Gluconeogenesis
Parent |
Metabolism of glucose |
Gene Ontology |
---|
QuickGO |
The gluconeogenesis ( latinisierte case of gluconeogenesis , a compound word from ancient Greek γλυκύς glykys "sweet", νέος Neos "new" and γένεσις genesis "generation") is the formation of D - glucose of organic non-carbohydrate precursors, such as pyruvate , oxaloacetate and dihydroxyacetone phosphate . The metabolic pathway can be found universally in all living things. In humans and vertebrates , the starting materials are amino acids that come from the breakdown of proteins . Plants , fungi , most bacteria and some invertebrates can use the glyoxylate cycle to produce glucose from acetyl-CoA and thus from fatty acids .
Need for gluconeogenesis in humans
The daily glucose requirement of an adult person is around 200 g at rest, 75% of which is used by the brain and a large part of the rest by erythrocytes . The amount of glycogen stored in the body is around 400 to 450 g. About two thirds of this is stored in the muscles and about one third in the liver. The amount of glucose available in the blood is about 5 mmol / L, which corresponds to about 900 mg / L.
The erythrocytes of humans and mammals do not have mitochondria and are therefore completely dependent on the supply of glucose to generate energy, which they break down via glycolysis and subsequent lactic acid fermentation . The brain meets its enormous need for quickly available energy mainly through the breakdown of glucose. This is the main reason why the synthesis of glucose begins even during relatively short periods of hunger, which takes place mainly in the liver and kidney cortex and less so in the brain, skeletal and heart muscles. Due to the build-up of glucose in gluconeogenesis, the glucose concentration never drops below 3.5 mmol / L (about 600 mg / L). Around 180 to 200 g of glucose can be formed per day.
Course of gluconeogenesis
Cellular localization
The course of gluconeogenesis in eukaryotes is distributed over three compartments of a cell . The majority takes place in the cytosol . A reaction step takes place in the mitochondrion , another in the smooth endoplasmic reticulum (SER after English smooth endoplasmic reticulum ), since the necessary for each enzyme ( pyruvate carboxylase and glucose-6-phosphatase ) is present only here.
Reaction steps
The starting materials for gluconeogenesis are either (1) pyruvate or oxaloacetate as products of amino acid breakdown and lactic acid fermentation (from lactate ), (2) pyruvate formed anaerobically in muscles ( Cori cycle ), (3) dihydroxyacetone phosphate as a derivative of glycerol from fat breakdown or ( 4) Propionate , which remains after the last step of β-oxidation when uneven-numbered fatty acids are broken down. This is converted by propionyl-CoA carboxylase and a racemase (methylmalonyl-CoA epimerase) into succinyl-CoA , from which oxaloacetate is formed in the course of the citric acid cycle .
The structure of glucose from L- lactate is shown below:
![]() |
NAD + NADH + H + lactate dehydrogenase ![]() |
![]() |
HCO 3 - ATP ADP + P i pyruvate carboxylase ![]() |
![]() |
GTP GDP + CO 2 PEPCK ![]() |
![]() |
|
L- lactate | Pyruvate | Oxaloacetate | Phosphoenolpyruvate |
![]() |
+ H 2 O enolase ![]() |
![]() |
Phospho glycerate mutase ![]() |
![]() |
ATP ADP phospho glycerate kinase![]() |
![]() |
Phosphoenolpyruvate | D - 2-phosphoglycerate | D - 3-phosphoglycerate | D -1,3-bisphosphoglycerate |
![]() |
H 2 O P i fructose 1,6-bisphosphatase![]() |
![]() |
Glucose-6-phosphate isomerase![]() |
![]() |
H 2 O P i glucose-6-phosphatase![]() |
![]() |
β- D - fructose-1,6-bisphosphate | β- D - fructose-6-phosphate | α- D - glucose-6-phosphate | α- D - glucose |
Gluconeogenesis only partially corresponds to the reverse reaction of glycolysis . In glycolysis, however, there are three reactions in which the chemical equilibrium is almost exclusively on the side of the reaction products. These steps, all catalyzed by kinases , are:
- the conversion of glucose into glucose-6-phosphate,
- from fructose-6-phosphate to fructose-1,6-bisphosphate and
- the reaction of phosphoenolpyruvate (PEP) to pyruvate.
