Globular clusters

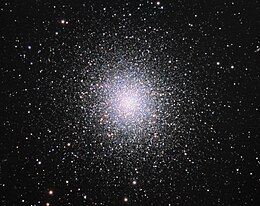
A globular clusters (short and globular clusters ) is a tight collection of many gravitationally together bound stars, where the density of stars is a spherically symmetric shows distribution by decreases from the center, where the stars very together stand close to the edge in all directions equally. Typical globular clusters contain several hundred thousand stars. The high star density near the center leads to mutual changes in orbit , which results in the spherical appearance.
Globular clusters, for their part, are gravitationally bound to galaxies in whose halo they move widely. They mainly consist of old, red stars that contain only a few heavy elements (“ metal poverty ”). This clearly distinguishes them from open star clusters , which are among the most recent formations in galaxies.
Globular clusters are common. In Halo of the Milky Way we are about 150 known, and it is estimated that some are more still undiscovered. There are around 500 globular clusters in the halo of the Andromeda Galaxy. The halos of huge elliptical galaxies like M87 can contain as much as 10,000. These globular clusters orbit the galaxy at a distance of 40 kilo parsec (around 131,000 light years ) or more.
In the local group , all larger, massive galaxies have a halo system of globular clusters. The Sagittarius Dwarf Galaxy and the Canis Major Dwarf Galaxy appear to be surrendering their globular clusters (such as Palomar 12 ) to the Milky Way. This shows how galaxies may have received their globular clusters.
The stars of such clusters - so-called extreme Population II stars - are all roughly the same age and show no spectral lines from heavier elements. These spectra indicate a high stellar age, since the heavy elements only became apparent in the course of billions of years. B. formed by supernovae . Old stars that were formed in the early universe can therefore hardly contain such elements in their shells. Young stars, especially Population I stars , on the other hand, are "recycled": they were formed from material (including heavy elements) that e.g. Some of it was already formed in older stars by nuclear fusion (see also section metal deposits ).
Although the stars in globular clusters were among the first to form in galaxies, their origins and role in galactic evolution are still unclear. It is now assumed that globular clusters differ significantly from elliptical dwarf galaxies and have formed more as part of a galaxy than as a single, separate galaxy.
Very young globular clusters can also be observed in the halo of some elliptical galaxies. These galaxies are believed to have arisen from the merging of two or more original galaxies. Such collisions trigger a wave of star formation (starburst) in which, according to the latest findings, globular clusters can also be formed again, so that several generations of globular clusters can be found in the halo of such a galaxy.
Observation history
The first globular cluster, M22 , was discovered in 1665 by the German amateur astronomer Johann Abraham Ihle . The resolution of the telescopes of his time was so low that only a diffuse, round spot could be seen and no individual stars in the cluster.
Nicolas Louis de Lacaille mentioned several such objects in his catalog published from 1751–1752, especially the objects later named NGC 104 , NGC 4833 , M55 , M69 and NGC 6397 . The M in front of a number stands for the catalog by Charles Messier published in its final form in 1781 , while NGC refers to the New General Catalog by Johan Dreyer (1880). The first globular cluster in which single stars could be observed was cataloged by Messier in 1764 as M4 . M4 is the closest globular cluster.
William Herschel began a new survey in 1782. With more powerful telescopes he was able to detect single stars in all 33 globular clusters known at the time and found 37 other star clusters. In his second catalog of deep sky objects , which appeared in 1789, he first used the term globular cluster to describe it .
The number of globular clusters discovered increased steadily, from 83 in 1915 to 93 in 1930 and 97 in 1947. Today 151 globular clusters are known in the halo of the Milky Way and another 10 to 50 are suspected to be behind the gas and dust of the Milky Way. Most globular clusters can be seen in the southern sky .
In 1914, Harlow Shapley began studies on globular clusters, which he published in 40 papers. He examined the Cepheids , variable stars of a certain type, in the star clusters and used their periodic fluctuations in brightness to determine distance.
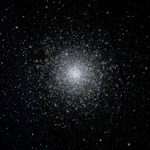
Most of the Milky Way's globular clusters are located near the galactic bulge . In 1918, Harlow Shapley took advantage of the highly asymmetrical distribution to determine the extent of the Milky Way. He assumed an approximately evenly spherical distribution of the globular clusters around the galactic bulge and used the position of the star clusters to determine the position of the sun relative to the galactic center .
