Black hole

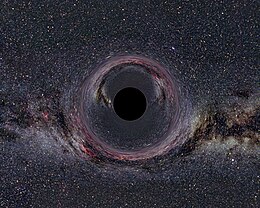
A black hole is an object whose mass is concentrated in an extremely small volume and, as a result of this compactness, generates such strong gravity in its immediate vicinity that even light can not leave or pass through this area. The outer limit of this area is called the event horizon . Nothing can cross an event horizon from the inside out - no information, no radiation, and certainly no matter. The general theory of relativity conclusively describes the fact that a “way out” is no longer even conceivable through an extreme curvature of spacetime .
There are different classes of black holes with their respective mechanisms of formation. The easiest to understand are stellar black holes, which are formed when a star of a certain size has used up all of its “fuel” and collapses. While the outer shells are then repelled in a supernova , the gravity of the core collapses into an extremely compact body. For a hypothetical black hole with the mass of the Sun, the event horizon would have a diameter of only about six kilometers, which corresponds to the 230,000th part of the current solar diameter. At the other end of the spectrum, there are supermassive black holes of billions to billions of times the mass of the Sun, which are at the center of galaxies and play an important role in their development.
Outside the event horizon, a black hole behaves like a normal mass body and can be orbited by other celestial bodies on stable orbits. The event horizon appears visually from the outside as a completely black and opaque object, in the vicinity of which the space behind is depicted as distorted by an optical lens .
The name Black Hole was coined in 1967 by John Archibald Wheeler . At that time, the existence of the theoretically described black holes was considered very likely, but was not yet confirmed by observations. Numerous examples of black hole effects were later observed, e.g. B. from 1992 the investigations of the supermassive black hole Sagittarius A * in the center of the Milky Way in the infrared range. 2016, the fusion of two black was holes on the thus generated gravitational by AGS observed and 2019. achieved a radioteleskopische taking a picture of super black hole M87 * in the center of the galaxy M87 .
In 2020, the scientists Roger Penrose , Reinhard Genzel and Andrea Ghez were awarded the Nobel Prize in Physics for their research on black holes .
Research history
18th century
As early as 1783, the British naturalist John Michell speculated about dark stars, whose gravity is sufficient to hold light captive. In a letter published by the Royal Society, he wrote:
“If the semi-diameter of a sphere of the same density as the Sun were to exceed that of the Sun in the proportion of 500 to 1, a body falling from an infinite height towards it would have acquired at its surface greater velocity than that of light, and consequently supposing light to be attracted by the same force in proportion to its vis inertiae [mass], with other bodies, all light emitted from such a body would be made to return towards it by its own proper gravity. This assumes that light is influenced by gravity in the same way as massive objects. "
“If the radius of a sphere of the same density as the sun exceeded that of the sun by a ratio of 500 to 1, a body falling towards it from infinite heights would have acquired a higher speed on its surface than that of light. Hence, assuming that light is attracted to the same force in relation to its mass as other bodies, all light given off by such a body would return to it as a result of its own gravitation. This is true under the assumption that light is influenced by gravity in the same way as massive objects. "
The idea of heavy stars, from which corpuscular light cannot escape, was also described by Pierre Simon Laplace in his Exposition du Système du Monde in 1796 . He created the term "dark body" (corps obscur) for this. These ideas moved within Newtonian physics .
First half of the 20th century: Contribution of the general theory of relativity
After Albert Einstein had set up the field equations of the general theory of relativity in 1915 , the German astronomer Karl Schwarzschild first specified a metric in 1916, the Schwarzschild metric , which corresponds to the gravitational field of a point mass. The Schwarzschild solution describes the size and behavior of a non-rotating and non-electrically charged static black hole with the so-called event horizon at and a central singularity at . It stands for the gravitational constant , for the mass of the black hole and for the speed of light .
For example, if the mass of the sun were to be compressed into a sphere with a radius of only three kilometers, no ray of light could escape from its surface. The mass of our earth ( ) would only form a black hole with a radius of less than one centimeter.
With the Kruskal-Szekeres coordinates in the 1950s, it could be shown mathematically that an external observer who sees an internal observer rushing towards the black hole must get the impression that the internal observer is only approaching the event horizon asymptotically , with despite regular transmission of slower and slower incoming signals. In contrast, the internal observer himself quickly crosses the event horizon without feeling anything special, although from now on he can no longer turn back and no longer exchange signals with the external observer and is very soon engulfed by the singularity at .
In the late 1920s, the Indian astrophysicist Subrahmanyan Chandrasekhar showed that a certain limit mass, the so-called Chandrasekhar limit , exists for an astrophysical object without nuclear reactions . Objects above this mass limit collapse into neutron stars or black holes, but not, as expected, into white dwarfs . Chandrasekhar's work sparked controversy with astronomer Arthur Eddington . The former believed that stars above the mass limit could collapse into objects whose gravity could trap electromagnetic rays. Eddington expected, however, that there would be a mechanism that would prevent the collapse. In 1939, Robert Oppenheimer , together with Robert Serber and George Michael Volkoff, used model calculations to show that a black hole would form if a large star collapsed.
Second half of the 20th century: Extended theory formation
In 1963, the mathematician Roy Kerr described a solution for a rotating black hole with the Kerr metric . Until then, the terms black stars or frozen stars were used - the latter as a metaphor for the fact that, according to the theory, time seems to stand still at the edge of the black hole due to the gravitational time dilation from the outside.
The term "black hole" was first identified in 1964 in a report by science journalist Ann Ewing on a symposium of the American Association for the Advancement of Science on the various terminal stages of stars. The author named Hong-Yee Chiu as organizer and Alastair Cameron , Charles Misner , Volker Weidemann and John Beverly Oke as speakers, without naming the originator of the expression. The term was established in 1967 after John Archibald Wheeler was looking for a replacement for the long term “gravitationally completely collapsed object” at a conference and took up the suggestion of an unknown listener.
The first observable candidate for a black hole followed in 1971 with the discovery of Cygnus X-1 . In the early 1970s , Jacob Bekenstein set up a thermodynamics of black holes in the theoretical field by assigning an entropy to the surface of the event horizon ( Bekenstein-Hawking entropy ). This was supported by the discovery by Stephen Hawking (1974) that black holes emit a radiation called Hawking radiation . At the same time, a connection between general relativity and quantum field theory was made.
Physical description
static | rotating | |
---|---|---|
uncharged | Schwarzschild metric | Kerr metric |
loaded | Reissner-Nordström metric | Kerr-Newman metric |
: Electric charge ; : Angular momentum |
Development dynamics

In general, the mass of a body always results in gravitational forces. If the mass is limited to a sufficiently small volume, the body holds itself together: The gravitational force leads to a compression of the body. Usually there are opposing forces inside that hold back further compression, resulting in a balance between gravity and the opposing forces. Depending on the size of the object, the opposing forces can be thermodynamic pressure , repulsion between atoms or nucleons or Fermi pressure . The last stable mass limit is around 1.5 to 3.2 solar masses ( Tolman-Oppenheimer-Volkoff limit ); In the case of objects that are lighter, the degeneracy pressure in the matter in the degenerate state can successfully counteract a gravitational collapse .
