Thyroid hormones
The term thyroid hormones is used to summarize the hormones formed in the follicular epithelial cells of the thyroid gland (thyrocytes) . These include in particular triiodothyronine (T 3 ) and thyroxine (tetraiodothyronine, T 4 ). The thyroid hormones play an important role in the energy metabolism and the growth of individual cells and the entire organism and are therefore absolutely vital.
In addition to T 4 and T 3, there are other iodothyronines (non-classic thyroid hormones) with weaker and sometimes antagonistic effects.
Thyronamines are hormones that in many ways have the opposite effects of the classic thyroid hormones. There is increasing evidence that they are formed from iodothyronines.
Iodothyroacetates are deaminated iodothyronines, which occur in serum in concentrations higher than T 3 and which have partially agonistic effects on iodothyronines.
The hormone calcitonin , which is formed by the parafollicular cells (C cells) of the thyroid gland, is usually not referred to as a thyroid hormone, as it is neither structurally nor in its function related to the classic thyroid hormones.
Chemical structure
The classic thyroid hormones from the iodothyronine class are non-proteinogenic α-amino acids. They consist of the amino acid thyronine , at its aromatic ring at three (tri-iodothyronine) or four (thyroxine) positions iodinated is. Thyronine is made from two iodinated tyrosines . Thyronine and thyroxine differ in the number of their iodine atoms . The most important thyroid hormones are thyroxine and triiodothyronine ; a weakly active thyroid hormone is diiodothyronine .
The still little researched thyronamines and iodothyroacetates, which arise from iodothyronines by decarboxylation or deamination, have a similar structure .
The calcitonin formed by the C cells of the thyroid gland does not belong to the iodothyronine class, rather it is a peptide hormone .
Education and release
Biosynthesis (human)
Monoiodtyrosine and diiodtyrosine are formed in the thyroid from L - tyrosine and iodide . The L- tyrosine is in the form of tyrosyl residues bound to thyroglobulin and the iodide is bound to the tyrosyl residues with the aid of the enzyme thyroid peroxidase (TPO); When one iodine atom binds, monoiodo tyrosine is produced first, and when another iodine atom binds, diiodo tyrosine (DIT) is produced. Since the TPO contains heme as a prosthetic group , the synthesis of MIT and DIT (and consequently that of the thyroid hormones) can be restricted by an iron deficiency .
Hormone precursor (human)
In humans, monoiodtyrosine and diiodtyrosine are one of the precursors of the thyroid hormone triiodothyronine (T 3 ) and, via diiodtyrosine, the indirect precursor of the thyroid hormone L - thyroxine (T 4 ).
- T 4 : thyroxine
T 3 : 3,3 ', 5-triiodo-L-thyronine
rT 3 : 3,3', 5'-triiodo-L-thyronine
3,3'T 2 : 3,3'-diiodine-L -thyronine
MIT and DIT are bound to thyroglobulins (TG) in the thyroid gland . The globulins are the immediate precursor of thyroid hormones produced in the thyroid gland in this form. By aggregating (coupling via an ether bridge ) in the TG molecule of an MIT and a DIT molecule, T 3 (approx. 11 μg / day), by aggregating two DIT molecules T 4 (approx. 100 μg / day) educated. In the thyroid gland, T 3 is produced both in the form of 3,5,3'-triiodothyronine (T 3 , approx. 10 μg / day) and as inactive 3,3 ', 5'-triiodothyronine (rT 3 , for: reverse triiodothyronine , approx. 1 μg / day). Thyroid peroxidase is also involved in these coupling processes.
Plasma protein binding (human)
The resulting hormones are not released but released into the bloodstream in an inactive form bound to transport proteins ( TBG , TTR , TBA , TBPA , SHBG ). More than 99% of them are present in the blood (T 4 to 99.95%, T 3 to 99.7%) in a form bound to proteins and only when required does the body produce free, active hormones from the bound thyroid hormones. Free T 4 (fT 4 ) is formed by simply releasing T 4 from its protein binding.