Shapley found that the Sun is very far from the center of the Milky Way, and concluded that the galaxy was much larger than previously thought. His estimate is in the same order of magnitude as the value accepted today.
This contradicted the then common model of the universe, since one perceives roughly the same number of stars in each direction in the night sky. It is now known that there is still a lot of gas and dust between the stars that make up the galactic disk , which absorbs most of the light from the galactic center. The globular clusters, on the other hand, are located outside of the galactic disk in the galactic halo , so that they can also be seen from greater distances. With the assumption of an approximately uniform distribution over the galactic disk, the true position and extent of the Milky Way became roughly recognizable for the first time.
Henrietta Hill Swope and Helen Hogg also studied star clusters. From 1927 to 1929, Shapley and Sawyer began to categorize the star clusters according to the concentration of the stars in the center of the star cluster. The star clusters with the greatest concentration were assigned to class I. With decreasing concentration, eleven more classes were formed up to class XII. These classes became known internationally as the Shapley-Sawyer Concentration Classes . Sometimes Arabic numbers are used instead of Roman numerals.
composition
Globular clusters generally consist of hundreds of thousands of metal-poor stars. Such stars can also be found in the bulge of spiral galaxies, but not in this amount in a volume of a few cubic parsecs. In addition, globular clusters do not contain gas or dust, as stars have already formed from them.
Although globular clusters can contain many stars, they are not a suitable place for a planetary system. The orbits of the planets are unstable because passing stars disrupt the orbit. A planet orbiting a star within one astronomical unit would only survive on average about 100 million years in a globular cluster like 47 Tucanae . However, a planetary system has been found ( PSR B1620-26 b ) that orbits the pulsar ( PSR B 1620-26 ), which belongs to the globular cluster M4 .
With a few exceptions, each globular cluster can be assigned an exact age. Since most of the stars in the cluster are all in the same phase of star evolution , it is reasonable to assume that they formed at the same time. Stars are not formed in any known globular cluster. As a result, globular clusters are the oldest objects in the Milky Way that were formed when the first stars formed.
Some globular clusters such as Omega Centauri in the halo of the Milky Way and Mayall II in the halo of the Andromeda Galaxy (M31) are particularly heavy with many millions of solar masses and contain several star populations. Both are believed to have been the nuclei of dwarf galaxies and were captured by a larger galaxy. It is believed that many globular clusters with heavy nuclei (such as M15 ) contain black holes .
Metal deposits
Globular clusters usually consist of Population II stars that contain little metal compared to Population I stars like the Sun. In astrophysics , the term metal includes all elements that are heavier than helium , such as lithium and carbon , see metallicity .
The Dutch astronomer Pieter Oosterhoff noticed that there was a second population of globular clusters, which was named Oosterhoff group. In this group, the periodicity of RR Lyrae stars is longer. Both groups contain only faint lines of metallic elements, but the stars of the Oosterhoff type I star clusters (OoI) are not as heavy as those in type II (OoII). Type I is called "metal-rich", while type II is called "metal-poor". In the Milky Way , the metal-poor star clusters are found in the outer halo and the metal-rich ones near the bulge .
These two populations have been observed in many galaxies (especially massive elliptical galaxies ). Both groups are roughly the same age (roughly the same as the universe itself), but differ in their metal deposits. Many scenarios have been proposed to explain the existence of the two different species, including the merger of galaxies with high gas deposits, the accumulation of dwarf galaxies, and the existence of multiple phases of star formation in a galaxy.
Since the metal-poor star clusters of the Milky Way are located in the outer halo, it seems reasonable to assume that these Type II star clusters were captured by the Milky Way and are not the oldest objects that were formed in the Milky Way, as previously assumed. The differences between the two types of globular clusters would then be explained by a time difference in their formation.
Unusual stars
Globular clusters have a very high star density, which leads to greater mutual influence and relatively frequent near-collisions between the stars. As a result, exotic stars such as blue stragglers , millisecond pulsars and light X-ray binary stars are much more common. A blue straggler arises from two stars, possibly from the collision of a binary star system. The resulting star has a higher temperature than comparable stars in the star cluster with the same brightness and is therefore outside of the main sequence stars .