If a critical density is exceeded, the opposing forces are no longer sufficient to compensate for gravity. A gravitational collapse is the result: The gravitational force increases faster than the counterforces resulting from the repulsion of the particles. This accelerates the process itself. The mass collapses to a vanishing volume. The ever increasing gravitation locally distorts the space and the passage of time in such a way that - viewed from a sufficient distance - the collapse slows down, the light rays emitted by the event become less and less energetic, and the volume never focuses on a single one Point together.
Black holes can arise from massive stars at the end of their stellar evolution . Stars in the main sequence above approx. 40 solar masses end up as black holes via the intermediate stages Wolf-Rayet star and supernova . Stars with masses between approx. 8 and approx. 25 solar masses and all massive stars with high metallicity end up as neutron stars . If their mass is between approx. 25 and approx. 40 solar masses, black holes can arise from the relapse of the material that was detonated during the incomplete supernova.
Gravitational effects
Since the mass is retained, the density of the body increases beyond all limits. Such bodies curve space-time around them so strongly that one could clearly speak of a hole in the structure of space, but they are called exact singularity . The singularity is surrounded by a space-time area from which neither matter nor information can get out. The limit of this area is the so-called event horizon , the distance of the event horizon from the singularity is the so-called Schwarzschild radius.
The event horizon is not a physical structure, it only designates a place or, more precisely, an interface. An observer who falls through the event horizon would therefore not notice anything himself. However, relativistic effects ( general theory of relativity ) mean that a body viewed by a second, distant observer, due to the time dilation, takes an infinitely long time to reach the event horizon, whereby it increasingly appears in red-shifted light and becomes weaker.
The gravitational field in the outer space of spherical, non-rotating and electrically uncharged bodies is described by the Schwarzschild metric . It is not only valid for black holes, but for all bodies with these properties and is usually a good approximation for stars or planets due to their low rotational speed. The size of the Schwarzschild radius is about 2.9 kilometers for a black hole of one solar mass, for an object the mass of the earth about 9 millimeters.
It is a widespread misconception that the gravitational field of a black hole or the curvature of space and time caused by it is extraordinarily strong at normal distances. Since both black holes and stars are described by the same metric , nothing would change in the gravitational field in the solar system if the sun were replaced by a black hole of the same mass. Apart from the lack of sunlight, an enormous increase in gravitational acceleration could only be observed in the immediate vicinity of the black hole (within roughly the previous core radius of the sun).
rotation
The rotating black hole is a more general form of this astrophysical phenomenon. Rotating black holes are those that have their own angular momentum. Like all black holes, they cause, due to their enormous gravitation , a correspondingly large change in the geometric structure of space and time (see space-time curvature ). In the case of a rotating black hole, however, the singularity takes on a circular or ring shape and carries away space-time around it instead of just bending it: The space is rotated in the direction of rotation of the black hole. This kind of space-time curvature does not appear with a black hole at rest, but with rotating black holes it also occurs outside the event horizon with the shape of a rotation ellipsoid flattened at the poles . All objects around a rotating black hole are rotated with it, precisely because space-time itself rotates with it.
To an observer who is stationary relative to his surroundings, it would appear as if the whole universe revolved around him. This effect decreases sharply with distance. But up to a certain distance (the so-called static limit ), in an area called the “ ergosphere ”, the speed of rotation is so high that all objects (and also energy such as rays of light) would have to be faster than light in order to reach the speed of rotation to compensate, so not to rotate. The angular speed of a particle at the actual event horizon corresponds exactly to the rotation speed of the black hole. The angular velocity of the particle decreases towards the outside, but its orbital velocity always has a component in the direction of rotation of the black hole. This does not mean that its own speed is greater than the speed of light, but that there cannot be any non-rotating particles within the ergosphere. This frame dragging is an extreme case of the Lense-Thirring-Effect known since 1918 . A special feature of this area is that the kinetic energy in this area can be negative from the perspective of an outside observer. A particle that is in the ergosphere can therefore decay into two particles in such a way that the kinetic energy of one of the two is greater than that of the original particle. The particle in question can leave the ergosphere, while its complement with negative kinetic energy (without further interaction) necessarily and in finite proper time crosses the event horizon. The energy apparently generated out of nowhere is withdrawn from the rotation energy of the black hole. This mechanism for generating energy was first proposed by Roger Penrose .
The extent of the ergosphere depends on the polar angle (corresponds to the complementary angle of the geographic latitude on Earth): At the poles of the rotating black hole, the static boundary coincides with the event horizon, in the equatorial region it extends to a boundary that depends on the angular momentum of the black hole Distance of a maximum of twice the Schwarzschild radius. The angular momentum of a black hole is limited , as described below .
Some observations, such as extremely fast matter jets ( jets ) that the area outside the event horizon perpendicular to the accretion leave be described by effects thereof can only occur within a ergosphere or in the presence. From general considerations on conservation of angular momentum , one can conclude that all black holes rotate, at least at the time of their formation. But of course only very fast rotating black holes show strong effects of the phenomena known as frame dragging. On the other hand, every rotating mass, regardless of the occurrence of an event horizon, including planet earth, twists the surrounding space-time. These effects on earth should be quantified by measurements, for example using the LAGEOS satellites. The first results from 1997 were so close to the range of measurement inaccuracy that they were discussed controversially. Only a repetition of the measurement in 2004 with the Gravity Probe B satellite confirmed the situation.
Theoretical considerations
Mathematical description
A black hole can be fully described by just three physical parameters (so-called hairlessness of black holes): mass, angular momentum and electrical charge . The multipole moments are omitted. So there are the following classes:
- Black holes that carry no electrical charge ( ) and do not rotate ( ) are described by the Schwarzschild metric .
- Black holes that carry no electrical charge ( ) and rotate ( ) are described by the Kerr metric .
- Black holes that are electrically charged ( ) and do not rotate ( ) are described by the Reissner-Nordström metric .
- Black holes that are electrically charged ( ) and rotate ( ) are described by the Kerr-Newman metric .
Black holes in general relativity
Formally, a black hole results from a special vacuum solution of the general theory of relativity , the so-called Schwarzschild solution (after Karl Schwarzschild , who was the first to find this solution), or for rotating and electrically charged black holes from the Kerr-Newman solution . A “vacuum solution” is a solution of the vacuum field equations - for example in the outer space around a star, where there is almost no matter and thus the energy-momentum tensor disappears. Inside, as Stephen Hawking and Roger Penrose have shown ( singularity theorem ), a singularity , a point with an infinitely high curvature of space , forms in the context of the description by the classical general theory of relativity . However, the area of validity of the general theory of relativity has been exceeded and a theory of quantum gravity is necessary to describe this location .