Deiodization (conversion)
Free T 3 (fT 3 ) and inactive rT 3 are both directly released from their protein binding, but mostly indirectly with the help of thyroxine deiodinases in the cytoplasm of the target cell from free T 4 that has migrated into the cell by splitting off an iodine atom
- at the 5 'position (= T 3 , approx. 25 μg from the 100 μg T 4 generated daily ) or
- at the 5 position (= rT 3 , approx. 35 μg from the 100 μg T 4 generated daily )
won. Since the thyroxine deiodinases are selenium-containing enzymes, the synthesis of fT 3 in the target cells can be restricted by a selenium deficiency .
It should be noted that on the one hand T 4 makes up the majority of the thyroid hormones formed in the thyroid gland, on the other hand the majority of the fT 3 released in the body or in the cells is produced indirectly from fT 4 that has migrated into the target cell . So is
- DIT the main preliminary stage of both fT 3 (formed in the target cells) and T 4 / fT 4 ,
- MIT is the immediate preliminary stage of the T 3 produced in small quantities in the thyroid, but mainly the indirect preliminary stage of the thyroid hormone components produced from DIT,
- fT 4 the immediate main preliminary stage of fT 3 .
In addition to T3 and rT3, other iodothyronines such. B. 3,5-T2 produced. Enzyme-mediated deiodination processes are also involved in the conversion of non-classical thyroid hormones, ie thyronamines and iodothyroacetates .
Although DIT precursor of thyroid hormones, it can not be increased (artificial) feeding be used to increase the thyroid hormone production as it paradoxically Thyreostatikum acts.
Iodine uptake into the thyroid follicle
The synthesis of thyroid hormones requires iodine, which is taken in with food in the form of iodide ions. The thyroid depends on a regular and sufficient supply of iodine. The iodide ions are transported via the blood to the follicular epithelial cells (thyrocytes) of the thyroid gland and are absorbed by them via a transport protein , the sodium iodide symporter (NIS). The sodium-iodide symporter, which is integrated into the basolateral plasma membrane of the thyrocytes, uses the energy-dependent electrochemical concentration gradient for the sodium ions, so that iodide and sodium, are built up by the sodium-potassium pump (sodium-potassium-ATPase) -Ions can be transported together (in symport ) into the follicular epithelial cells. It is a secondary active transport .
With this transport system, significantly higher concentrations are achieved inside the cell than is the case in the extracellular space, i.e. outside the cell. The iodide concentration in blood plasma is, for example, 25 to 30 times lower than in follicular epithelial cells. The activity of the sodium iodide symporter is regulated by the thyrotropin (syn. Thyroid -stimulating hormone, TSH) formed in the pituitary gland . Thyrotropin increases the uptake of iodine by the thyrocytes.
Two independent passive transport proteins are involved in the transport of iodide ions into the lumen of the thyroid follicle, which are only localized in the apical membrane of the thyrocytes: Pendrin and the human apical iodide transporter (hAIT).
Iodination
The thyroglobulin formed by the thyrocytes through protein biosynthesis is released into the lumen of the thyroid follicle through exocytosis , i.e. through the fusion of secretory vesicles with the apical plasma membrane of the follicular epithelial cells.
Thyroglobulin is rich in tyrosine side chains. Tyrosine is an amino acid and part of thyroglobulin. The tyrosine side chains are iodinated as part of the thyroid hormone synthesis. Responsible for this is an enzyme belonging to the peroxidase group, the so-called thyroid peroxidase , which is integrated into the apical cell membrane of the thyrocytes and protrudes into the follicle lumen. It converts the very inert (inert) iodide ions (I - ) outside of the cells into a more reactive molecule through oxidation and consumption of hydrogen peroxide (H 2 O 2 ). These are probably short-lived iodonium ions (I + ). The hydrogen peroxide is formed by the NADPH-dependent oxidase (ThOx), which is also integrated in the apical membrane.
The aromatic ring of the tyrosine side chains of thyroglobulin is then iodinated, first at position 3, so that monoiodotyrosine is formed, and then at position 5 of the same side chain, so that diiodotyrosine is formed. Through the intramolecular coupling of two molecules, T 4 is initially formed via the precursors monoiodotyrosine and diiodotyrosine . T 3 is formed from this with the loss of an iodine atom . For storage, the hormones are bound to transport proteins, in particular thyroxine-binding globulin (TBG).