Black holes
Astronomers have been looking for black holes in globular clusters since the 1970s . This requires an accuracy that is currently only possible with the Hubble space telescope . A medium-weight black hole of 4,000 solar masses in the globular star cluster M15 (constellation Pegasus) and a 20,000 solar mass black hole in the globular cluster Mayall II in the halo of the Andromeda Galaxy were discovered in independent programs . These are of interest because they were the first black holes to be intermediate in size between a conventional star-formed black hole and the supermassive black holes that exist in centers of galaxies such as the Milky Way. The mass of these medium-weight black holes is proportional to the mass of the star cluster, whereby these are in the same mass ratio as the supermassive black holes with their surrounding galaxies. The discovery of medium-weight black holes in globular clusters is, however, controversial and the observations can also be explained without the assumption of a central black hole.
Black holes can be in the center of globular clusters (see M15 above), but they do not necessarily have to be present. The densest objects migrate to the center of the cluster due to the mass separation . In old globular clusters, these are mainly white dwarfs and neutron stars . In two scientific works under the direction of Holger Baumgart it was shown that the mass-light ratio can increase significantly even without black holes in the center. This applies to both M15 and Mayall II.
In the summer of 2012, radio telescopes were used to discover that Messier 22 in the Sagittarius constellation even contains two black holes, which was previously considered impossible for reasons of celestial mechanics . The two radio sources each have 10–20 solar masses.
Hertzsprung-Russell diagram
The Hertzsprung-Russell diagram (HR diagram) is a graph that shows the stars with their absolute brightness and color. The color index shows the difference between the brightness of the star in blue light (B) and the brightness in yellow to green light (V). Large positive values indicate a red star with a cold surface temperature , while negative values indicate a blue star with a hot surface.
If you enter stars from the area around the sun in the diagram , then many of them lie on this diagram in a curved curve, the so-called main series . The diagram also includes stars in the later stages of their evolution that have moved slightly away from the main sequence.
Since all stars in a globular cluster are approximately the same distance from Earth, their absolute brightness differs from the visible or apparent brightness by the same amount. It is estimated that the main sequence stars in the globular cluster in the diagram are on the same curve as the stars in the vicinity of the sun. The accuracy of this estimate was confirmed by comparing the brightness of neighboring rapidly changing stars, such as RR Lyrae stars and the Cepheids , with those in the star cluster.
Since these curves agree in the HR diagram, the absolute brightness of the main sequence stars in the star cluster can be determined. With the help of the apparent brightness of the stars, one can get the distance of the star cluster from the earth. This distance is determined from the difference between the apparent and absolute brightness, the distance module .
When the stars of a globular cluster are plotted on an HR diagram, most of them are on a well-definable curve. This differs from stars in the vicinity of the sun, since stars of different origins and ages were not collected here. The shape of the curve is characteristic of a group of stars that formed around the same time with the same material and differ only in their mass. Since the positions of the stars from globular clusters in the HR diagram only differ in terms of their age, one can deduce their age from this.

The heaviest stars in the globular cluster are also the brightest and the first to become giant stars . Later stars with lower masses will also turn into giants. You can also determine the age of a globular cluster by looking for stars that have already reached the level of giant stars. These form a "bend" in the HR diagram and connect the lower right end with the line of the main series. The age of the globular cluster can be read directly from the absolute brightness of this “bend”, so that an axis for the age of globular clusters could be drawn in parallel to the brightness axis in an HR diagram. However, one might as well determine the age by examining the temperature of the coldest white dwarfs in this globular cluster.
The typical age for globular clusters is 12.7 billion years. In comparison, open star clusters are much younger, only ten million years old.
The age of globular clusters sets limits to the age of the entire universe. The lower limit embarrassed cosmology . During the early 1990s, astronomers found globular clusters that appeared to be older than the cosmological model would allow. However, better measurements of the cosmological parameters, such as the COBE satellite, showed that the earlier measurements were incorrect.
By studying the occurrence of metals (in astronomy metals are elements that are heavier than helium ), one can determine the concentration of the original substances and then transfer these values to the entire Milky Way.
Recent studies with space-based satellites and telescopes of the 8-meter class have shown that all globular clusters examined in detail do not consist of a chemically homogeneous population. Variations in the abundance of elements such as carbon, oxygen, nitrogen, sodium and aluminum have been detected spectroscopically in various globular clusters, and the existence of several main sequences has been detected photometrically . A particular example is Omega Centauri , in which three separate main rows and five distinguishable red giant branches could be detected. Therefore, when globular clusters were formed, several phases of star formation occurred.
shape
In contrast to open star clusters , most of the stars in globular clusters are gravitationally bound throughout their life. One exception is strong interactions with other massive objects. This leads to the scattering of the stars.
