uranium
properties | |||||||||||||||||||||||||||||||||||||||||||||||||||||||||||||||||||||||||||||||||||||||||||||||||||||||
---|---|---|---|---|---|---|---|---|---|---|---|---|---|---|---|---|---|---|---|---|---|---|---|---|---|---|---|---|---|---|---|---|---|---|---|---|---|---|---|---|---|---|---|---|---|---|---|---|---|---|---|---|---|---|---|---|---|---|---|---|---|---|---|---|---|---|---|---|---|---|---|---|---|---|---|---|---|---|---|---|---|---|---|---|---|---|---|---|---|---|---|---|---|---|---|---|---|---|---|---|---|---|---|
General | |||||||||||||||||||||||||||||||||||||||||||||||||||||||||||||||||||||||||||||||||||||||||||||||||||||||
Name , symbol , atomic number | Uranium, U, 92 | ||||||||||||||||||||||||||||||||||||||||||||||||||||||||||||||||||||||||||||||||||||||||||||||||||||||
Element category | Actinoids | ||||||||||||||||||||||||||||||||||||||||||||||||||||||||||||||||||||||||||||||||||||||||||||||||||||||
Group , period , block | Ac , 7 , f | ||||||||||||||||||||||||||||||||||||||||||||||||||||||||||||||||||||||||||||||||||||||||||||||||||||||
Appearance | Silver white | ||||||||||||||||||||||||||||||||||||||||||||||||||||||||||||||||||||||||||||||||||||||||||||||||||||||
CAS number | 7440-61-1 | ||||||||||||||||||||||||||||||||||||||||||||||||||||||||||||||||||||||||||||||||||||||||||||||||||||||
EC number | 231-170-6 | ||||||||||||||||||||||||||||||||||||||||||||||||||||||||||||||||||||||||||||||||||||||||||||||||||||||
ECHA InfoCard | 100.028.336 | ||||||||||||||||||||||||||||||||||||||||||||||||||||||||||||||||||||||||||||||||||||||||||||||||||||||
Mass fraction of the earth's envelope | 3.2 ppm | ||||||||||||||||||||||||||||||||||||||||||||||||||||||||||||||||||||||||||||||||||||||||||||||||||||||
Atomic | |||||||||||||||||||||||||||||||||||||||||||||||||||||||||||||||||||||||||||||||||||||||||||||||||||||||
Atomic mass | 238.02891 (3) et al | ||||||||||||||||||||||||||||||||||||||||||||||||||||||||||||||||||||||||||||||||||||||||||||||||||||||
Atomic radius | 138.5 (α-uranium) pm | ||||||||||||||||||||||||||||||||||||||||||||||||||||||||||||||||||||||||||||||||||||||||||||||||||||||
Covalent radius | 142 pm | ||||||||||||||||||||||||||||||||||||||||||||||||||||||||||||||||||||||||||||||||||||||||||||||||||||||
Electron configuration | [ Rn ] 5 f 3 6 d 1 7 s 2 | ||||||||||||||||||||||||||||||||||||||||||||||||||||||||||||||||||||||||||||||||||||||||||||||||||||||
1. Ionization energy | 6th.19405 (6) eV ≈ 597.63 kJ / mol | ||||||||||||||||||||||||||||||||||||||||||||||||||||||||||||||||||||||||||||||||||||||||||||||||||||||
2. Ionization energy | 11.6 (4) eV ≈ 1 120 kJ / mol | ||||||||||||||||||||||||||||||||||||||||||||||||||||||||||||||||||||||||||||||||||||||||||||||||||||||
3. Ionization energy | 19th.8 (3) eV ≈ 1 910 kJ / mol | ||||||||||||||||||||||||||||||||||||||||||||||||||||||||||||||||||||||||||||||||||||||||||||||||||||||
4. Ionization energy | 36.7 (1.0) eV ≈ 3 540 kJ / mol | ||||||||||||||||||||||||||||||||||||||||||||||||||||||||||||||||||||||||||||||||||||||||||||||||||||||
5. Ionization energy | 46.0 (1.9 eV) ≈ 4 440 kJ / mol | ||||||||||||||||||||||||||||||||||||||||||||||||||||||||||||||||||||||||||||||||||||||||||||||||||||||
Physically | |||||||||||||||||||||||||||||||||||||||||||||||||||||||||||||||||||||||||||||||||||||||||||||||||||||||
Physical state | firmly | ||||||||||||||||||||||||||||||||||||||||||||||||||||||||||||||||||||||||||||||||||||||||||||||||||||||
Modifications | 3 | ||||||||||||||||||||||||||||||||||||||||||||||||||||||||||||||||||||||||||||||||||||||||||||||||||||||
Crystal structure |
orthorhombic ( room group Cmcm ( room group no.63 ) ) |
||||||||||||||||||||||||||||||||||||||||||||||||||||||||||||||||||||||||||||||||||||||||||||||||||||||
density | 19.16 g / cm 3 | ||||||||||||||||||||||||||||||||||||||||||||||||||||||||||||||||||||||||||||||||||||||||||||||||||||||
Mohs hardness | 2.5-3 | ||||||||||||||||||||||||||||||||||||||||||||||||||||||||||||||||||||||||||||||||||||||||||||||||||||||
magnetism | paramagnetic ( Χ m = 4.1 · 10 −4 ) | ||||||||||||||||||||||||||||||||||||||||||||||||||||||||||||||||||||||||||||||||||||||||||||||||||||||
Melting point | 1406 K (1133 ° C) | ||||||||||||||||||||||||||||||||||||||||||||||||||||||||||||||||||||||||||||||||||||||||||||||||||||||
boiling point | 4203 K (3930 ° C) | ||||||||||||||||||||||||||||||||||||||||||||||||||||||||||||||||||||||||||||||||||||||||||||||||||||||
Molar volume | 12.49 10 −6 m 3 mol −1 | ||||||||||||||||||||||||||||||||||||||||||||||||||||||||||||||||||||||||||||||||||||||||||||||||||||||
Heat of evaporation | 417.1 kJ / mol | ||||||||||||||||||||||||||||||||||||||||||||||||||||||||||||||||||||||||||||||||||||||||||||||||||||||
Heat of fusion | 15.5 kJ mol −1 | ||||||||||||||||||||||||||||||||||||||||||||||||||||||||||||||||||||||||||||||||||||||||||||||||||||||
Speed of sound | ~ 3400 ( long. ), ~ 2000 ( trans. ) Ms −1 at 293 K |
||||||||||||||||||||||||||||||||||||||||||||||||||||||||||||||||||||||||||||||||||||||||||||||||||||||
Specific heat capacity | 116 J kg −1 K −1 at 298 K | ||||||||||||||||||||||||||||||||||||||||||||||||||||||||||||||||||||||||||||||||||||||||||||||||||||||
Electric conductivity | 3.24 · 10 6 A · V −1 · m −1 at 293 K | ||||||||||||||||||||||||||||||||||||||||||||||||||||||||||||||||||||||||||||||||||||||||||||||||||||||
Thermal conductivity | 27.6 W m −1 K −1 at 300 K | ||||||||||||||||||||||||||||||||||||||||||||||||||||||||||||||||||||||||||||||||||||||||||||||||||||||
Chemically | |||||||||||||||||||||||||||||||||||||||||||||||||||||||||||||||||||||||||||||||||||||||||||||||||||||||
Oxidation states | +3, +4, +5, +6 | ||||||||||||||||||||||||||||||||||||||||||||||||||||||||||||||||||||||||||||||||||||||||||||||||||||||
Normal potential | −1.660 V (U 3+ + 3 e - → U) | ||||||||||||||||||||||||||||||||||||||||||||||||||||||||||||||||||||||||||||||||||||||||||||||||||||||
Electronegativity | 1.38 ( Pauling scale ) | ||||||||||||||||||||||||||||||||||||||||||||||||||||||||||||||||||||||||||||||||||||||||||||||||||||||
Isotopes | |||||||||||||||||||||||||||||||||||||||||||||||||||||||||||||||||||||||||||||||||||||||||||||||||||||||
|
|||||||||||||||||||||||||||||||||||||||||||||||||||||||||||||||||||||||||||||||||||||||||||||||||||||||