The limit beyond which information can no longer reach an observer who is in infinity is called the event horizon, its radius is the Schwarzschild radius. Since a non-rotating black hole is spherical when viewed from the outside, the event horizon also has the shape of a spherical surface. For a given mass, black holes cannot have an arbitrarily large charge or an arbitrarily large angular momentum. If you insert too high a charge and / or too high an angular momentum into the corresponding solutions of the general theory of relativity, a so-called naked singularity results instead of a black hole : a central singularity is formed, but it is not of one Surrounding event horizon: One can imagine that the rotation of space-time would accelerate the incident matter so strongly ( centrifugal force ) that it cancels the gravitation again. As a result, there would be no event horizon because the matter could escape again. However, it can be shown that no naked singularity can arise from a normal black hole through the addition of charge or angular momentum, because the energy supplied at the same time would increase its mass sufficiently, so that it is always prevented that an ordinary black hole with a naked one is prevented Singularity arises. Roger Penrose called this Cosmic Censorship , but the proof of the non-existence of naked singularities within general relativity is open.
The event horizon is limited by rays of light (the so-called photon sphere ) for stars that have collapsed into non-rotating black holes . These rays of light are the last ones that have not yet been attracted by the gravitation of the black hole. In the case of rotating black holes (see above) there is not only one radius on which light rays can circle the singularity, but an infinite number within the ergosphere. Near the singularity, i.e. well within the Schwarzschild radius, the distortion of spacetime is so strong that for an object falling into it, the reception of messages is limited to a shrinking horizon. This phenomenon, which is only theoretically accessible, is called asymptotic silence .
The "Principles of Black Hole Dynamics"
For black holes, the general theory of relativity follows laws that are strikingly similar to those of thermodynamics. Black holes behave in a similar way to a black body , so they have a temperature. The following laws apply in detail:
- The first law of the "black hole dynamics" is, as in ordinary thermodynamics , the law of conservation of energy , but taking into account the relativistic energy-mass equivalence . The other conservation laws of mechanics and electrodynamics also apply : In addition to energy, momentum , angular momentum and charge are retained.
- The second law of the “black hole dynamics” - postulated by Stephen W. Hawking - states that the sum of the areas of the event horizons can never decrease, no matter what happens to the black holes. This applies not only when matter falls into the black hole (which increases its mass - and thus its event horizon -), but also for the merging of two black holes and for every other conceivable process. This corresponds to the second law of thermodynamics , whereby the area of the event horizon takes on the role of entropy . The Bekenstein-Hawking entropy of the black hole is (explanation of the symbols: see below). Black holes have the highest entropy of all known physical systems of the same mass.
Hawking radiation
Quantum theoretical considerations show that every black hole also emits radiation. This seems to contradict the statement that nothing can leave the black hole. However, the process can be interpreted as the production of particle / antiparticle pairs close to the Schwarzschild radius, where one of the particles falls into the center of the black hole while the other escapes into the surroundings. In this way, a black hole can shed particles without anything crossing the event horizon from the inside out. The energy for this process, known as Hawking radiation , comes from the gravitational potential of the black hole. This means that it loses mass due to the radiation.
Seen from the outside, it looks as if the black hole is “evaporating” and slowly becoming smaller, the smaller the faster. If there were very small black holes during the Big Bang, then they would have completely evaporated in the meantime. The resulting radiation would be very characteristic and could serve as evidence of such holes. However, this radiation has not yet been found. This results in an upper limit for the number of small black holes created during the Big Bang.
Black holes formed from stars in the main sequence emit very little Hawking radiation and evaporate on a timescale that is dozens of orders of magnitude longer than the age of the universe . At the moment they are growing just by absorbing the background radiation .
Entropy and temperature
Hawking realized in 1974 after preliminary work by the Israeli physicist Jacob Bekenstein that black holes have a formal entropy and a temperature . The formal entropy of a black hole is proportional to the surface of its horizon and otherwise only dependent on natural constants. The temperature corresponds to the thermal energy spectrum of Hawking radiation and is inversely proportional to the mass of the black hole:
- or
- or
It is the reduced Planck's constant , the speed of light , the circle number Pi, the Boltzmann constant , the gravitational constant , the mass and the Schwarzschild radius.
From the equation it can be calculated that a black hole with a mass that corresponds to 2.4% of the earth's mass would be as hot as the cosmic background radiation (2.725 K), i.e. would have the same spectrum.
lifespan
Since a black hole is constantly losing energy in the form of Hawking radiation, it will be completely annihilated after a certain period of time , provided it cannot take up any new mass during this period. This time span is calculated through
where the mass of the black hole at the beginning of the period and is a constant.
The no-hair theorem and the information paradox of black holes
A uniqueness theorem by Werner Israel states that a black hole is completely characterized by mass (see Schwarzschild metric ), electrical charge (see Reissner-Nordström metric ) and angular momentum (see Kerr metric ). This prompted John Archibald Wheeler to state that "black holes have no hair". One speaks therefore of the no-hair theorem, no-hair theorem or bald head. Further information from inside cannot be obtained, not even from the Hawking radiation, since it is purely thermal.
The no-hair theorem suggests that black holes cause a loss of information, since the Hawking radiation produced during dissolution does not contain any information about the history of the black hole's formation. The disappearance of information contradicts a basic principle of quantum mechanics, the postulate of the unitarity of the evolution of time. The problem is also known as the black hole information paradox .
Prominent representatives of this view were Kip Thorne and, for a long time, Stephen Hawking. Stephen Hawking changed his mind, however, and stated at the 17th International Conference on General Relativity and Gravitation (July 18-23, 2004 in Dublin) that black holes could have hair. Furthermore, among others, Roger Penrose, John Preskill and Juan Maldacena assume that at least certain information could additionally leak out. In his book The Universe in a Nutshell, Stephen Hawking also expresses the assumption that black holes would release the information they had collected when they dissolved. The information paradox has been tightened by Joseph Polchinski in the firewall paradox. In 2013, Juan Maldacena and Leonard Susskind proposed a solution through the equivalence of quantum entanglement and wormholes (ER-EPR conjecture), further expanded through an explicit proposal of such traversable wormholes by Ping Gao, Daniel Louis Jafferis and Aron C. Wall (see wormhole ) . The area is controversial, and Hawking came back to it in one of his last publications.
A more recent approach suggests testing the no-hair theorem based on the precession of the orbital ellipses of two stars orbiting closely around Sagittarius A * . If the no-hair theorem is true, then the ratio of the two precession rates should only depend on the angular momentum of the suspected black hole Sagittarius A *. Should it turn out that the ratio of the precession rates obey more complicated relationships, the no-hair theorem would be refuted.
Binary black hole
Emergence
A distinction is made between two ways in which binary black holes arise. On the one hand, it can originate from two strongly interacting galaxies when they have collided and swing-by processes seem to play a role. As an example of a previous collision, it is believed that the supermassive black hole in the center of M87 was formed by merging.
On the other hand, an interacting double star can be the starting point if both stars are very massive. After a wind Roche-Lobe overflow , a black hole plus a white dwarf is usually created. Alternatively, however, the overflow can be atypical and a common shell can develop in the meantime, so that ultimately two black holes are formed.
Merging
Once a black hole pair has formed, it can merge into a single black hole after a period of circling. In the Abell 400 galaxy cluster 300 million light years away , indications of the impending merger of two black holes have been found. Such a collision was detected for the first time in 2015 when, according to predictions, in the last fraction of a second before the merger, the extent of the acceleration with simultaneous release of matter or energy was so great that the gravitational wave generated in this way could be measured in the LIGO observatories.