The formation of globular clusters is a poorly understood phenomenon. Through observations of globular clusters it could be shown that they formed in areas in which strong star formation was in progress and where the interstellar medium possessed a greater density than in average star formation areas. Globular clusters usually form in star formation regions and in interacting galaxies .
After the stars have formed, they begin to gravitationally influence each other. As a result, the magnitude and direction of the speed of each star are constantly changing, so that after a short time no conclusions can be drawn about its original speed. This characteristic interval is called the relaxation time . It depends on the length of time it takes a star to traverse the cluster and the number of stars in the system. The relaxation time varies from star cluster to star cluster, but averages one billion years.
Although globular clusters usually have a spherical shape, elliptical shapes are also possible due to the effects of tides .
Radii
Astronomers characterize the shape of a globular cluster by standard radii. These are the core radius (r c ), the half-light radius (r h ) and the tidal radius (r t ). The overall brightness decreases with increasing distance from the core, and the core radius is the distance at which the surface brightness has fallen to half. The half-light radius represents a comparable quantity, which marks the distance from the center up to which half of the total light is captured. This value is usually larger than the core radius.
It should be noted that the half-light radius includes stars that are in the outer part of the cluster when they are on the line of sight through the core, so that theorists still need the half-mass radius (r m ). This radius indicates the size of the area that contains half the mass of the star cluster. If the semi-mass radius is very small compared to the total size, then it has a dense core. For example, the globular cluster M3 has a visible extension of 18 arc minutes , but only a half-mass radius of 1.12 arc minutes.
The tidal radius indicates the distance from the center of the core at which the gravitational influence of the galaxy is greater than that of the other stars in the star cluster. This is the distance at which individual stars can escape the globular cluster. The tidal radius of M3 is around 38 '.
Star concentration
In the center of typical globular clusters there is an estimated star concentration of 1000 to 10,000 stars per cubic parsec (for comparison: the star density in the vicinity of our sun is 0.14 stars / pc³). That means an average distance between the individual stars in the order of 0.1 light years , which is only about 7 times the maximum solar distance of the minor planet Sedna . If the solar system were in the center of a globular cluster (where it could not exist permanently), our sky would be littered with brilliantly bright stars. Collisions are not uncommon, and mutual path influences are inevitable.
brightness
If the brightness of a globular cluster is given as a function of the radius, the brightness of most globular clusters increases with increasing distance from the nucleus, but decreases again after a certain point. This is usually one or two parsecs away from the core. However, 20% of the globular clusters have experienced the process of core collapse. With them, the luminosity increases steadily towards the center. An example of such a core collapse can be found in the globular cluster M15 .
It is believed that core collapse occurs when heavy stars collide with less heavy companion stars in a globular cluster. As a result, they lose kinetic energy and begin to move towards the core. Over a longer period of time this leads to a mass concentration in the core.
The brightness distribution of the globular clusters in the halo of the Milky Way and in the halo of the Andromeda Galaxy (M31) can be imagined as a Gaussian curve . A Gaussian curve can be characterized with the help of two items, the average brightness M v and the variance σ 2 . This distribution of brightness in a globular cluster is called the Globular Cluster Luminosity Function (GCLF). The GCLF is also used as a standard candle to determine the distance to other galaxies. One assumes, however, that the globular clusters in the halo of the observed galaxy behave exactly like those in the halo of the Milky Way.
N-body simulations
In order to calculate the movements of the stars in the globular cluster, one investigates the interactions between the stars in the globular cluster. Since each of the N stars in the star cluster interacts with N-1 stars at the same time, one is dealing with an N-body problem . With simple computer algorithms, the time required would be proportional to N 2 , so that an accurate simulation can take up a lot of computing time. In order to simulate the stars to save time, they can be dynamically combined into groups of stars with similar positions and speeds. The movements are then described with the Fokker-Planck equation . This can be solved as an equation or calculated using the Monte Carlo simulation . However, the simulation becomes more difficult once you add the effects of double stars and the gravitational forces of the Milky Way to the model.