For other isotopes see list of isotopes | |||||||||||||||||||||||||||||||||||||||||||||||||||||||||||||||||||||||||||||||||||||||||||||||||||||||
Hazard and safety information | |||||||||||||||||||||||||||||||||||||||||||||||||||||||||||||||||||||||||||||||||||||||||||||||||||||||
![]() Radioactive |
|||||||||||||||||||||||||||||||||||||||||||||||||||||||||||||||||||||||||||||||||||||||||||||||||||||||
|
|||||||||||||||||||||||||||||||||||||||||||||||||||||||||||||||||||||||||||||||||||||||||||||||||||||||
As far as possible and customary, SI units are used. Unless otherwise noted, the data given apply to standard conditions . |
Uranium (named after the planet Uranus ) is a chemical element with the element symbol U and the atomic number 92. In the periodic table it is in the group of actinides ( 7th period , f-block ). Uranium is a metal whose isotopes are all radioactive . Uranium naturally occurring in minerals consists of about 99.3% of the isotope 238 U and 0.7% of 235 U.
Uranium gained special importance after the discovery of nuclear fission in 1938. The uranium isotope 235 U can be fissioned by thermal neutrons and is therefore the only naturally occurring nuclide with the one , in addition to the extremely rare plutonium isotope 239 Pu, which can be produced from uranium self-sustaining nuclear fission chain reaction is possible. It is therefore used as a primary energy source in nuclear power plants and nuclear weapons .
history

Uranium was isolated from the mineral pitchblende in 1789 by the German chemistry professor and pharmacist Martin Heinrich Klaproth , who was then living in Berlin . It is named after the planet Uranus (and thus after the Greek god Uranos ), who was discovered eight years earlier (1781) by Friedrich Wilhelm Herschel . On September 24, 1789, Klaproth announced the discovery in a speech to the Prussian Academy of Sciences . His discovery was first called uranite , then renamed uranium in 1790 . Klaproth made his discovery while analyzing the ore from the " Georg Wagsfort " mine in Wittigsthal near Johanngeorgenstadt in Saxony . He treated the ore with acid and heated it strongly. The result was a black powder that he called uranium.
Klaproth had indeed identified a new element, but what he had gained was not the element uranium itself, but an oxide . It was not until fifty years later in 1841 that the French Eugène Peligot succeeded in extracting pure uranium metal. In the first half of the 19th century uranium was mined along with other minerals in St. Joachimsthal and in some mines in Cornwall (England).
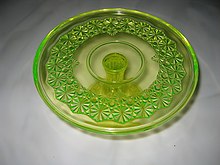
Uranium compounds were throughout the 19th century to the coloring of glass and ceramics used to vases and decorative items, as well as everyday objects to give as bowls glasses etc. a yellow-green color ( "anna green"). Glass manufacturers in Joachimsthal (Bohemia) used this technology as early as 1826. Uranium was still used for coloring glass until the middle of the 20th century, only then was it replaced by other, less questionable coloring minerals. Uranium-containing ceramic glazes from orange to bright red have been used for dishes and architectural accessories.
In photography, uranyl nitrate was used for the brown and red tinting of slide plates, platinum pictures and bromide silver pictures until well into the 20th century .
Antoine Henri Becquerel first established that uranium is radioactive in 1896 .
Uranium has long been considered the element with the highest atomic number that occurs naturally. In 1971, however, the tiniest traces of the plutonium isotope 244 Pu were detected, so that plutonium (Z = 94) replaced uranium as a known natural element with the highest atomic number.
Occurrence
Uranium is not dignified in nature, but always in oxygen-containing minerals. Important uranium minerals include brannerite and uraninite (oxides), torbernite , heinrichite and carnotite (phosphates, arsenates and vanadates) as well as coffinite and uranophane (silicates). There are around 230 uranium minerals in total, which can also be of local economic importance. Pseudomorphoses of uranium minerals (usually uraninite in the form of pitchblende) after fossil wood or bacteria can also form in sedimentary deposits .
The two decisive factors for the distribution of the radioactive element uranium on earth are, on the one hand, the lithophilic character of the element and its different mobility in aqueous solutions under oxidizing and reducing conditions. The lithophilic character ensures that uranium accumulates in silicate-rich melts . Therefore, Felsic magmatites such as granite as plutonite or rhyolite as volcanite usually contain the highest concentrations of this element. The continental crust is the area on earth with the highest levels of uranium, averaging 2.5 ppm , while the oceanic crust and mantle have orders of magnitude lower uranium levels. In igneous rocks, uranium is usually incorporated into accessory minerals such as zircon or monazite , with which one can therefore very well date the age of the rocks.
The different solubility of uranium under oxidizing or reducing conditions in solutions is the second decisive factor for the distribution of the element and plays a major role in the formation of uranium deposits. Under oxidizing conditions (UO 2 2+ ) uranium is relatively mobile in aqueous solutions, while under reducing conditions (U 4+ ) it is sparingly soluble. Therefore, redox limits are often deposit-controlling factors for the element.
Based on the above factors and a few others, there is a wide range of uranium deposits from igneous hydrothermal to sedimentary types. The IAEA differentiates between important individual types .
The highest uranium contents are reached in discordance-bound deposits with average uranium contents of 0.3 to 20%. These are currently also the two largest uranium producers. The largest single uranium resource on earth is Olympic Dam with a proven uranium content of over 2 million tons at an average uranium content of about 0.03%. The world's first industrial-scale uranium mine in Jáchymov (Czech Republic) produced from hydrothermal veins.
The natural reactors of Oklo in Gabon as well as a neighboring uranium deposit are a specialty : it is known that chain reactions occurred there about 1.5 to 2 billion years ago over thousands of years in a natural environment, during which also plutonium isotopes were formed.
