resistance to earthquakes, tornadoes, hurricanes, earthquakes and seichen or tsunamis .
Individual evidence
- ↑ PG Lucuta, RA Verrall, Hj. Matzke, BJ Palmer: Microstructural features of SIMFUEL - Simulated high-burnup UO 2 -based nuclear fuel . In: Journal of Nuclear Materials . 178, No. 1, January 1991, pp. 48-60. doi : 10.1016 / 0022-3115 (91) 90455-G .
- ^ Solution of Fission Products in UO 2 . Archived from the original on September 10, 2008. Retrieved May 18, 2008.
- ↑ RWMAC's Advice to Ministers on the Radioactive Waste Implications of Reprocessing . Radioactive Waste Management Advisory Committee (RWMAC). November 3, 2002. Archived from the original on August 29, 2008. Retrieved on May 18, 2008.
- ↑ David W. Shoesmith . University of Western Ontario . Retrieved May 18, 2008.
- ^ Electrochemistry and corrosion studies at Western . Shoesmith research group, University of Western Ontario. Retrieved May 18, 2008.
- ^ [1] Testimony of Robert Meyers Principal deputy Assistant Administrator for the Office of Air and Radiation US Environmental Protection Agency before the subcommittee on Energy and Air Quality Committee on Energy and Commerce US House of Representatives, July 15, 2008
- ^ H. Josef Hebert: Nuclear waste won't be going to Nevada's Yucca Mountain, Obama official says . In: Chicago Tribune . Archived from the original on March 24, 2011.
- ↑ Vincent Ialenti: Death and succession among Finland's nuclear waste experts . In: Physics Today . 70, No. 10, October 2017, pp. 48-53. bibcode : 2017PhT .... 70j..48I . doi : 10.1063 / PT.3.3728 .
- ↑ Fukushima's Spent Fuel Rods Pose Grave Danger . In: The Nation , March 15, 2011.
- ↑ Are Nuclear Spent Fuel Pools Secure? . Council on Foreign Relations. June 7, 2003. Archived from the original on April 12, 2011. Retrieved April 5, 2011.
- ↑ Mark Benjamin: How Safe Is Nuclear-Fuel Storage in the US? . In: Time Magazine , March 23, 2011.
- ^ WH Huang, TW Krause, BJ Lewis: Laboratory Tests of an Ultrasonic Inspection Technique to Identify Defective CANDU Fuel Elements . In: Nuclear Technology . 176, No. 3, April 10, 2017, pp. 452-461. doi : 10.13182 / NT11-A13320 .
- ↑ Fact Sheet on Storage of Spent Nuclear Fuel . Archived from the original on October 27, 2014. Retrieved June 25, 2017.
- ^ Nuclear Waste Disposal . Archived from the original on July 6, 2012. Retrieved June 5, 2012.