The results of the N-body simulation show that the stars can make unusual movements through the star cluster. They loop or fall straight into the core instead of circling it. Because of the interactions with other stars, individual stars can get enough speed to escape the star cluster. Over a longer period of time this leads to dissolution. The dissolution usually takes place over a period of 10 10 years.
With up to 50% of the stars, binary star systems make up a considerable proportion of a globular cluster. Simulations have shown that binary star systems can stop and even reverse the process of core collapse. When a binary star interacts with a single star, the binary stars become more tightly bound and transfer kinetic energy to the single star. When this process speeds up the massive stars in the star cluster, the core continues to expand and does not collapse as easily.
Intermediate forms
There is no clear dividing line between the two types of star clusters, the globular clusters and the open star clusters . For example, in the southern part of the halo of the Milky Way galaxy is the star cluster BH 176, which is both.
In 2005, astronomers found an entirely new type of star cluster in the halo of the Andromeda Galaxy. These objects resemble globular clusters in terms of number of stars, age and metallicity. The difference, however, lies in the much larger extent of many hundreds of light years, so that these extended star clusters have a much lower density. Their size lies between the globular star clusters and the spherical dwarf galaxies .
How these star clusters formed is not known. However, they could have formed in a similar way to the globular clusters. It is also unknown why the Andromeda Galaxy has such a star cluster while there does not seem to be such an object in the halo of the Milky Way, and whether there are other galaxies with these extended star clusters.
Axis of rotation, rotation
In many globular clusters there is a rotation signal in the center. This means that the cluster has an axis of rotation around which more stars orbit in one direction than in the other. This is shown by studies with the VIRUS-W instrument. In addition, the rotation is very closely related to a relatively weak flattening of the globular clusters.
Tidal effect
When a globular cluster comes near a very heavy object, like the core region of a galaxy, gravitational forces act on it. The difference between the gravitational force on the location of the star cluster that comes closest to the heavy object and the location that is furthest away from it is called the tidal force. If the object crosses the plane of a galaxy, one can speak of a "tidal surge".
The tidal surge causes many of the stars in the cluster, which happen to have a lot of kinetic energy, to be torn out, so that the cluster then pulls a stream of stars behind it. In the case of small clusters, this can contain a large part of the original stars in the cluster, and clumping can occur in these streams.
For example, the small globular cluster Palomar 5 was torn apart when it crossed the galactic disk of the Milky Way. It is now near the apogalactic point of its orbit and has been stretched to a length of 13,000 light years. The tidal forces have hurled many stars away from Palomar 5 and it is expected that further traverses of the galactic disk of the Milky Way will transform it into a single stream of stars that will then travel through the halo of the Milky Way.
Tidal effects give additional kinetic energy to many of the remaining stars in the cluster, heating the cluster and dramatically increasing the rate of dissolution. A tidal shock also leads to the faster core collapse.
See also
Web links
- What are globular clusters? from the alpha-Centauri television series(approx. 15 minutes). First broadcast on Mar 3. 2002.
- astronews.com: Black holes in globular clusters September 18, 2002
- astronews.com: Relics of star formation in the young universe? April 6, 2018
- Determination of the distance to globular clusters , high school diploma thesis
- Very good and understandable representation of globular clusters
- ESO: VISTA discovers 96 star clusters hidden behind dense dust August 3, 2011
- ESO: VISTA discovers new globular clusters + photos & animations October 19, 2011
- ESO: VLT discovers new type of globular cluster May 13, 2015
Individual evidence
- ^ Hubble Images a Swarm of Ancient Stars (07/01/1999). In: hubblesite.org. Retrieved January 30, 2015 .
- ↑ Hartmut Frommert (Ed.): Milky Way Globular Clusters. 2007, accessed September 1, 2008 .
- ↑ a b Ashman, Keith M .; Zepf, Stephen E .: The formation of globular clusters in merging and interacting galaxies . In: Astrophysical Journal , Part 1 . 384, 1992, pp. 50-61. bibcode : 1992ApJ ... 384 ... 50A .
- ↑ P. Barmby & JP Huchra : M31 Globular Clusters in the Hubble Space Telescope Archive. I. Cluster detection and completion . In: The Astrophysical Journal . 122, 2001, pp. 2458-2468.
- ↑ Swiss: The halo globular clusters of the giant elliptical galaxy Messier 87 . In: Astrophysical Journal . 245, No. 5457, 1981, pp. 416-453. bibcode : 1981ApJ ... 245..416S .