Uranium occurs as a trace element in normal soil . The US Agency for Toxic Substances and Disease Registry (ATSDR) estimates that the top 33 cm of soil of a square mile of land contains an average of about 4 tons of uranium, or about 1.5 tons per square kilometer.
Uranium bound in complexes is also a ubiquitous element in the hydrosphere . The uranium concentration in seawater is approx. 3.3 µg / l compared to the sometimes significantly lower concentrations in the rivers (0.03 µg / l in the Amazon to 3.9 µg / l in the Ganges ). This shows that uranium is accumulating in seawater. German rivers usually have uranium concentrations between approx. 1 and 3 µg / l. The source of the uranium lies in the geogenic structure of the areas drained by the rivers, e.g. B. surface water from bogs can contain higher uranium concentrations and is therefore of natural origin. Only in exceptional cases can the uranium content in rivers be attributed to human influence, e.g. the use of uranium-containing phosphate fertilizers and uranium mining ( Zwickauer Mulde : approx. 10 µg / l). In Germany, uranium is found in unaffected groundwater in concentrations of less than 1 to over 100 µg / l. Regular consumption of drinking water with high levels of uranium can lead to the occurrence of kidney cancer. For this reason, the World Health Authority (WHO) recommends a limit value of 30 µg / l for drinking water.
According to the International Atomic Energy Agency (IAEA), the largest uranium ore reserves are in the USA , Niger , Australia , Kazakhstan , Namibia , South Africa , Canada , Brazil , Russia , Ukraine and Uzbekistan .
Traces of uranium are also found in hard coal and lignite. The coal used annually for power generation worldwide contains around 10,000 t of uranium and 25,000 t of thorium , which either end up in the environment or accumulate in power plant ash and filter dust. There have been isolated efforts to extract uranium from power plant ash.
The connection between the increased uranium content in mineral and drinking water and the geology of the groundwater storage rocks was examined nationwide for the first time in 2009. It turned out that increased uranium contents are mainly linked to formations such as red sandstone or Keuper, which themselves have geogenically increased uranium contents. However, uranium levels from agricultural phosphate fertilization have already found their way into the groundwater, because rock phosphates contain 10–200 mg / kg uranium, which can lead to an input of approx. 5 g / ha / a uranium when properly fertilized.
Dismantling
In Germany, uranium first became conventional in Saxon Switzerland ( Königstein ) and later through leaching , in Dresden ( Coschütz / Gittersee especially in Gittersee ) and in the Ore Mountains ( Schlema , Schneeberg , Johanngeorgenstadt , Pöhla ) as well as in East Thuringia ( Ronneburg ) mostly underground as pitchblende mined by the SDAG bismuth . Small amounts were also extracted in the Black Forest and the Fichtel Mountains . The GDR was at that time the world's third largest uranium producer. Most of the mining areas were closed after 1990, as they were uneconomical due to the low world market price and the demand for uranium declined due to the changed political world situation (less importance of strategic nuclear weapons).
The world production of uranium in 2006 was 39,603 tons. Major producing countries are Australia, Canada, Russia, Niger, Namibia, Kazakhstan, Uzbekistan, South Africa and the USA. Consumption worldwide was 66,500 tons in 2006 and is estimated by the International Atomic Energy Agency (IAEA) to be 93,775 to 121,955 tons in 2030 due to the construction of new nuclear power plants. The mining covers about 60% of the current demand, the rest is covered by stocks, reprocessing and disarmed nuclear weapons. Estimates by the IAEA, Greenpeace and the nuclear industry about the range of uranium deposits are based on different information about global resources and future consumption. They are between 20 and 200 years.
Uranium mining brings uranium and radioactive decay products (e.g. the radioactive noble gas radon ) from the underground to the surface. The associated release leads to damage to the environment and health.
presentation
Processing of uranium ore
Uranium ores, e.g. B. uraninite (pitchblende, U 3 O 8 ) or carnotite (KUO 2 VO 4 · 1.5 H 2 O), are digested acidic with sulfuric acid or alkaline with soda .
The solutions resulting from the acidic digestion are treated with ammonia, whereupon the yellow cake precipitates. This mainly contains ammonium diuranate ((NH 4 ) 2 U 2 O 7 ) and other polyuranates , uranyl hydroxides and sulphates . The solution of the alkaline digestion is mixed with NaOH , so that sodium diuranate (Na 2 U 2 O 7 ) precipitates. To remove the sodium, it is then dissolved in H 2 SO 4 and then precipitated as (NH 4 ) 2 U 2 O 7 with aqueous NH 3 .
The "yellow cake" is dissolved in nitric acid (HNO 3 ), whereby insoluble parts precipitate and are removed by filtration or centrifugation. Crude uranyl nitrate (UO 2 (NO 3 ) 2 ) can then be crystallized out of the solution. A solution of uranyl nitrate is then extracted with tributyl phosphate (TBP) ( PUREX process ), after evaporation and washing pure uranyl nitrate is obtained.
Careful pyrolysis leads to the various modifications of uranium (VI) oxide (UO 3 ), depending on the temperature and oxygen pressure. To reduce weight during transport, the “yellow cake” is thermally decomposed, resulting in black U 3 O 8 .
Powdered yellow cake
Yellowcake and
uranyl nitrate solution
UO 2 (NO 3 ) 2
+ Ammonium diuranate
(NH 4 ) 2 U 2 O 7
+ Uranium (IV) oxide
UO 2
Pure representation
Uranium (VI) oxide (UO 3 ) is reduced to uranium (IV) oxide (UO 2 ) with hydrogen . If uranium dioxide is reacted with anhydrous hydrogen fluoride , uranium tetrafluoride is formed , from which pure uranium is finally obtained by reduction using calcium or magnesium :
Uranium can generally be produced by reducing uranium halides with alkali or alkaline earth metals:
Electrolysis of KUF 5 or UF 4 in molten calcium chloride (CaCl 2 ) / sodium chloride (NaCl) can also take place. Very pure uranium can be produced by the thermal decomposition of uranium halides on a glow wire. From uranium dioxide it is u. a. obtainable by reduction with calcium.
properties
Physical Properties
Uranium is a relatively soft, silver-white, high- density metal that comes in three modifications.
phase | stable temperature range |
Density (temp.) |
Crystal system (axes in picometers ) |
---|---|---|---|
α-uranium | below 688 ° C |
orthorhombic (a = 285.4, b = 586.9, c = 495.6) |
|
β-uranium | 688 ° C to 776 ° C |
tetragonal (a = 1075.9, c = 565.6) |
|
γ-uranium | above 776 ° C |
cubic (a = 352.5) |
Uranium-rhodium-germanium (URhGe) is the first alloy to be discovered that shows invariant superconductivity in very strong magnetic fields .
Chemical properties
Uranium is self-igniting in a finely divided state . Most acids dissolve metallic uranium, while bases do not attack it. In the air, the metal is covered with an oxide layer .