Release of thyroid hormones
The thyroid releases around 90–95% T 4 ( thyroxine ) and only a small amount of T 3 ( triiodothyronine ). About 80% of this is in the bloodstream and liver. The hormones are formed in the gland by the addition of iodine to the amino acids that were first synthesized and provided with a carbohydrate component . They are therefore counted among the amino acid derivatives. Thyroxine has a half-life of approx. 7 days in the body , T 3 a half-life of approx. 1 day.
Regulation of thyroid hormone synthesis and release
The release of thyroxine is increased when the thyroxine level in the blood plasma drops too much and vice versa. This regulates the maintenance of the thyroid hormone level.
The hypothalamus releases the TRH (syn. Thyroliberin or thyrotropin-releasing hormone). TRH stimulates the pituitary gland to release TSH (syn. Thyrotropin or thyroid stimulating hormone).
The TSH of the pituitary gland increases the production of the thyroid hormones T 3 and T 4 . The thyroid hormones reach the target cells via the bloodstream and develop their effect there, acting in a very similar way to steroid hormones . The hormones also reach the hypothalamus and pituitary gland via the bloodstream . These can register the T 3 and T 4 blood levels with special receptors .
In the context of the thyrotropic control loop , the formation of TRH and TSH is increasingly inhibited with increasing T 3 and T 4 levels.
The thyrotropic control loop is a multi- loop control loop between the hypothalamus , pituitary and thyroid gland . It regulates the concentration of thyroid hormones in the blood plasma .
The pituitary secretes the control hormone thyrotropin (TSH), which stimulates the secretion of thyroxine (T 4 ) and triiodothyronine (T 3 ) in the thyroid gland . The thyroid hormones in turn inhibit the sense of a negative feedback (negative feedback) the production and secretion of TSH, so that normally an equilibrium level of the amount of thyroid hormone in the blood sets. The production and release of TSH also depends on the level of thyroid liberin (TRH), which is produced and released by the hypothalamus . The hypothalamus specifies the target value for the thyroid hormones in the blood and constantly measures the actual value . In order to adapt the actual value of the thyroid hormones in the blood to the target value of the thyroid hormones in the blood, the hypothalamus can influence the production amount of TRH and thus the production amount of TSH and thyroid hormones.
Apart from this main control loop , there are other feedback loops , e.g. B. Ultra-short feedback from TSH on its own release, long feedback from thyroid hormones on TRH release and control loops that set the proportions of free (active) and - through at T 4 and T 3 Plasma protein binding - non-free (non-active) hormones.
Functional states of the pituitary-thyroid control circuit
- Euthyroidism (normal thyroid function)
-
Hypothyroidism (underactive thyroid)
- Primary hypothyroidism (control loop in the thyroid gland impaired, e.g. due to poor incretion performance after surgery or in the case of autoimmune thyroid disorders )
- Secondary hypothyroidism (impaired control loop in the pituitary gland, e.g. as part of HVL insufficiency )
- Tertiary hypothyroidism (the target value is not specified due to a lack of TRH , e.g. in the context of damage to the hypothalamus , a Pickardt syndrome or a euthyroid sick syndrome .)