- ↑ B. Dauphole, M. Geffert, J. Colin, C. Ducourant, M. Odenkirchen, H.-J. Tucholke: The kinematics of globular clusters, apocentric distances and a halo metallicity gradient. . In: Astronomy and Astrophysics . 313, 1996, pp. 119-128. bibcode : 1996A & A ... 313..119D .
- ^ William E. Harris: Globular cluster systems in galaxies beyond the Local Group . In: Annual Review of Astronomy and Astrophysics . 29, 1991, pp. 543-579. bibcode : 1991ARA & A..29..543H .
- ↑ DI Dinescu, SR Majewski, TM Girard, KM Cudworth: The Absolute Proper Motion of Palomar 12: A Case for Tidal Capture from the Sagittarius Dwarf Spheroidal Galaxy . In: The Astronomical Journal . 120, No. 4, 2000, pp. 1892-1905. bibcode : 2000astro.ph..6314D .
- ^ National Optical Astronomy Observatory: M22. In: noao.edu. Retrieved January 30, 2015 .
- ↑ Harlow Shapley : Globular Clusters and the Structure of the Galactic System . In: Publications of the Astronomical Society of the Pacific . 30, No. 173, 1918, p. 42 ff .. bibcode : 1918PASP ... 30 ... 42S .
- ^ Helen Battles Sawyer Hogg, Harlow Shapley and Globular Clusters . In: Publications of the Astronomical Society of the Pacific . 77, 1965, pp. 336-346.
- ^ Steinn Sigurdsson: Planets in globular clusters? . In: Astrophysical Journal . 399, No. 1, 1992, pp. L95-L97. bibcode : 1992ApJ ... 399L..95S .
- ↑ Z. Arzoumanian, K. Joshi, FA Rasio, SE Thorsett: Orbital Parameters of the PSR B1620-26 Triple System . In: Proceedings of the 160th colloquium of the International Astronomical Union . 105, 1999, p. 525. bibcode : 1996astro.ph..5141A .
- ^ Roeland van der Marel: Black Holes in Globular Clusters. (No longer available online.) Space Telescope Science Institute, March 16, 2002, archived from the original on May 25, 2012 ; Retrieved June 8, 2006 .
- ^ A b T. S. van Albada, Norman Baker: On the Two Oosterhoff Groups of Globular Clusters . In: Astrophysical Journal . 185, 1973, pp. 477-498.
- ^ WE Harris: Spatial structure of the globular cluster system and the distance to the galactic center . In: Astronomical Journal . 81, 1976, pp. 1095-1116. bibcode : 1976AJ ..... 81.1095H .
- ^ YW Lee, SJ Yoon: On the Construction of the Heavens . In: An Aligned Stream of Low-Metallicity Clusters in the Halo of the Milky Way . 297, 2002, p. 578. bibcode : 2002astro.ph..7607Y .
- ^ A b V. C. Rubin, WKJ Ford: A Thousand Blazing Suns: The Inner Life of Globular Clusters . In: Mercury . 28, 1999, p. 26. Retrieved June 2, 2006.
- ↑ Hubble Discovers Black Holes in Unexpected Places (09/17/2002). In: hubblesite.org. September 17, 2002, accessed January 30, 2015 .
- ^ Matthew J. Benacquista, Jonathan M. B. Downing: Relativistic Binaries in Globular Clusters . In: Astrophysics. Solar and Stellar Astrophysics . 2011, arxiv : 1110.4423v1 .
- ↑ Baumgardt, Holger; Hat, piet; Makino, Junichiro; McMillan, Steve; Portegies Zwart, Simon: On the Central Structure of M15 . In: Astrophysical Journal Letters . 582, 2003, p. 21. bibcode : 2003ApJ ... 582L..21B .
- ↑ Baumgardt, Holger; Hat, piet; Makino, Junichiro; McMillan, Steve; Portegies Zwart, Simon: A Dynamical Model for the Globular Cluster G1 . In: Astrophysical Journal Letters . 589, 2003, p. 25. Retrieved September 13, 2006.
- ↑ Nature Sept. 2012, p. a. Wiener Zeitung October 4, 2012
- ↑ Shapley, H .: Studies based on the colors and magnitudes in stellar clusters. I, II, III. . In: Astrophysical Journal . 45, 1917, pp. 118-141. bibcode : 1917ApJ .... 45..118S .