Uranium forms a number of compounds in which it can exist in the oxidation states +2 to +6. The color of uranium complexes is usually highly dependent on the oxidation number , but also on the ligand environment . In aqueous solution as well as in solid compounds , the following combinations of color and oxidation state are often observed: U 3+ (violet), U 4+ (green), U V O 2 + (pale purple) and U VI O 2 2+ ( yellow). Other color combinations often result in non-aqueous solutions with organic ligands . Uranium occurs predominantly in nature with the valences +4 or +6. Quadrivalent uranium minerals are almost insoluble in water under normal pH / EH conditions. Uranium compounds are poisonous. The toxicity is v. a. determined by their solubility. The easily soluble uranyl salts are the most toxic, the poorly soluble oxides are less toxic. Uranium is teratogenic .
Biological aspects
In the case of the genus Desulfovibrio , the ability to use uranium as an electron acceptor has been demonstrated: uranium (VI) is reduced to uranium (IV). Desulfovibrio vulgaris uses cytochrome c3 as a uranium reductase. If uranium (VI) is the only electron acceptor that can be used by the bacterium, however, no growth was observed. A bacterium that can use uranium (VI) as the only electron acceptor and also shows growth is Geobacter metallireducens from Geobacteraceae .
Insoluble uranium can be mobilized through bacterial activity. Under aerobic conditions, the iron-sulfur bacteria Acidithiobacillus ferrooxidans and Leptospirillum ferrooxidans can oxidize pyrite (FeS 2 ) to iron (II) sulfate (FeSO 4 ) and then to iron (III) sulfate (Fe 2 (SO 4 ) 3 ) . Iron (III) ions can oxidize insoluble uranium (IV) to soluble uranium (VI).
The reduction of soluble uranium (VI) to insoluble uranium (IV) by prokaryotes has been proposed as a possible method for the biological remediation of uranium-contaminated groundwater and hazardous waste.
Isotopes
At present, 25 isotopes and 3 nuclear isomers with half-lives between 1 µs and 4.468 billion years are known of uranium . Of these, only the four most long-lived isotopes occur in nature. Of these, 238 U and 235 U come from the time the solar system was formed; they were formed in supernovae in the r process . 234 U is formed in several intermediate stages during the decay from 238 U, 236 U through rare neutron capture from 235 U. The artificially producible fifth-longest-lived isotope 233 U also plays a role in technology. All other isotopes have half-lives of a maximum of 68.9 years.
In natural uranium ( natural uranium ), therefore, the isotope found 238 U to 99.27%, 235 U to 0.72%, 234 U to 0.0055%, and 236 U in traces. The isotope ratio of the uranium isotopes changes over time, as 238 U and 235 U decay at different rates. The frequency of the third natural isotope 234 U remains constant in relation to the frequency of 238 U, since 234 U is a decay product of 238 U and is in equilibrium with it.
Another ratio of uranium isotopes can be found in the area of natural reactors, of which Oklo in Gabon is the first to be discovered and therefore the best known. With today's isotope ratio of 235 U and 238 U, such a reactor zone is no longer possible.
- 238 U has a half-life of 4.468 billion years and, like the other natural isotopes ( 234 U and 235 U), is an α-emitter . The specific activity of 238 U is 12,450 Bq / g. 238 U is the natural beginning of the uranium-radium series .
- 235 U has a half-life of 703.8 million years. It is the natural start of the uranium actinium series . It is fissile and has a share of around 0.7% in natural uranium deposits. Because of its fissile nature, it is of great economic importance.
- 234 U has a half-life of 245,500 years. Because of its relatively short half-life compared to 238 U, it is only present in traces, but makes an equally large contribution to radioactivity as the latter. It arises according to:
- The times given are half-lives .
- 236 U is an α emitter with a half-life of 23.42 million years and only occurs in traces in nature. It is created by neutron capture from 235 U. When uranium is exposed to an increased neutron flux, such as B. in a nuclear reactor, the proportion of 236 U increases significantly. The proportions of the isotopes 234 U, 235 U, 236 U in a uranium contamination can provide information about its origin. 236 U disintegrates over the thorium series which has been extended to the natural plutonium 244 Pu .
- 233 U has a half-life of 159,200 years and is fissile. It is not contained in natural uranium, but is incubated in breeder reactors such as the THTR-300 from the difficult to split thorium 232 Th (splitting cross section 3 µb, as in the 238 U). 233 U breaks down into the Neptunium series .
- The times given are half-lives .
Cleavage
The cross-section for induced nuclear fission by a thermal neutron is large at 233 U and 235 U with 530 and 586 b ( Barn ), respectively , at 238 U, however, very small at only 3 µb. In the technical-practical sense, only the isotopes 233 and 235 are “easily fissile” and thus possible fuels for nuclear reactors .
Uranium is designated as “enriched” if its share of 235 U has been increased compared to 238 U through uranium enrichment . Weakly enriched uranium (in the technical jargon "LEU", lightly enriched uranium) is used in nuclear power plants , highly enriched uranium ("HEU" highly enriched uranium) for research purposes, in medicine, in the reactors of the US Navy and for the manufacture of nuclear weapons . The limit between LEU and HEU is usually set at an enrichment level of 235 U of 20%.
The critical mass of 235 U is about 49 kg; here, the cross-section of the rapid splitting is decisive, since there is no moderator . With a 20 cm thick water reflector, the critical mass can be reduced to 22 kg, with a 30 cm steel reflector to 16.8 kg. In an aqueous solution, the critical mass can be reduced to less than 600 g with an optimal density of 0.059 g / cm³ with a reflector.
The critical mass of 233 U is only around 16 kg. Here, too, the critical mass can be lowered with a reflector: around 7.4 kg with 20 cm water and 6.2 kg with 30 cm steel. The critical mass can be reduced to 425 g in aqueous solution. The isotope can be hatched in nuclear reactors from 232 Th by neutron capture and two subsequent beta decays.
The isotope with the lowest critical mass (3.6 kg) is 232 U. Like all even-numbered uranium isotopes, it can be split almost exclusively by fast neutrons. With a steel reflector, the critical mass can be reduced to 1.9 kg. The isotope is, however, very difficult to obtain, since it cannot be produced in a nuclear reactor.
The fourth uranium isotope that can sustain a chain reaction is 234 U. Its critical mass is 145 kg. It is contained in natural uranium as a by-product of 238 U and can also be bred from 233 U or 232 Th. Because of the high critical mass and the laborious extraction compared to other fissile isotopes, it does not play a major role in nuclear technology. In most enrichment methods, however, it is enriched even more than 235 U in relative terms, but this is mostly of no consequence due to the low initial concentration .
The isotopes 236 U and 238 U cannot themselves carry a chain reaction. However, 238 U is used as breeding material in connection with other, fissile isotopes in a fast breeder . The capture of neutrons and the subsequent beta decays then produce 239 Pu , which in turn can be easily split.
Use and distribution
Civil Uses
The uranium isotope 235 U is used in nuclear power plants to generate energy. The isotope 238 U can be used in breeder reactors to produce plutonium . The isotope 235 U occurs only in low concentrations (around 0.72%) in natural uranium and is concentrated through enrichment . The remaining fraction is called depleted uranium ( depletion ).