-
Hyperthyroidism (overactive thyroid)
- Primary hyperthyroidism (inappropriate secretion of thyroid hormones due to a disease of the thyroid gland, e.g. in autonomy and in Graves' disease )
- Secondary hyperthyroidism (e.g. due to TSH-producing tumors of the pituitary gland)
- Tertiary hyperthyroidism (due to overproduction of TRH in the hypothalamus)
- Thyrotoxicosis (oversupply of thyroid hormones, e.g. due to excessive drug treatment of hypothyroidism)
- Thyroid hormone resistance (impairment of the control loop at the receptors in the pituitary gland or the periphery )
Medical covers
Children and adults | Free T 3 in pmol / l |
Total T 3 in µg / l |
Free T 4 in ng / dl |
Total T 4 in ng / ml |
---|---|---|---|---|
Umbilical cord blood | 1.6-3.2 | 0.4-1.3 | 1.0-1.8 | 60-131 |
1st and 2nd day of life | 5.2-14.3 | 0.8-2.6 | 1.6-3.8 | 107-258 |
3rd to 30th day of life | 4.3-10.6 | 0.7-2.0 | 1.5-3.0 | 78-197 |
1st to 12th month of life | 5.1-10.0 | 1.1-2.3 | 1.1-1.8 | 54-138 |
1 to 7 years of age | 5.2-10.2 | 1.2-2.0 | 0.9-1.7 | 53-123 |
7 to 13 years of age | 6.2-9.5 | 1.1-2.0 | 0.9-1.7 | 60-111 |
13 to 18 years of age | 5.2-8.6 | 1.0-1.8 | 0.9-1.8 | 49-107 |
Adults | 3.4-7.2 | 0.52-2.05 | 0.73-1.95 | 43-111 |
Transport in the blood
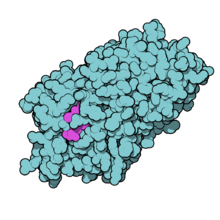
The thyroid hormones are not soluble in water. In the circulating blood they are mostly bound to three proteins: thyroxine-binding globulin (TBG), thyroxine-binding prealbumin (TTR, also transthyretin) and albumin . The binding is reversible for all transport proteins. Although the transport capacity of albumin is much greater, most of the thyroid hormones are transported through the TBG - with thyroxine, for example, this is around 75%. The cause lies in the affinity to the thyroid hormones, which is by far the highest in TBG and slightly higher in TTR than in albumin.
The much smaller but biologically active part of the thyroid hormones is not bound to proteins but circulates freely in the blood. They are called free triiodothyronine (free T 3 , fT 3 ) and free thyroxine (free T 4 , fT 4 ). The free thyroid hormones only make up about 0.3% of all thyroid hormones.
Membrane transport
Since thyroid hormones are charged amino acid derivatives, they cannot - as is often assumed - passively cross the cell membrane, but require specific transport proteins. By regulating the uptake of thyroid hormones in the cell, local availability is also controlled, thereby adding a further level of regulation. About 25 thyroid hormone transporters are known. Mutations in a very specific thyroid hormone transporter, the monocarboxylate transporter 8 (MCT8), cause severe X-linked mental retardation in humans, the Allan-Herndon-Dudley syndrome . The transporters MCT8 and MCT10 also appear to play a major role in passing through the thyroid cell membrane into the bloodstream.
Mode of action
In healthy people, the thyroid hormones serve to maintain a balanced energy balance in the organism. They enable the metabolism to be adapted to the respective needs. In childhood, the hormones stimulate the cells of all organs in the body. They promote growth in this phase of life.
In adulthood they have no influence on the tissues of the brain, testicles and spleen, in all other tissues they increase the metabolism. The biochemical effect in the individual body cells has not yet been fully clarified.
The thyroid hormones also have an effect on the incorporation of α- and β- adrenoceptors and thus affect the function of the heart, but also on muscles, adipose tissue and lymphocytes. The thyroid hormones increase β-receptors , while α1- and α2-receptors are reduced in the cell membranes.
It is important, however, that the thyroid hormones also have an effect on the activity of other endocrine glands. They promote the release of the growth hormone STH through the pituitary gland, intervene in the glucose metabolism by increasing the release of insulin from the pancreas and stimulate the activity of the adrenal gland , especially the adrenal cortex . An interaction with the sex hormones is also known.
Liothyronine (for example, the prescription preparation Thybon ) is sometimes prescribed for the treatment of sub-function in combination with thyroxine, for example, if the patient does not have enough own T 3 is formed from the thyroxine.
Epidemiology of Thyroid Hormones and Pharmacoepidemiology of Thyroid Drugs
Extensive studies by the Robert Koch Institute are available on the epidemiology of thyroid hormones and the pharmacoepidemiology of thyroid drugs in the German population .
Thyroxine in animals
The metamorphosis from tadpole to frog is triggered by thyroxine. If you raise tadpoles in iodine-free water, they won't turn into a frog, but an overly large tadpole. The occurring in Central America salamander Axolotl normally does not go through metamorphosis and achieved while maintaining many of larval features the sexual maturity . Only through a thyroxine treatment does it change into the "landform" that does not occur in nature.