Classification
great | Dimensions | Size (Schwarzschild radius) |
---|---|---|
Super massive black hole | ≈ 10 5 -10 10 M ☉ | ≈ 0.001-200 AU |
Medium black hole | ≈ 1000 M ☉ | ≈ 3000 km |
Stellar black hole | ≈ 10 M ☉ | ≈ 30 km |
Primordial black hole | up to ≈ M moon | up to ≈ 0.1 mm |
Black holes are divided into the classes shown on the right according to the method of formation and based on their mass, which are discussed below:
Super massive black holes
Supermassive (supermassive) black holes ( english supermassive black hole, SMBH ), the millions to billions of times the mass of the Sun have. They are located in the centers of bright elliptical galaxies and in the bulge of most or even all spiral galaxies . How they came into being and how their formation is related to the evolution of galaxies is the subject of current research.
The strong radio source Sagittarius A * (Sgr A * for short) in the center of the Milky Way is a supermassive black hole of 4.3 million solar masses. A few years ago the mass estimate based on the observation of gas clouds (e.g. the so-called mini-spiral) was still around 2.7 million solar masses. Thanks to the improved resolution and sensitivity of the telescopes, the mass for the black hole in the center of the galaxy could be specified more precisely by analyzing the trajectories of, for example, S0-102 or S0-2 .
Natarajan and Treister have developed a model that predicts an upper mass limit on the order of 10 billion solar masses. The reason is - explained clearly - in the fact that the falling matter is accelerated by the gravitational force of such a supermassive black hole in such a way that a stable orbit outside the Schwarzschild radius results. In addition, the electromagnetic radiation and the “matter winds” that are emitted by the matter in the accretion disk act as resistance to further incident matter, so that a balance is ultimately established between incident and repelled matter (see Eddington limit ).
One unsolved mystery is the formation of supermassive black holes in the early universe. It is known that as early as 700 million years after the Big Bang, super-massive holes of around 2 billion solar masses existed ( ULAS J1120 + 0641 ). The most distant known object ULAS J1342 + 0928 as of December 2017 , less than 690 million years after the Big Bang, is already a supermassive black hole. Most scientists agree that they originated from smaller black holes, with one camp seeing these "seeds" in black holes of a maximum of a few hundred solar masses, the other in those of thousands to tens of thousands of solar masses. The former are easier to manufacture, but must have a rapid growth mechanism that bypasses the Eddington limit. In the second case, the black holes start with a larger initial mass and can absorb more mass from gas clouds in the vicinity before they reach the Eddington limit, but a theory is required that naturally explains their existence. In 2017, N. Yoshida and colleagues published a simulation of the early universe, in which supermassive stars of around 34,000 solar masses are formed by the interaction of very supersonic gas winds and the dynamics of clumps of dark matter that then collapse into a black hole. In other scenarios, the intense UV light of young stars from neighboring galaxies prevents star formation in a gas cloud until it collapses directly into a black hole of around 100,000 solar masses. It is hoped that the James Webb Space Telescope will provide more information about stars and gas clouds in the early universe .
In 2008, a Swiss team from the Swiss Federal Institute of Technology in Lausanne (EPFL) headed by Alexander Eigenbrod observed an energy-rich ring formation around a quasar 10 billion light years away , the Einstein Cross in the constellation Pegasus , at the VLT and thus very well confirmed the theory of super-massive holes.
The largest known black hole is TON 618 with an estimated 66 billion solar masses. Another example of an estimated 21 billion solar masses is in the center of the galaxy NGC 4889 (2011). With a supermassive black hole of about 20 billion solar masses, the quasar APM 08279 + 5255 (about 12 billion light years away), around which enormous amounts of water vapor were discovered in 2011, is also one of the most massive candidates known to date.
In the center of the relatively nearby galaxy M87 (approx. 55 million light-years away) a black hole with a mass of 6.6 billion solar masses has been detected.
Supermassive black holes were also found in (ultra-compact) dwarf galaxies (first in 2014 in M60- UCD 1), which indicates that these originated as “normal” galaxies, from which a large part of the stars were torn from collisions with larger galaxies.
In September 2017, the discovery of a double supermassive black hole was published, which could be observed with the help of Very Long Baseline Interferometry (VLBI). These are two black holes orbiting each other at a distance of 1.1 light years with a total mass of 36 million solar masses in the spiral galaxy NGC 7674, which is 380 million light years away .
In 2015, with the help of NuStAR and XMM-Newton, it was discovered that supermassive black holes emit “plasma winds” (gases from high-energy and highly ionized atoms) in a spherically symmetrical form and that these are strong enough to prevent star formation in large areas of the host galaxy. Due to their spherical symmetry, they differ significantly from jets. In 2017 it was shown at the Keck Observatory that the winds of black holes (in this case in Quasar 3C 298 9.3 billion light years away ) even have the ability to actively shape the entire host galaxy. The galaxy has only one-hundredth the mass that would be expected from the normal relation between the mass of supermassive black holes and their host galaxies.
Medium weight black holes
Moderate Black Holes ( English intermediate-mass black hole, IMBH ) from a few hundred to a few thousand solar masses may arise as a result of stellar collisions and -verschmelzungen. In early 2004, researchers published the results of a study of neighboring galaxies with the Chandra space telescope , in which they found indications of medium- weight black holes in so - called ultra-luminous X-ray sources ( ULX ). After that, however, based on observations with the VLT and the Subaru telescope, there were strong doubts that ULX were medium-weight black holes.
New candidates are the centers of the globular clusters Omega Centauri in the Milky Way and Mayall II in the Andromeda galaxy , as well as in the spiral galaxy Messier 82 and in a dwarf Seyfert galaxy .
Stellar black holes
Stellar black holes ( English stellar black hole, SBH ) represent the final stage of the evolution of massive stars . Stars with an initial mass less than three solar masses cannot become black holes. They end their life as a comparatively unspectacularly cooling remnant of stars ( white dwarf / neutron star ). Stars with an initial mass greater than three solar masses (such as blue giants ) go through the higher stages of nucleosynthesis at the end of their life until they burn silicon . They explode in a core collapse supernova , with the remaining star remnants collapsing into a black hole if it still has more than 2.5 solar masses ( Tolman-Oppenheimer-Volkoff limit ). Otherwise, stars up to 15 times the solar mass - depending on how much mass they lose as a supernova - can also end up as neutron stars if the remaining mass is between 1.5 and 2.5 solar masses. Neutron stars can develop into black holes through the accretion of further matter - for example as compact companions in an X-ray binary star .
By observing gravitational waves , the merging of two stellar black holes with about 36 and 29 solar masses could be observed in September 2015. The resulting black hole has a mass of about 62 solar masses (the energy of 3 solar masses was radiated as gravitational waves). This is the most massive known stellar black hole (as of March 2016).
Another very massive black hole in the dwarf galaxy IC 10 in the constellation Cassiopeia has a mass of 24 to 33 solar masses. It is part of a binary star system. The black hole was discovered indirectly through the X-ray radiation of the accompanying star, which fluctuated in strength, which may be an indication of an object periodically covering the source. Calculations from data from the Swift satellite and the Gemini telescope on Hawaii confirmed the assumptions.