When a 235 U atomic nucleus is split , an average of 210 MeV of energy is released. Of this, about 190 MeV can be thermally utilized in a reactor. The splitting of 1 g of 235 U therefore provides around 0.95 MWd (megawatt days) = 22.8 MWh of thermal energy.
This corresponds to a theoretically usable energy content of 78 tera joules or 2.7 million SKE per kg 235 U. The amount of electricity actually generated from 1 kg of natural uranium depends on the type of reactor used and the fuel cycle and is around 36–56 MWh for the case of direct final disposal of the spent fuel elements, i.e. without reprocessing and without breeding . (The energy expenditure for mining, possible enrichment, transport and final storage is not taken into account.)
Due to its high absorption effect for ionizing radiation is depleted uranium ( depleted uranium , DU) in the radiation protection used as shielding material. DU is used for aircraft trim weights and keel weights for high performance sailboats due to its high density. After the discussion sparked by the crash of a cargo plane in Amsterdam , it is being replaced by tungsten in airplanes .
In the first half of the 20th century in particular, uranium was also very popular in the USA as a component of vaseline glass and uranium glazes for ceramics .
Military uses
Nuclear reactors are used to power large warships, such as aircraft carriers and submarines. However, few countries have nuclear-powered warships. Each of the 10 Nimitz-class aircraft carriers of the US Navy has 2 reactors with 140 MW each.
Along with plutonium, 235 U is the most important raw material for the construction of nuclear weapons and primers for hydrogen bombs .
Many armed forces use uranium ammunition . This is armor-piercing ammunition that contains depleted uranium as projectile core material. The ammunition is atomized when it enters the tank interior and burns explosively. The resulting dusts and aerosols are poisonous and damage the health of contaminated people. Uranium bullets are not hard core bullets , but belong to the more general supergroup of impact bullets . Uranium is just as dense as tungsten , but less hard and melts earlier. Uranium is available to nuclear weapon states as a waste product from enrichment. The procurement and processing are cheaper than the alternative tungsten.
Recently, several hundred parties have been involved in Indian-Pakistani border conflicts, in Chechnya , during the Soviet invasion of Afghanistan , in the Kosovo war , in the Second Gulf War and in the military invasion of the United States and other states into Iraq ( Third Gulf War ) Tons of uranium ammunition used.
Depleted uranium is also used as armor in tanks such as the M1 Abrams . It is sandwich armor with a layer of uranium between two layers of armor steel. One consequence of the military use is the legal and illegal distribution of uranium and plutonium-containing material.
links
→ Category: uranium compound
Oxidation states
Uranium forms a number of compounds in which it can exist in the oxidation states +2 to +6. In nature it occurs predominantly with the valences +4 or +6.
Uranium (II)
In 2013, uranium was first shown in the +2 oxidation state. The synthesis was achieved by reducing a tris (cyclopentadienyl) uranium (III) compound using in situ generated alkalides . The existence of molecular uranium (II) compounds could also be verified by the reduction of a tris (aryloxide) arene-uranium (III) complex, which was chemically generated by elemental potassium in the presence of 2.2.2- cryptand , as well as electrochemically at a potential of -2.50 V (against Fc + / Fc ) could be reduced. The two uranium (II) complexes have a different electron configuration, which is determined by the ligand field. The tris (cyclopentadienyl) uranium (II) complex has the electronic configuration [Rn] 5 f 3 6 d 1 , whereas the tris (aryloxide) arene-uranium (II) compound adopts a [Rn] -5 f 4 configuration .
Uranium (III)
The first uranium (III) compound was presented as UCl 3 by Peligot in 1842 . The U 3+ ion is a strong reducing agent (reduction potential between -2.2 and 1.5 V versus Fc + / Fc ) and releases hydrogen in the presence of water. Uranium (III) compounds are relatively stable in oxygen-free and halogen-free organic solvents and can be stored under anaerobic conditions. The synthesis of low-valent U (III) complexes is generally carried out starting from UI 3 or the U HMDS produced therefrom . For this purpose, UI 3 is synthesized from metallic uranium with elemental iodine. The special interest in low-valent uranium results from the high reactivity towards small molecules with biological and industrial relevance, such as. B. CO, CO 2 , N 2 , NO, or H 2 O.
Uranium (IV)
Of the compounds of uranium (IV), the oxide ( UO 2 ) and the four halides ( UF 4 , UCl 4 , UBr 4 and UI 4 ) are known. These are solids with high melting points above 500 ° C.
Uranium (V)
Uranyl (V) compounds have been known in the solid state since 2003. Since then, a large number of other uranyl (V) compounds have been synthesized.
Uranium (VI)
The hexavalent uranium occurs in nature exclusively in the form of the UO 2 2+ group (uranyl group), i. i.e. there is no U 6+ . This group preferentially binds to oxygen-containing binding partners: as phosphate, sulfate, carbonate and with water as the hydroxide. Uranyl acetate and uranyl nitrate are soluble uranium salts. These salts are commercially available and have the same toxicity as other heavy metal nitrates and acetates.
Oxides
→ Overview: uranium oxides
Uranium dioxide (UO 2 ) is a black, crystalline powder that was used as a ceramic glaze from the late 19th century to the middle of the 20th century. Nowadays it is mainly used as a nuclear fuel in fuel rods. Also known are uranium trioxide (UO 3 ), triuranium octoxide (U 3 O 8 ) and uranyl peroxide (UO 2 O 2 ).
Yellowcake

Yellowcake is a uranium oxide concentrate. The name is derived from the earlier color and texture. Nowadays, higher calcination temperatures are used, which means that the “yellow cake” tends to be dark green to black. Originally, the chemical compounds contained in Yellowcake were unknown. It was believed to be ammonium diuranate or sodium diuranate . The composition varied and depended on the smelting process. The compounds found in Yellowcake include uranyl hydroxide , uranyl sulfate , sodium para- uranium, and uranyl peroxide , along with a number of uranium oxides. Today's yellow cake typically contains 70 to 90 percent ( mass fraction ) of uranium oxide (U 3 O 8 ).
The light yellow ammonium diuranate is an intermediate product in the production of yellow cake. Sometimes it is also referred to as "yellowcake", but this is not in accordance with general usage.
Halides

For uranium, halides in the oxidation states +3 to +6 are known. For the levels +3 to +5 all compounds of the four halogens fluorine , chlorine , bromine and iodine are known, for the oxidation level +6 it is UF 6 and UCl 6 .