Dogs often suffer from an underactive thyroid . There is a close relationship between aggressive behavior in dogs and an underactive thyroid. This also applies if the thyroxine values measured in the blood are still within the clinical normal range. Similar to humans, the thyroxine can be supplied in tablet form. The dose is often much higher than in humans: 1000 to 4000 µg per day are not uncommon.
Phylogenetics
The thyroid hormones thyroxine (T 4 ) and triiodothyronine (T 3 ) are genetically very old compounds that can already be detected in invertebrate chordates and also in some plants.
literature
- Johannes W. Dietrich: The pituitary-thyroid control circuit. Development and clinical application of a non-linear model. Logos-Verlag, Berlin 2002, ISBN 3-89722-850-5 .
- JW Dietrich, A. Tesche, CR Pickardt, U. Mitzdorf: Thyrotropic Feedback Control: Evidence for an Additional Ultrashort Feedback Loop from Fractal Analysis. In: Cybernetics and Systems. 35 (4), 2004, pp. 315-331. doi: 10.1080 / 01969720490443354
- C. Gauna, GH den Berghe, AJ der Lely: Pituitary Function During Severe and Life-threatening Illnesses. In: Pituitary. 8 (3-4), 2005, pp. 213-217. doi: 10.1007 / s11102-006-6043-3
Web links
Individual evidence
- ↑ thyroid hormone. In: Hoffmann-La Roche AG, Urban & Schwarzenberg (Ed.): Roche Lexicon Medicine. 4th edition. Urban & Schwarzenberg 1998, ISBN 3-541-17114-6 .
- ↑ S. Piehl, CS Hoefig, TS Scanlan, J. Köhrle: Thyronamines - past, present, and future. In: Endocr Rev . 32 (1), Feb 2011, pp. 64-80. PMID 20880963 .
- ↑ a b C. S. Hoefig, J. Köhrle, G. Brabant, K. Dixit, B. Yap, CJ Strasburger, Z. Wu: Evidence for extrathyroidal formation of 3-iodothyronamine in humans as provided by a novel monoclonal antibody-based chemiluminescent serum immunoassay. In: J Clin Endocrinol Metab . 96 (6), Jun 2011, pp. 1864-1872. PMID 21490071 .
- ↑ a b c d e section Physiology in "Thyroid" ( Memento from September 29, 2007 in the Internet Archive ) at DPC-Bühlmann GmbH.
- ↑ a b c d iodine. In: Lexicon for Orthomolecular Medicine - Vital Substance Lexicon.
- ↑ a b c Jörg Sasse: Plasma concentrations of prolactin, cortisol, thriiodothyronine and thyroxine in sleep deprivation responders with tryptophan depletion in the context of endogenous depression . Berlin 2000, DNB 959789901 , section 1.5.3 , urn : nbn: de: kobv: 11-10012126 (dissertation, Humboldt University Berlin).
- ↑ a b c d e f g h i Katarzyna Agata Fischmann: Changes in the thyroid parameters TSH, fT3 and fT4 in the course of detoxification and withdrawal therapy in alcoholics . Tübingen 2005, DNB 97437086X , section 1.1.2 , urn : nbn: de: bsz: 21-opus-16593 (dissertation, Eberhard Karls University of Tübingen).
- ↑ a b Christoph Kraft: The influence of triiodothyronine on the extracellular matrix of rat hearts and rat kidneys. Inaugural dissertation. Department of Human Medicine at the Justus Liebig University Giessen, 2001, Section 1.1.1.
- ↑ a b Thyroxine 5-deiodinase (EC 1.97.1.11) (English) at ExPASy.
- ↑ ATC-H03BX01 entry (English) at ATC / DDD Index 2007 .
- ↑ A. Fonyó (Ed.): Principles of Medical Physiology. 1st edition. Medicina Publishing House Co., 2002, ISBN 963-242-726-2 , pp. 765 ff.
- ^ L. Lacroix: Expression of the apical iodide transporter in human thyroid tissues: a comparison study with other iodide transporters. In: J Clin Endocrinol Metab. 89 (3), Mar 2004, pp. 1423-1428. PMID 15001644 .