In 2008, the candidate for the smallest black hole was XTE J1650-500 , also an X-ray binary star whose mass is now estimated to be around 10.7 solar masses. IGR J17091-3624 is currently being investigated. It is a binary star system made up of a normal star and a black hole, which is estimated to be less than three solar masses based on the changes in its X-ray signal. In November 2019 a candidate for a black hole of only around 3.3 solar masses (within the limits 2.6 to 6.1) in a binary star system was reported (2MASS J05215658 + 4359220). The compact object does not act with its companion star via the accretion of mass and was therefore not identified by the X-ray emission but by the effect of gravity; it does not itself emit any radiation. It is either a black hole or an unusual neutron star (commonly the upper limit for the mass of neutron stars is estimated at 2.5 solar masses).
Primordial black holes
In 1966 Jakow Borissowitsch Seldowitsch and Igor Dmitrijewitsch Novikow and in 1971 Stephen Hawking , who dealt with this in more detail, were the first to suggest that there might be so-called primordial black holes in addition to the black holes created by supernovae . These are black holes that were already formed during the Big Bang in areas of space in which the local mass and energy density was sufficiently high (if you count back the constantly decreasing matter density in the universe, you will find that it is in the first thousandth of a second after the Big Bang exceeded the density of the atomic nucleus). The influence of fluctuations in the even density distribution (see cosmic background radiation ) in the early universe was also decisive for the formation of primordial black holes, as was the accelerated expansion during the inflation phase after the Big Bang. At that time, small black holes with a mass of around 10 12 kilograms could have formed. For such a black hole, a Schwarzschild radius of only about 10 −15 meters or a femtometer is given, less than the classical size of a proton. It would therefore be extremely difficult to localize in space using optically based methods. The small Jupiter moons S / 2003 J 9 and S / 2003 J 12 with a diameter of around 1 km or an Irish mountain of similar size have a similar mass . Since the mid-1990s it has been discussed whether the shortest gamma-ray bursts measured on Earth could come from radiating primordial black holes, because their calculated lifespan is on the order of the age of the present-day universe.
From his reflections on small black holes, Hawking concluded in 1974 the existence of the Hawking radiation named after him, meaning that black holes can not only swallow matter but also release it again. Although the existence of primordial black holes is by no means certain, valuable new insights in the field of cosmology , quantum physics and the theory of relativity have resulted from hypothetical considerations alone .
Black micro-holes
According to some unified theories , such as string theory , the minimum mass for black holes should be well below the Planck mass , so that black micro-holes could arise in the operation of future particle accelerators. Indeed, for this reason, the operation of the LHC accelerator has been opposed and even sued since 2008 . The lawsuit was rejected in the final instance in 2012. The plaintiffs feared that such a micro-hole could fall into the core of the earth, grow there and eventually absorb the whole earth. This is contradicted by the fact that the theories that predict the micro-holes also ascribe an extremely short lifespan to them. In addition, nothing has happened to the earth for billions of years, despite permanent collisions with even more energetic cosmic rays .
Observation methods
Black holes do not emit any observable light or other measurable radiation. According to current theories, black holes are able to give off energy in the form of so-called Hawking radiation . If so, it would mean that black holes gradually “evaporate”, the smaller the mass of the black hole, the faster this process is. But the Hawking radiation would be so low in energy that it could not be distinguished from the usual background.
In contrast, the effects on matter outside the event horizon are observed.
The consequences of the falling of matter are of particular importance for the discovery of black holes. Since the event horizon encloses a very small area for cosmic conditions, the incident matter is subject to a very high optical compression and acceleration due to gravitational forces in an area in front of the event horizon. With rotating black holes, this happens in the form of an accretion disk. There the matter rubs against each other and releases large amounts of energy, both as electromagnetic radiation and as the acceleration of particles through electromagnetic fields and impact processes. One result of these processes are jets of matter that are ejected perpendicular to the accretion disk along an axis through the black hole. These jets are particularly noticeable in the case of supermassive black holes: there, the charged particles flow into the intergalactic medium with such great accelerations that they reach far beyond their original galaxy. In addition, accelerated charged particles generate synchrotron radiation , which leads to strong gamma-ray emissions from such jets. This was observed e.g. B. At the end of 2007 at the black hole in the center of the galaxy 3C 321 . Another well-known example is the galaxy M 87 with the impressive jet of its central black hole.
Historically, there are many types of active galaxy nuclei , depending on our perspective on the object, the energy scales of the processes and the activity (how much matter is currently flowing into the object). The quasars are an example .
Kinematic proof
The orbit and the speed of stars orbiting the black hole are used as evidence. If an enormously high mass, which is also dark and dense, is calculated, the assumption is that it is a black hole. The measurement of the orbit of the star S2 , which orbits Sgr A * in the center of our Milky Way on a Kepler orbit, allowed very precise statements to be made about the mass concentration in the central area of Sgr A *. In another kinematic method, the Doppler shift and the distance between the dark object and the star orbiting it are determined, from which the gravitational redshift and then the mass can be estimated.
Eruptive evidence
Stars that come too close to the tidal radius of a black hole can be torn apart by the tidal forces and thereby release characteristic X-rays that can be detected by devices such as the Nuclear Spectroscopic Telescope Array .
Aberrative evidence
Black holes have the ability to deflect or focus electromagnetic radiation, which makes it possible to identify them. For example, if the shape of the elliptical orbit of a star appears distorted, it can be assumed that there is a black hole between the observer and the star.
Obscurative evidence
Due to the gravitational redshift , a black coloration can be seen at the edge of the black holes, since the relativistic redshift factor influences electromagnetic waves and thus the radiation in the vicinity of the event horizon is suppressed so that a black hole can be recognized.
Temporal evidence
Due to the temporal distortion (the so-called time dilation ) (recognizable by an analysis of the light curves ) that triggers a black hole in objects that orbit it or are in the vicinity, it is possible to identify a black hole as such.
Spectroscopy
Lens effects and gravitational shifts alienate the spectra of stars that are in the vicinity of black holes.
Gravitational waves
Accelerated black holes or black hole collisions can cause waves in spacetime that can be measured with gravitational wave detectors like LIGO . The observations of gravitational waves from the merging of two smaller black holes of 29 and 36 solar masses presented by LIGO in 2016 were the first direct evidence of gravitational waves (see Gravitational wave # Experimental evidence ).
Radio telescope recordings with VLBI
With Very Long Baseline Interferometry (VLBI), radio telescopes can achieve a resolution that is comparable to the radius of a black hole. The Event Horizon Telescope project has thus succeeded in recording images of the accretion flows around the supermassive black hole M87 * in the center of the galaxy Messier 87 and thus for the first time obtaining direct images of the surroundings of a black hole. The presentation in April 2019 of the results of the coordinated action from April 2017 is considered a scientific sensation that made it onto the front page of the news magazine Spiegel , for example . Due to gravitational and relativistic effects, the accretion flows and images of the heated gases in the vicinity of the black hole appear as a ring that encloses a dark area - the so-called " shadow " of the black hole. The shadow is an image, enlarged by the gravitational lensing , of the area bounded by the event horizon . On a linear scale, it is up to five times larger than the event horizon and is limited by the photon orbit, on which light circulates around the black hole and, in the event of small disturbances, either disappears in the black hole or penetrates outwards. By comparing them with computer simulations, the images allow conclusions to be drawn about the mass and rotation of the black hole, but not yet about the angular momentum. According to the current state of the art, only the shadow of the supermassive black holes in M87 and Sagittarius A * in the center of the Milky Way is so large that they can be observed with the EHT. The EHT also took pictures of Sagittarius A, but due to the much more dynamic nature of Sagittarius A, they are more indistinct and will be presented soon (as of April 2019). Sagittarius A has a smaller mass, but is also closer to earth. The shadow therefore appears about the same size.