Oxidation number | F. | Cl | Br | I. |
---|---|---|---|---|
+6 |
Uranium (VI) fluoride UF 6 colorless |
Uranium (VI) chloride UCl 6 green |
||
+5 |
Uranium (V) fluoride UF 5 colorless |
Uranium (V) chloride UCl 5 brown |
Uranium (V) bromide UBr 5 black |
( Uranium (V) iodide ) (UI 5 ) |
+4 |
Uranium (IV) fluoride UF 4 green |
Uranium (IV) chloride UCl 4 green |
Uranium (IV) bromide UBr 4 brown |
Uranium (IV) iodide UI 4 black |
+3 |
Uranium (III) fluoride UF 3 purple |
Uranium (III) chloride UCl 3 red |
Uranium (III) bromide UBr 3 red |
Uranium (III) iodide UI 3 black |
Uranium tetrafluoride (UF 4 ), also known as “green salt” , is an intermediate product in the manufacture of uranium hexafluoride.
Uranium hexafluoride (UF 6 ) is a white solid that sublimes at a temperature of 56.5 ° C and only forms a liquid phase under a pressure of at least 1.5 bar and 64.1 ° C. UF 6 is the uranium compound that is used for the two most common enrichment processes , gas diffusion and gas centrifuge . It is simply referred to as a "witch" in the industry.
Organometallic compounds
Uranocene U (C 8 H 8 ) 2 is one of the first organourane compounds and the best-known compound of cyclooctatetraene with the f elements . The derivative U (C 8 H 4 Ph 4 ) 2 and the cycloheptatrienyl ion [U (C 7 H 7 ) 2 ] - should also be mentioned, for example, in the air .
Analytics
Classical qualitative analysis of uranium
Uranium usually occurs in solution as a UO 2 2+ cation. In the inorganic separation process, UO 2 2+ is detected in the ammonium sulfide urotropin group . After numerous separation and precipitation steps, it is extracted as UO 2 (SCN) 2 · 3 ether into an ether phase.
The detection is carried out by adding yellow blood liquor salt (K 4 [Fe (CN) 6 ]), whereby a mixed complex forms at relatively high concentrations (K 2 (UO 2 [Fe (CN) 6 ])). This falls out as a red-brown precipitate.
Instrumental quantitative analysis of uranium
Thermally excited optical spectroscopy
Atomic absorption spectrometry (AAS) in the form of flame AAS and inductively coupled plasma optical emission spectrometry ICP-OES are used for analysis only in rare exceptions due to their low sensitivity. The graphite furnace AAS is only rarely used because of disturbances caused by strong carbide formation.
Inductively coupled plasma mass spectrometry (ICP-MS)
With ICP-MS, uranium is measured very sensitively, the three naturally occurring uranium isotopes can be determined directly. So z. B. the isotope 238 U (99.274%) in human hair samples can be determined by ICP-MS with a detection limit of 0.2 ng / g.
Neutron Activation Analysis (NAA)
In the NAA, the activation reaction 238 U (n, γ) 239 U is used for the determination of uranium . 239 U has a half-life of 23.5 min. The photopeak with a gamma radiation energy of 74 keV is used for the quantitative evaluation. With this highly sensitive method, a detection limit of 4 pg / ml uranium in sea water was achieved.
Fluorescence spectrometry
Uranium in the form of uranyl ions (UO 2 2+ ) can be determined quantitatively with the help of time-resolved, laser-induced fluorescence spectrometry (TR-LIF). This method is often used in the monitoring and detection of uranium in groundwater and surface water in the vicinity of processing plants, as it offers the possibility of on-line monitoring. Detection limits of 40 ng / L were achieved with this technique.
Adsorptive Cathodic Stripping Voltammetry (AdCSV)
The AdCSV is increasingly being used for the determination of uranium in sewage, groundwater and drinking water. The UO 2 2+ ion is complexed with chloranilic acid in an acidic environment and electrochemically enriched by adsorption at 150 mV on a hanging mercury drop electrode (HMDE). A voltammogram from 50 mV to −200 mV is then recorded. The reduction peak is around −90 mV. A detection limit of 24 ng / l was achieved with this method. Due to the very good selectivity, the process was standardized as a standard process (DIN 38406-17).
safety instructions

Because of its radioactivity, uranium is dangerous and, like most heavy metals, chemically toxic.
Water-soluble uranium compounds, which, like lead , cadmium and mercury, primarily damage the tubules of the kidneys , are chemically dangerous . In 2003, the World Health Organization ( WHO) recommended - in view of the use of depleted uranium in uranium ammunition - a limit value for the daily intake of soluble uranium compounds of 0.5 μg / kg body weight, 5 μg / kg for insoluble compounds and a maximum of 1 μg / m³ in the Ambient air when absorbed via the respiratory tract. When uranium and uranium compounds are taken orally, between 0.2 and 2% is absorbed, when inhaled about 5%, the rest is excreted in the urine.
Its radioactivity generates ionizing radiation , which can trigger genetic changes - such as mutations - and subsequent cancer . The long-lived uranium isotopes are α-emitters which , if absorbed into the body, result in a relatively high radiation dose. When handling and storing uranium and its compounds, it should be noted that there are secondary products from the uranium decay series that also emit beta and penetrating gamma rays; as well as radon , which finds its way everywhere as a gas.
literature
- Ingmar Grenthe, Janusz Drożdżyński, Takeo Fujino, Edgar C. Buck, Thomas E. Albrecht-Schmitt, Stephen F. Wolf: Uranium. In: Lester R. Morss, Norman M. Edelstein, Jean Fuger (Eds.): The Chemistry of the Actinide and Transactinide Elements. Springer, Dordrecht 2006, ISBN 1-4020-3555-1 , pp. 253-698 ( doi: 10.1007 / 1-4020-3598-5 5 ).
- Walter D. Loveland, David Morrissey, Glenn T. Seaborg: Modern Nuclear Chemistry. Wiley-Interscience, 2006, ISBN 0-471-11532-0 .
- Robert J Schwankner, Gerolf Lieckfeld, Doris Lienert: The early history of uranium. In: The Geosciences. 7, 8, 1989, pp. 215-224 ( doi: 10.2312 / geoswissenschaften.1989.7.215 ).
Web links
- Entry to uranium. In: Römpp Online . Georg Thieme Verlag, accessed on January 3, 2015.
- Uranium: Human Health Fact Sheet ( Memento of 6 February 2004 at the Internet Archive ) (Engl.) (PDF, 46 kB)
- Peter Eichstaedt: Uranium , Chemical & Engineering News, 2003
Individual evidence
- ↑ a b c d e f g Harry H. Binder: Lexicon of chemical elements. S. Hirzel Verlag, Stuttgart 1999, ISBN 3-7776-0736-3 , pp. 674-682.
- ↑ The values of the atomic and physical properties (info box) are taken from www.webelements.com (Uranium) , unless otherwise stated .
- ↑ CIAAW, Standard Atomic Weights Revised 2013 .
- ↑ a b c d e Entry on uranium in Kramida, A., Ralchenko, Yu., Reader, J. and NIST ASD Team (2019): NIST Atomic Spectra Database (ver. 5.7.1) . Ed .: NIST , Gaithersburg, MD. doi : 10.18434 / T4W30F ( https://physics.nist.gov/asd ). Retrieved June 13, 2020.
- ↑ a b c d e entry on uranium at WebElements, https://www.webelements.com , accessed on June 13, 2020.