- ↑ Martin Schwarzschild: Structure and Evolution of Stars . Princeton University Press, 1958.
- ^ Sandage, AR : Observational Approach to Evolution. III. Semiempirical Evolution Tracks for M67 and M3. . In: Astrophysical Journal . 126, 1957, p. 326. bibcode : 1957ApJ ... 126..326S .
- ↑ BMS Hansen, J. Brewer, GG Fahlman, BK Gibson, R. Ibata, M. Limongi, RM Rich, HB Richer, MM Shara, PB Stetson: The White Dwarf Cooling Sequence of the Globular Cluster Messier 4 . In: Astrophysical Journal Letters . 574, 2002, p. L155. arxiv : astro-ph / 0205087 .
- ^ Ashes from the Elder Brethren - UVES Observes Stellar Abundance Anomalies in Globular Clusters . March 1, 2001
- ^ Sarah L. Martell: Light-element abundance variations in globular clusters . In: Astrophysics. Solar and Stellar Astrophysics . 2011, arxiv : 1105.5010 .
- ↑ BG Elmegreen, YN Efremov: A Universal Formation Mechanism for Open and Globular Clusters in Turbulent gas . In: Astrophysical Journal . 480, No. 2, 1999, p. 235. bibcode : 1997ApJ ... 480..235E .
- ^ A b Matthew J. Benacquista: Globular cluster structure . In: Living Reviews in Relativity . 2006. Retrieved August 14, 2006.
- ^ R. Buonanno, CE Corsi, A. Buzzoni, C. Cacciari, FR Ferraro, F. Fusi Pecci: The Stellar Population of the Globular Cluster M 3. I. Photographic Photometry of 10,000 Stars . In: Astronomy and Astrophysics . 290, 1994, pp. 69-103. bibcode : 1994A & A ... 290 ... 69B .
- ↑ Globular cluster, part III , University of Heidelberg 2015, accessed on May 2, 2020
- ↑ Astrophysics II , University of Munich 2017/18, accessed on May 2, 2020
- ↑ S. Djorgovski, IR King: A preliminary survey of collapsed cores in globular clusters . In: Astrophysical Journal . 305, 1986, pp. L61-L65. bibcode : 1986ApJ ... 305L..61D .
- ↑ Heggie, DC; M. Giersz, R. Spurzem, K. Takahashi (1998). Johannes Andersen "Dynamical Simulations: Methods and Comparisons". Highlights of Astronomy Vol. 11A, as presented at the Joint Discussion 14 of the XXIIIrd General Assembly of the IAU, 1997 , 591+, Kluwer Academic Publishers. (from May 28, 2006) bibcode : 1997astro.ph.11191H
- ^ Matthew J. Benacquista: Relativistic Binaries in Globular Clusters . In: Living Reviews in Relativity . No. Irr-2006-2, 2006. Retrieved May 28, 2006.
- ^ J. Goodman and P. Hut (eds.): Dynamics of Star Clusters (International Astronomical Union Symposia) . 1st edition. Springer, Netherland 1985, ISBN 90-277-1963-2 .
- ↑ S. Ortolani, E. Bica, B. Barbuy: BH 176 and AM-2: globular or open clusters? . In: Astronomy and Astrophysics . 300, 1995, p. 726. bibcode : 1995A & A ... 300..726O .
- ↑ a b A.P. Huxor, NR Tanvir, MJ Irwin, R. Ibata: A new population of extended, luminous, star clusters in the halo of M31 . In: Monthly Notices of the Royal Astronomical Society . 360, 2005, pp. 993-1006. arxiv : astro-ph / 0412223 .
- ↑ http://www.mpe.mpg.de/5879328/News_20140507 from May 7, 2014
- ^ P. Di Matteo, P. Miocchi, R. Capuzzo Dolcetta: Formation and Evolution of Clumpy Tidal Tails in Globular Clusters . American Astronomical Society, DDA meeting # 35, # 03.03, June 2, 2006 bibcode : 2004DDA .... 35.0303D
- ↑ Jakob Staude: Sky Survey Unveils Star Cluster Shredded By The Milky Way. In: Image of the Week. Sloan Digital Sky Survey, June 3, 2002, accessed June 2, 2006 .