Known black holes
Sagittarius A *
Sagittarius A * is the supermassive black hole in the center of the Milky Way . Since 1992, a team of astronomers has been studying its surroundings, mainly in the infrared range. The orbits and the speeds of 28 stars were measured. Near-infrared cameras with adaptive optics were used at the Very Large Telescope in Cerro Paranal in Chile, the imaging spectrograph Sinfoni, the speckle imaging camera SHARP I and other instruments from the European Southern Observatory . In addition, observations from the Keck telescope on Hawaii, the New Technology telescope and images from the Hubble telescope were evaluated.
The investigations showed that the central mass can only be explained by a black hole and that around 95% of the total mass in the observed sector must be in this black hole. The measurements of the infrared and X-ray emissions in the accretion zone indicate that the black hole has a high angular momentum.
More black holes in the Milky Way
In addition to the assumed central black hole in our galaxy , namely Sagittarius A * with approx. 4.3 million solar masses , there are a number of other assumed small black holes that are distributed in the Milky Way and have a mass of a few to a dozen solar masses . They are all components of double or multiple star systems, apparently pulling off matter from their partner in an accretion disk and radiating in the X-ray range .
The latest research results show that in the star group IRS 13, which is only three light years away from Sgr A *, there is a second black hole with a comparatively low 1,300 solar masses. It is currently not clear whether it will unite with Sgr A * in the future, whether it will be in a stable orbit, or even move away from it.
In January 2005, the Chandra X-ray telescope was used to observe outbursts of brightness near Sgr A *, which suggest that there are 10,000 to 20,000 smaller black holes within a radius of about 70 light years that orbit the supermassive central black hole in Sgr A * . According to one theory, these are supposed to "feed" the central black hole with stars from the surrounding area at regular intervals.
The so far closest known black hole belongs together with two stars visible to the naked eye to the multiple system HR 6819 in the constellation Telescope and is around 1000 light years away. It has at least four times the solar mass. One of the companion stars orbits the black hole in 40 days.
Surname | Mass ( M ☉ ) |
Mass partner ( M ☉ ) |
Rotation time (days) |
Estimated Distance from Earth ( Lj ) |
---|---|---|---|---|
≈ | 1,000||||
A0620−00 | ≈ 11 | ≈ 0.7 | 0.33 | ≈ | 3,500
GRO J1655-40 | ≈ 7.0 | ≈ 2.34 | 2.62 | ≈ 10,500 |
XTE J1118 + 480 | 6-8 | 0.17 | ≈ | 6,200|
Cyg X-1 | 7-13 | 0.25 | 5.6 | 6,000-8,000 |
GRO J0422 + 32 | 3-5 | 1.1 | 0.21 | ≈ | 8,500
GS 2000 + 25 | 7-8 | 4.9-5.1 | 0.35 | ≈ | 8,800
V404 Cyg | ≈ | 90.5 | 6.5 | ≈ 10,000 |
XTE J1650−500 | ≈ | 3.8≈ 2.7 | 0.32 | ≈ 15,000 |
V4641 Sagittarii | ≈ 10 | ≈ 7 | 2.82 | 10,000-25,000 |
GX 339-4 | 5-6 | 1.75 | ≈ 15,000 | |
GRS 1124-683 | 6.5-8.2 | 0.43 | ≈ 17,000 | |
XTE J1550-564 | 10-11 | 6.0-7.5 | 1.5 | ≈ 17,000 |
XTE J1819−254 | 10-18 | ≈ 3 | 2.8 | <25,000 |
4U 1543-475 | 8-10 | 0.25 | 1.1 | ≈ 24,000 |
Sgr A * | 4.3 million | - | - | ≈ 25,000 |
Others
In the galaxy NGC 6240 there are two black holes that orbit each other at a distance of 3000 light years and will merge in a few hundred million years.
As of 2010, the most distant stellar black hole in the observable universe was tracked down by astronomers from the European Southern Observatory (ESO) using the Very Large Telescope at the Paranal Observatory in the galaxy NGC 300 .
The first black hole outside of our galaxy was detected in 1982 in the Large Magellanic Cloud about 150,000 light years away and forms a component of the X-ray binary star LMC X-3.
In the center of NGC 4889 is (as of December 2011) the largest directly measured black hole with a mass of an estimated 21 billion solar masses (“best fit” from the range 6 to 37 billion solar masses).
The black hole with the catalog number SDSS J0100 + 2802 is very old, the state is observed from Earth 875 million years after the Big Bang. At this point in time its mass was already around twelve billion solar masses. It's unclear how it got so massive so early.
Alternative explanations for ultra-compact dark objects
Some alternative explanations have been proposed for ultra-compact dark objects that do not have singularities and have no information paradox. Since these models do not make predictions that can be observed with today's means that would distinguish them from a black hole, acceptance in the specialist literature is low. One example is the hypothetical gravastars , also known as “Quasi Black Hole Objects” (QBHO). The inventors of the theory, Mazur and Mottola, suggested that the theory provides a solution to the black hole information paradox and that gravastars could be sources of gamma-ray bursts. The theory attracted little public interest because the theory has no advantage over the black hole theory and is purely speculative. Another attempt to solve the information paradox based on string theory comes from Samir Mathur. According to this “ball of lint model”, the event horizon covers a conglomerate of branes and strings , and is not itself sharply delineated.
Adaptations in Science Fiction
Black holes are often portrayed in science fiction literature as a possible means of transporting faster than light , for example in Stanisław Lem's novel Fiasko , or as the ultimate possibility of generating energy , for example in the TV series Stargate .
The 1979 film " The Black Hole " - starring Maximilian Schell and Anthony Perkins - which, among other things, addresses the strong gravitational force of black holes, was nominated for two Oscars in 1980 . The film Interstellar from 2014 by director Christopher Nolan also includes the topics of the black hole and gravitational forces. In the television series Andromeda, the spaceship Andromeda Ascendant comes close to the event horizon of a black hole, which causes the ship and crew to freeze due to the time dilation until they are recovered and thus for 300 years in time.
See also
literature
- Kip S. Thorne: Curved Space and Warped Time. Droemer Knaur, Munich 1996, ISBN 3-426-77240-X .
- Kip S. Thorne: Black Holes and Time Warps: Einstein's Outrageous Legacy. WW Norton & Company , New York 1994, ISBN 0-393-31276-3 .
- Max Camenzind: From Recombination to the Formation of Black Holes. In: Stars and Space. Heidelberg 44.2005,3, pp. 28-38. ISSN 0039-1263 .
- Stephen W. Hawking: A Brief History of Time. Rowohlt Tb., Reinbek near Hamburg 1988, ISBN 3-499-60555-4 .