- ↑ a b c d e A. F. Holleman , E. Wiberg , N. Wiberg : Textbook of Inorganic Chemistry . 102nd edition. Walter de Gruyter, Berlin 2007, ISBN 978-3-11-017770-1 , p. 2149.
- ↑ Robert C. Weast (Ed.): CRC Handbook of Chemistry and Physics . CRC (Chemical Rubber Publishing Company), Boca Raton 1990, ISBN 0-8493-0470-9 , pp. E-129 to E-145. Values there are based on g / mol and given in cgs units. The value specified here is the SI value calculated from it, without a unit of measure.
- ^ Entry on uranium in the GESTIS substance database of the IFA , accessed on August 9, 2016(JavaScript required) .
- ↑ Entry on uranium in the Classification and Labeling Inventory of the European Chemicals Agency (ECHA), accessed on August 1, 2016. Manufacturers or distributors can expand the harmonized classification and labeling .
- ↑ The hazards emanating from radioactivity do not belong to the properties to be classified according to the GHS labeling.
- ↑ Fritz Schmidt: Compendium of practical photography . 10th, significantly improved edition. Leipzig 1906. Uranium nitrate p. 191, Urantonung p. 268, 291, 339.
- ↑ DC Hoffman, FO Lawrence, JL Mewherter, FM Rourke: Detection of Plutonium-244 in Nature. In: Nature . Volume 234, 1971, pp. 132-134 ( doi: 10.1038 / 234132a0 ).
- ↑ Helmut Tonndorf: Metallogeny of Uranium in the East German Zechstein - A contribution to the detection and characteristics of material redistribution and enrichment processes . (= Treatises of the Saxon Academy of Sciences in Leipzig, mathematical and natural science class. Volume 58, Issue 3). Akademie Verlag, Berlin 1994, ISBN 3-05-501621-1 .
- ^ Geology of Uranium deposits .
- ↑ K. Ehrig, BHP Billiton. Presentation at the South Australia Explorers Conference, Adelaide 2008.
- ↑ Various authors: Uranium Mining in the Erzgebirge and Cold War . Selected contributions from the RADIZ workshop on October 10th and 11th 1997 in Schlema, RADIZ-Information 16/1998, RADIZ eV, Schlema.
- ^ Broder Merkel, Britta Planer-Friedrich, Christian Wolkersdorfer (eds.): Uranium in the Aquatic Environment. Springer-Verlag , Heidelberg 2002, ISBN 3-540-43927-7 .
- ^ World Nuclear Association: Naturally-Occurring Radioactive Materials (NORM) ; March 2009.
- ↑ F. Knolle: A contribution to the occurrence and origin of uranium in German mineral and tap water. TU Braunschweig, 2009 (PDF)
- ^ A b OECD Nuclear Energy Agency and International Atomic Energy Agency : Uranium 2007: Resources, Production and Demand . OECD Publishing, 2008, ISBN 978-92-64-04768-6 (English, online [PDF; accessed July 7, 2009]).
- ↑ Uranium has become a sought-after raw material . In: FAZ. January 6, 2006.
- ↑ The dirtiest nuclear fuel, questions and answers about the origin of uranium ( PDF; 790 kB ( Memento from January 30, 2012 in the Internet Archive )).
- ^ Federation of American Scientists: Uranium Production .
- ^ Frank Settle: Nuclear Chemistry, Uranium Production. ( Memento from June 25, 2013 in the web archive archive.today )
- ^ Carl Willis: Uranium Chemistry .
- ^ AF Holleman , E. Wiberg , N. Wiberg : Textbook of Inorganic Chemistry . 102nd edition. Walter de Gruyter, Berlin 2007, ISBN 978-3-11-017770-1 , p. 1950.
- ↑ a b Georg Brauer: Uranium . In: Handbook of Preparative Inorganic Chemistry . Ferdinand Enke Verlag, Stuttgart 1954, p. 1069-1073 .
- ^ CR Hammond: The Elements . In: Handbook of Chemistry and Physics . 81st edition. CRC Press, 2000, ISBN 0-8493-0481-4 ( online [PDF]).
- ↑ Wilhelm Jander: About representation of pure uranium. In: Journal of Inorganic and General Chemistry . 138 (1), 1924, pp. 321-329 ( doi: 10.1002 / zaac.19241380131 ).
- ↑ Georg Brauer (Ed.): Handbook of Preparative Inorganic Chemistry . 3., reworked. Edition. tape II . Enke, Stuttgart 1978, ISBN 3-432-87813-3 , p. 1195 .
- ↑ F. Levy, I. Sheikin, B. Grenier, AD Huxley: Magnetic Field-Induced Superconductivity in the ferromagnet URhGe. In: Science . 309, 2005, pp. 1343-1346 ( doi: 10.1126 / science.1115498 ).
- ^ AF Holleman , E. Wiberg , N. Wiberg : Textbook of Inorganic Chemistry . 102nd edition. Walter de Gruyter, Berlin 2007, ISBN 978-3-11-017770-1 , p. 1956.
- ^ DR Lovley, PK Widman, JC Woodward, EJ Phillips: Reduction of Uranium by Cytochrome c3 of Desulfovibrio vulgaris. In: Appl. Environ. Microbiol. 59 (11), 1993, pp. 3572-3576. PMID 8285665 ; PMC 182500 (free full text).
- ^ DR Lovley, EJ Phillips: Reduction of Uranium by Desulfovibrio desulfuricans. In: Appl. Environ. Microbiol. 58 (3), 1992, pp. 850-856. PMID 1575486 ; PMC 195344 (free full text).
- ↑ DR Lovley, EJP Phillips, YA Gorby, ER Landa: Microbial Reduction of Uranium. In: Nature . 350, 1991, pp. 413-416 ( doi: 10.1038 / 350413a0 ).
- ↑ OH Tuovinen, TM Bhatti: Microbiological Leaching of Uranium Ores. In: Minerals and Metallurgical Processing . 16, 1999, pp. 51-60; doi : 10.1007 / BF03403234 .
- ^ DR Lovley: Bioremediation of Organic and Metal Contaminants with Dissimilatory Metal Reduction. In: J. Ind. Microbiol. 14 (2), 1995, pp. 85-93. PMID 7766214 .
- ^ The Biochemical Periodic Tables - Uranium.
- ↑ Nuclear Wallet Card - Z (92) (Engl.)
- ^ P. Steier, R. Golser, W. Kutschera, V. Liechtenstein, A. Priller, A. Valenta, C. Vockenhuber: Heavy ion AMS with a small accelerator, Nuclear Instruments and Methods. In: Physics Research B . 188 (1-4), 2002, pp. 283-287 ( doi: 10.1016 / S0168-583X (01) 01114-4 ).
- ↑ MAC Hotchkis, D. Child, D. Fink, GE Jacobsen, PJ Lee, N. Mino, AM Smith, C. Tuniz: Measurement of 236 U in environmental media, Nuclear Instruments and Methods. In: Physics Research B . 172 (1-4), 2000, pp. 659-665 ( doi: 10.1016 / S0168-583X (00) 00146-4 ).