- ↑ G. Löffler, PE Petrides (Ed.): Biochemistry and Pathobiochemistry. 7th edition. Springer Medizin Verlag, Heidelberg 2003, ISBN 3-540-42295-1 , pp. 871 ff.
- ↑ B. Caillou et al .: Expression of reduced nicotinamide adenine dinucleotide phosphate oxidase (ThoX, LNOX, Duox) genes and proteins in human thyroid tissues. In: J Clin Endocrinol Metab. 86 (7), Jul 2001, pp. 3351-3358. PMID 11443211 .
- ↑ Entry on Thyroid Hormones. In: Römpp Online . Georg Thieme Verlag, accessed on June 16, 2014.
- ↑ JW Dietrich: The pituitary-thyroid control circuit. Development and clinical application of a non-linear model. Logos-Verlag, Berlin 2002, ISBN 3-89722-850-5 .
- ↑ JW Dietrich, A. Tesche, CR Pickardt, U. Mitzdorf: Thyrotropic Feedback Control: Evidence for an Additional Ultrashort Feedback Loop from Fractal Analysis. In: Cybernetics and Systems. 35 (4), 2004, pp. 315-331. doi: 10.1080 / 01969720490443354 .
- ↑ C. Gauna, GH den Berghe, AJ der Lely: Pituitary Function During Severe and Life-threatening Illnesses. In: Pituitary. 8 (3-4), 2005, pp. 213-217. doi: 10.1007 / s11102-006-6043-3 .
- ↑ laboratory lexicon - journal for laboratory medicine: T 3 , free . ISSN 1860-966X ( online version : accessed February 16, 2008).
- ↑ Laborlexikon - trade journal for laboratory medicine: T 3 , total . ISSN 1860-966X ( online version : accessed February 16, 2008).
- ↑ laboratory lexicon - journal for laboratory medicine: T 4 , free . ISSN 1860-966X ( online version : accessed February 16, 2008).
- ↑ Laborlexikon - trade journal for laboratory medicine: T 4 , total . ISSN 1860-966X ( online version : accessed February 16, 2008).
- ↑ PDB 2CEO
- ↑ a b Siegenthaler (Ed.): Clinical Pathophysiology. 9th edition. Georg Thieme Verlag, Stuttgart 2006, ISBN 3-13-449609-7 , p. 271 ff.
- ↑ JW Dietrich, K. Brisseau, BO Boehm: Resorption, transport and bioavailability of thyroid hormones. In: Dtsch Med Wochenschr. 133 (31-32), Aug 2008, pp. 1644-1648. PMID 18651367 .
- ↑ D. Braun, EK Wirth, U. Schweizer: Thyroid hormone transporters in the brain. In: Reviews in the neurosciences. Volume 21, Number 3, 2010, pp. 173-186, ISSN 0334-1763 . PMID 20879691 .
- ↑ Edith CH Friesema, Jurgen Jansen, Jan-willem Jachtenberg, W. Edward Visser, Monique HA Kester, Theo J. Visser. Effective Cellular Uptake and Efflux of Thyroid Hormone by Human Monocarboxylate Transporter. In: Molecular endocrinology. 2008. doi: 10.1210 / me.2007-0112 .
- ↑ K. Brix, D. Führer, H. Biebermann: Molecules important for thyroid hormone synthesis and action - known facts and future perspectives. In: Thyroid Res. 4 Suppl 1, Aug 3, 2011, p. S9. doi: 10.1186 / 1756-6614-4-S1-S9
- ^ Hans-Christian Pape, Armin Kurtz, Stefan Silbernagl: Physiology . 7th edition. Georg Thieme Verlag, Stuttgart 2014, ISBN 978-3-13-796007-2 , p. 624 .
- ↑ Hans-Ulrich Melchert, Bernd Görsch, Wulf Thierfelder: Thyroid hormones and thyroid medication in test subjects in the national health surveys . Robert Koch Institute, Berlin 2002, ISBN 3-89606-138-0 .
- ↑ Ulrich Welsch: Textbook Histology . 4th edition. Urban & Fischer at Elsevier, Munich 2014, ISBN 978-3-437-44433-3 , p. 437 .