- Stephen W. Hawking: The Universe in a Nutshell. 2nd Edition. Dtv, Munich 2004, ISBN 3-423-34089-4 .
- Bernard J. Carr, Steven B. Giddings: Black holes in the laboratory. In: Spectrum of Science. Heidelberg 2005, 9, ISSN 0170-2971 .
- Ute Kraus: Destination - Black Hole. In: Stars and Space. Heidelberg 2005, 11. ISSN 0039-1263 .
- Rüdiger Vaas: Tunnel through space and time. 6th edition. Franckh-Kosmos, Stuttgart 2013, ISBN 978-3-440-13431-3 .
- Stephen W. Hawking: The Shortest History of Time. Rowohlt Tb., Reinbek near Hamburg 2006, ISBN 3-499-62197-5 .
- Mitchell Begelman, Martin Rees: Black holes in the cosmos - the magical attraction of gravity. Spektrum Akademischer Verlag, Heidelberg 2000, ISBN 3-8274-1044-4 .
- Fulvio Melia: The galactic supermassive black hole. Princeton Univ. Pr., Princeton 2007, ISBN 978-0-691-09535-6 .
- Pietro Fré: Classical and quantum black holes. Inst. Of Physics Publ., Bristol 1999, ISBN 0-7503-0627-0 .
- Hyun Kyu Lee et al. a .: Black hole astrophysics 2002. World Scientific, Singapore 2002, ISBN 981-238-124-4 .
- Valerij P. Frolov et al. a .: Black hole physics - basic concepts and new developments. Kluwer, Dordrecht 1998, ISBN 0-7923-5146-0 .
- Piotr T. Chruściel, João Lopes Costa, Markus Heusler: Stationary Black Holes, Uniqueness, and Beyond . In: Living Rev. Relativity . tape 15 , no. 7 , 2012 ( livingreviews.org [PDF; 1.4 MB ; accessed on December 15, 2012]).
Web links
- The colorful world of black holes. In: Werner Kasper: Adventure Universe. Detailed but easy to understand.
- The Nature of Space and Time. Lectures by Stephen Hawking (Part 2 contains a caricature of the no-hair theorem).
Videos
- What are black holes? from the alpha-Centauri television series(approx. 15 minutes). First broadcast on Jan 3, 1999. On Jan 3, 1999.
- Are there black holes in the Milky Way? from the alpha-Centauri television series(approx. 15 minutes). First broadcast on May 9, 1999. May 9, 1999.
- Where is the next black hole? from the alpha-Centauri television series(approx. 15 minutes). First broadcast on June 4, 2000. From June 4, 2000.
- Are black holes merging? from the alpha-Centauri television series(approx. 15 minutes). First broadcast on May 27, 2001. May 27, 2001.
- Do black holes move in space? from the alpha-Centauri television series(approx. 15 minutes). First broadcast on Jan 20, 2002. Jan 20, 2002 (treated by KV Ursae Majoris ).
- Do black holes dance? from the alpha-Centauri television series(approx. 15 minutes). First broadcast on Jan 21, 2004. January 21, 2004.
- Do black holes eat stars? from the alpha-Centauri television series(approx. 15 minutes). First broadcast on Oct 27, 2004. October 27, 2004.
- Are black holes rotating? from the alpha-Centauri television series(approx. 15 minutes). First broadcast on Feb 16, 2005. February 16, 2005.
- Is there a 2nd black hole in the Galactic Center? from the alpha-Centauri television series(approx. 15 minutes). First broadcast on May 10, 2006. On May 10, 2006.
References and comments
- ^ Astronomers Capture First Image of a Black Hole. Event Horizon Telescope (EHT), accessed April 14, 2019 .
- ^ The Nobel Prize in Physics 2020 . In: nobelprize.org, October 6, 2020.
- ^ Letter to Henry Cavendish , quoted from en: Dark star (Newtonian mechanics) .
- ↑ Karl Schwarzschild : About the gravitational field of a mass point according to Einstein's theory. In: Session reports of the Royal Prussian Academy of Sciences . 1, 189-196 (1916).
- ^ R. and H. Sexl: White dwarfs, black holes. RoRoRo paperback, 1979.
- ↑ World Wide Words: Black Hole.
- ^ David L. Meier: Black Hole Astrophysics. The Engine Paradigm. 2009, p. 397.
- ^ John Antoniadis: Multi-Wavelength Studies of Pulsars and Their Companions. 2015, p. 4.
- ↑ Matt Visser: The Kerr spacetime: A brief introduction. (PDF; 321 kB), p. 35, Fig. 3. First publication: arxiv : 0706.0622 .
- ↑ Bent spacetime. Satellite measurements prove Einstein to be right. In: Spiegel.de. April 15, 2007, accessed September 1, 2018 .
-
↑ To justify the given formulas, two very simplistic plausibility arguments apply :
In thermodynamics , the formula where stands for reversibly supplied heat energy (in the case of irreversible supply, the smaller symbol applies instead). is the (complete) differential of entropy and is the absolute temperature . The heat energy supplied (e.g. through radiation of particles into the black hole) is proportional to the area of the event horizon. As in thermodynamics, the “useful energy share” is proportional to the weighting factor (not to ) and given by where the mass of the black hole and the speed of light are (cf. “ E = mc 2 ”). - ^ Clifford Will : Testing the General Relativistic “No-Hair” Theorems Using the Galactic Center Black Hole Sagittarius A *. In: Astrophysical Journal Letters. 674, 2008, pp. L25-L28, doi: 10.1086 / 528847 .
- ↑ Mathematically rigorous statements about the validity or invalidity of the no-hair theorem can be found in a “ Living Review ” at the end of the bibliography.
- ^ The LIGO Scientific Collaboration, the Virgo Collaboration: Search for gravitational waves from binary black hole inspiral, merger and ringdown. 2011, arxiv : 1102.3781 .
- ↑ Krzysztof Belczynski, Tomasz Bulik, Bronislaw Rudak: The First Stellar Binary Black Holes: The Strongest Gravitational Wave Sources burst. 2004, arxiv : astro-ph / 0403361 .
- ↑ Two black holes before merging. In: astronews.com.
- ↑ A monster in the sights. Astronomers measure the black hole in the center of the Milky Way. In: Wissenschaft.de. December 10, 2008, archived from the original on July 29, 2009 ; Retrieved October 1, 2009 .
- ↑ Natarajan, Treister: Is there an upper limit to black hole masses? 2008. bibcode : 2009MNRAS.393..838N .
- ↑ D. Mortlock et al.: A luminous quasar at a redshift of z = 7.085. Nature, Volume 474, 2011, pp. 616-619.
- ↑ Eduardo Bañados et al. a .: An 800-million-solar-mass black hole in a significantly neutral Universe at a redshift of 7.5 . In: Nature . December 6, 2017. arxiv : 1712.01860 . bibcode : 2018Natur.553..473B . doi : 10.1038 / nature25180 . Retrieved August 18, 2018.
- ↑ Yasemin Saplakogu: zeroing in on how supermassive black holes FORMED. In: Scientific American. Online edition, September 29, 2017.