- ^ DL Donohue: Strengthening IAEA safeguards through environmental sampling and analysis. In: Journal of Alloys and Compounds . 271-273, 1998, pp. 12-18 ( doi: 10.1016 / S0925-8388 (98) 00015-2 ).
- ↑ G. Pfennig, H. Klewe-Nebenius, W. Seelmann-Eggebert (eds.): Karlsruher Nuklidkarte . 7th edition. 2006.
- ↑ world-nuclear.org: Radioisotopes in Medicine ( Memento from December 26, 2013 in the Internet Archive ).
- ^ Ending the Production of Highly Enriched Uranium for Naval Reactors. (PDF 235 kB, p. 2 (87)) cns.miis.edu, accessed on April 12, 2016 (English).
- ↑ Institut de Radioprotection et de Sûreté Nucléaire : Evaluation of nuclear criticality safety data and limits for actinides in transport. P. 16 ( PDF ( Memento of November 18, 2014 in the Internet Archive )).
- ↑ Nuclear energy - where does it come from? Future workshop Jena
- ^ US Nuclear Regulatory Commission: Systematic Radiological Assessment of Exemption for Source and Byproduct Materials. Section 3.17, pp. 531–533 ( PDF; 3.3 MB ).
- ^ Daniel Rhodes: Clay and Glazes for the Potter . 2015.
- ↑ University of Oldenburg: Information on uranium ammunition .
- ↑ ES Craft, AW Abu-Qare, MM Flaherty, MC Garofolo, HL Rincavage, MB Abou-Donia: Depleted and natural Uranium: Chemistry and toxicological effects. In: Journal of Toxicology and Environmental Health - Part B - Critical Reviews . 7 (4), 2004, pp. 297-317 ( doi: 10.1080 / 10937400490452714 ).
- ^ A b Matthew R. MacDonald, Megan E. Fieser, Jefferson E. Bates, Joseph W. Ziller, Filipp Furche , William J. Evans: Identification of the +2 Oxidation State for Uranium in a Crystalline Molecular Complex, [K (2.2 .2-Cryptand)] [(C 5 H 4 SiMe 3 ) 3 U]. In: J. Am. Chem. Soc. 135, 2013, pp. 13310-13313 ( doi: 10.1021 / ja406791t ).
- ^ A b Henry S. La Pierre, Andreas Scheurer, Frank W. Heinemann, Wolfgang Hieringer, Karsten Meyer: Synthesis and Characterization of a Uranium (II) Monoarene Complex Supported by δ Backbonding. In: Angewandte Chemie International Edition . 53 (28), 2014, pp. 7158-7162 ( doi: 10.1002 / anie.201402050 ).
- ^ Henry S. La Pierre, Hajime Kameo, Dominik P. Halter, Frank W. Heinemann, Karsten Meyer: Coordination and Redox Isomerization in the Reduction of a Uranium (III) Monoarene Complex . In: Angewandte Chemie International Edition . tape 53 , no. 28 , July 7, 2014, p. 7154–7157 , doi : 10.1002 / anie.201402048 .
- ^ William J. Evans, Stosh A. Kozimor: Expanding the chemistry of U3 + reducing agents . In: Coordination Chemistry Reviews (= Actinide Chemistry ). tape 250 , no. 7 , April 1, 2006, pp. 911-935 , doi : 10.1016 / j.ccr.2006.01.017 .
- ^ Wiley: Progress in Inorganic Chemistry, Volume 58 - Kenneth D. Karlin. Retrieved October 14, 2017 .
- ↑ J.-C. Berthet, M. Nielich, M. Ephritikhine: Isolation of a Uranyl [UO 2 ] + Species: Crystallographic Comparison of the Dioxouranium (V) and (VI) Compounds [UO 2 (OPPh 3 ) 4 ] (OTf) n ( n = 1, 2) . In: Angew. Chem. Int. Ed. 42, 2003, p. 1952 ( doi: 10.1002 / anie.200250506 ).
- ^ Polly L. Arnold, Jason B. Love, Dipti Patel: Pentavalent uranyl complexes. In: Coordination Chemistry Reviews . 253 (15-16), 2009, pp. 1973-1978 ( doi: 10.1016 / j.ccr.2009.03.014 ).
- ^ AF Holleman , E. Wiberg , N. Wiberg : Textbook of Inorganic Chemistry . 102nd edition. Walter de Gruyter, Berlin 2007, ISBN 978-3-11-017770-1 , p. 1969.
- ^ A. Streitwieser, U. Müller-Westerhoff: Bis (cyclooctatetraenyl) uranium (Uranocene). A New Class of Sandwich Complexes That Utilize Atomic f Orbitals. In: J. Am. Chem. Soc. 90 (26), 1968, pp. 7364-7364 ( doi: 10.1021 / ja01028a044 ).
- ↑ Christoph Elschenbroich : Organometallchemie. 6th edition. Wiesbaden 2008, ISBN 978-3-8351-0167-8 , pp. 587-591.
- ↑ D. Seyferth: Uranocene. The First Member of a New Class of Organometallic Derivatives of the f Elements. In: Organometallics . 23 (15), 2004, pp. 3562-3583 ( doi: 10.1021 / om0400705 ).
- ↑ G. Jander, E. Blasius: Textbook of analytical and preparative inorganic chemistry. 16th edition. S. Hirzel Verlag, Stuttgart 2006, ISBN 3-7776-1388-6 .
- ^ H. Sela, Z. Karpas, C. Pickhardt, JS Becker: Biomonitoring of hair samples by laser ablation inductively couple plasma mass spectrometry (LA-ICP-MS). In: International Journal of Mass Spectrometry . 261 (2-3), 2007, pp. 199-207 ( doi: 10.1016 / j.ijms.2006.09.018 ).
- ↑ R. lobinski, Z. Marczenko: Spectrochemical Trace Analysis for Metals and metalloid. Elsevier, Amsterdam 1997.
- ↑ G. Romanovskaya, V. Pogonin, A. Chibisov: Determination of Trace Amounts of Uranium (VI) in Various material by a Repetitive Laser Technique. In: Talanta . 34 (1), 1987, pp. 207-210 ( doi: 10.1016 / 0039-9140 (87) 80028-0 ; PMID 18964281 ).
- ↑ G. Henze: Polarography - Voltammetry; Basics and analytical practice. Springer Verlag, Berlin / Heidelberg / New York 2001.
- ↑ Thomas Efferth: Molecular pharmacology and toxicology: Biological foundations of drugs and poisons. Springer, 2006, ISBN 3-540-21223-X , p. 238.
- ↑ Werner Böcker, Helmut Denk, Philipp Ulrich Heitz: Repetitorium Pathologie. Elsevier, Urban & Fischer, 2007, ISBN 978-3-437-43400-6 , p. 296.
- ↑ a b WHO: Depleted uranium: sources, exposure and health effects. Executive summary, January 2003 ( PDF; 23 kB ).