- ↑ Shingo Hirano, Takashi Hosokawa, Naoki Yoshida, Rolf Kuiper: Supersonic gas streams enhance the formation of massive black holes in the early universe. In: Science. Volume 357, 2017, pp. 1375-1378. Arxiv.
- ↑ John Regan, John H. Wise, Greg Bryan, and others. a .: Rapid Formation of Massive Black Holes in close proximity to Embryonic Proto-Galaxies. In: Nature Astronomy. March 2017, Arxiv.
- ↑ Theory about rings around black holes confirmed. In: Welt.de. December 15, 2008, accessed October 1, 2009 .
- ^ A b Nicholas J. McConnell: Two ten billion solar mass black holes at the centers of giant elliptical galaxies. (PDF) Nature, December 8, 2011, archived from the original on December 6, 2011 ; Retrieved December 6, 2011 .
- ↑ Spectrum of Quasar APM 08279 + 5255. English.
- ↑ 12 billion light years away. US researchers discover gigantic water reservoir in space. Water vapor at APM 08279 + 5255 sets records for volume and distance. At: Spiegel.de.
- ↑ MPE astronomer finds most massive black hole in galaxy M87. Max Planck Institute for Extraterrestrial Physics (MPE), June 8, 2009, archived from the original on January 2, 2010 ; accessed on April 20, 2019 .
- ↑ mass record. The heaviest object in the universe. In: Spiegel Online . January 17, 2011, retrieved on April 20, 2019 (New mass determination of the black hole in M87).
- ↑ Harald Zaun: A monster pulsates in the center of a dwarf galaxy. In: Welt.de. 18th September 2014.
- ↑ Anil Seth, Matthias Frank, Nadine Neumayer u. a .: A supermassive black hole in an ultra-compact dwarf galaxy. ( Memento of October 2, 2014 in the Internet Archive ). In: Nature. Volume 513, 2014, pp. 398-400, abstract.
- ↑ Rainer Kayser: Double black hole observed. Welt der Physik from September 18, 2017, accessed on September 20, 2017.
- ↑ How black hole winds blow. In: NASA.gov. 17th August 2015.
- ↑ Michelle Starr: We Finally Have Evidence That Black Holes Drive Winds Shaping Their Entire Galaxy. In: ScienceAlert.com. December 28, 2017.
- ↑ A. Vayner, Shelley Wright, et al. a .: Galactic-scale Feedback Observed in the 3C 298 Quasar Host Galaxy. In: Astroph. J. Volume 851, 2017, No. 2, abstract.
- ↑ Astronomers Shed Light on Formation of Black Holes and Galaxies. In: KeckObservatory.org. 20th December 2017.
- ↑ MW Pakull u. a .: Ultraluminous X-Ray Sources, Bubbles and Optical Counterparts. Preprint.
- ↑ A black hole in Omega Centauri. In: Stars and Space. May 2008, p. 21. ISSN 0039-1263 .
- ↑ Xiaobo Dong et al. a .: SDSS J160531.84 + 174826.1: A Dwarf Disk Galaxy With An Intermediate-Mass Black Hole. Preprint.
- ↑ Massive Black Hole Smashes Record. At: NASA.gov.
- ↑ microquasars. The heartbeat of a black hole. At: Astronews.com.
- ↑ Todd A. Thompson et al. a .: A noninteracting low-mass black hole-giant star binary system. Science, Volume 366, November 1, 2019, pp. 637-640, online
- ^ Zeldovich, Novikov, The Hypothesis of Cores Retarded During Expansion and the Hot Cosmological Model, Soviet Astronomy, Volume 10, Issue 4, 1966, pp. 602-603
- ↑ M. Sasaki et al. a .: Primordial Black Holes - Perspectives in Gravitational Wave Astronomy, Classical and Quantum Gravity, Arxiv 2018
- ^ Hawking: Gravitationally collapsed objects of very low mass , Mon. Not. R. Astron. Soc., Vol. 152, 1971, p. 75
- ^ Greg Landsberg: Black Holes at Future Colliders and Beyond. (PDF; 191 kB), lecture at the 10th SUSY conference, June 2002, DESY / Hamburg.
- ^ Felix Knoke: Black Holes in Geneva. Fear of the end of the world - Americans complain against particle accelerators. In: Spiegel.de. March 31, 2008.
- ↑ Fear of the end of the world. Lawsuit against Cern finally failed. In: Spiegel.de. October 16, 2012.
- ↑ Norbert Frischauf: Doomsday at CERN? The Orion, October 25, 2008, accessed July 5, 2014 .
- ↑ a b c d e Andreas Müller: How to discover a black hole. In: Wissenschaft-online.de.
- ↑ At the end of space and time. In: Der Spiegel. No. 16, April 13, 2019.
- ↑ Ulf von Rauchhaupt: It is not a naked singularity. In: Frankfurter Allgemeine Sonntagszeitung. April 14, 2019, p. 56.
- ↑ Kazunori Akiyama et al. a. (Event Horizon Telescope Collaboration): First M87 Event Horizon Telescope Results. I. The Shadow of the Supermassive Black Hole. In: Astroph. J. Letters. April 10, 2019, IOPScience.
- ↑ Galactic Black Hole disrupts Gas Cloud. In: mpe.mpg.de. Retrieved January 28, 2014 .
- ↑ R. Genzel et al. a .: Near IR Flares from Accreting Gas Near the last stable Orbit around the Supermassive Black Hole in the Galactic Center. In: Nature . London 425.2003, 954.
- ^ J. Casares: Observational evidence for stellar-mass black holes. arxiv : astro-ph / 0612312 , preprint.
- ↑ MR Garcia et al. a .: Resolved Jets and Long Period Black Hole Novae. arxiv : astro-ph / 0302230 , preprint.
- ↑ Sagittarius A * is swarmed around? of 10,000 little relatives. At: Bild der Wissenschaft. 2005. After a lecture by Michael Muno.
- ↑ See Sagittarius A * # Other black holes .
- ↑ ESO Instrument Finds Closest Black Hole to Earth. In: ESO.org. May 6, 2020, accessed May 6, 2020.
- ↑ Th. Rivinius, D. Baade, P. Hadrava, M. Heida, R. Klement: A naked-eye triple system with a nonaccreting black hole in the inner binary. In: Astronomy & Astrophysics. Volume 637, 2020, L3, online.
- ↑ The little black one. ( Memento of April 6, 2008 in the Internet Archive ). In: Wissenschaft.de. April 3, 2008, accessed February 2, 2019.
- ↑ New distance record for black holes. In: Press release ESO. Retrieved January 28, 2014 .
- ^ AP Cowley et al. a .: Discovery of a massive unseen star in LMC X-3. In: The Astrophysical Journal . 272, 118, 1983, bibcode : 1983ApJ ... 272..118C .
- ↑ Rainer Kayser: Super massive black hole too massive. On: weltderphysik.de. February 25, 2015, accessed February 26, 2015.
- ↑ P. Rocha et al. a .: Bounded excursion stable gravastars and black holes.
- ↑ Samir D. Mathur: Where are the states of a black hole?
- ^ NKS, Mathur states, 't Hooft-Polyakov monopoles, and Ward-Takahashi identities. At: wolframscience.com.