To reverse these reactions, the cell would have to be able to build up extreme concentrations. Therefore, these three steps in glycolysis are de facto irreversible and are circumvented in the reverse order in gluconeogenesis as follows:
- the carboxylation of pyruvate to oxaloacetate using ATP ( pyruvate carboxylase ) and the subsequent phosphorylating decarboxylation of oxaloacetate to PEP using GTP (phosphoenolpyruvate carboxykinase);
- the fructose-1,6-bisphosphatase catalyzes the reaction of fructose-1,6-bisphosphate to fructose-6-phosphate;
- Glucose-6-phosphate is converted into glucose by glucose-6-phosphatase (in glycolysis, a hexokinase or glucokinase (hexokinase IV) catalyzes the reverse reaction ).
The other conversion processes are in equilibrium, which is why they also play a role in gluconeogenesis.
Another important difference to glycolysis is the location of the reaction. While this takes place exclusively in the cytosol , gluconeogenesis is distributed over three compartments . The conversion of pyruvate to oxaloacetate takes place in the lumen of the mitochondrion . However, oxaloacetate cannot freely pass through the inner membrane of the mitochondrion and must first be converted. There are two ways of doing this. Either mitochondrial oxaloacetate is converted into PEP by a mitochondrial PEP carboxykinase. PEP then leaves the mitochondrion through a special anion shuttle system. In the cytoplasm, PEP is converted into glucose as a result of gluconeogenesis.
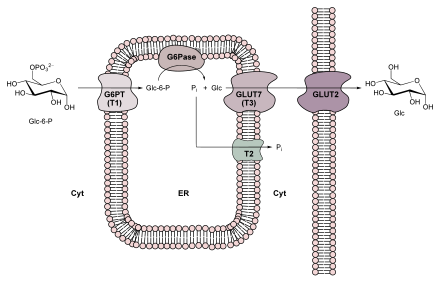
If you are hungry, a second route is taken for transport. In the liver , L - alanine is deaminated to pyruvate and thus serves as a source of oxaloacetate. When hungry, the amount of reducing agent in the form of NADH is low in the cytosol and high in the mitochondrion. However, NADH is required in the cytosol for gluconeogenesis. The so-called malate-aspartate shuttle system is used to transport both NADH and oxaloacetate from the mitochondrion into the cytosol . The oxaloacetate generated in the mitochondrion is reduced to L - malate by a mitochondrial malate dehydrogenase and can then be translocated through the inner membrane. In addition to the malate-aspartate shuttle, the mitochondrial dicarboxylate carrier is also available for transport. In the cytosol, a cytosolic malate dehydrogenase oxidizes malate to oxaloacetate, whereby NAD + is reduced to NADH and is used in gluconeogenesis.
The last reaction step in gluconeogenesis does not take place in the cytosol, but in the lumen of the endoplasmic reticulum (ER). The transport into the ER and the hydrolysis of glucose-6-phosphate is carried out by a glucose-specific membrane-enzyme complex consisting of glucose-6-phosphate translocase and glucose-6-phosphatase (see also figure on the right).
Pyruvate carboxylase
Pyruvate carboxylase is only active with its prosthetic group: biotin . Biotin acts as a mobile carrier of activated carbon dioxide . The biotin is bound to the ε-amino group of a specific lysine residue via its carboxy group . This creates a flexible arm, which allows the biotin group to “swing” from one active center to the second. The carboxylation takes place in two steps:
The first partial reaction depends on the presence of acetyl-CoA, without this no carboxylation of biotin is possible. This regulation is a form of allostery , as a high acetyl-CoA level is a sign of a greater need for oxaloacetate in the citric acid cycle. Acetyl-CoA is a powerful and the only effector of the enzyme. Oxaloacetate can either be used for glucogenesis or is incorporated into the citric acid cycle . The catalyzed reaction of pyruvate carboxylase is an example of an anaplerotic reaction . If there is an excess of ATP, the oxaloacetate is consumed in gluconeogenesis, which means that it is not enriched. The second reaction step of pyruvate carboxylase is acetyl-CoA independent.
Comparison of gluconeogenesis and glycolysis
Energy balance compared to glycolysis reversal
For the biosynthesis of one molecule of glucose, starting from pyruvate, four molecules of ATP and two molecules each of GTP and NADH are required.
The balance shown below makes it clear that the upper reaction will take place preferentially, since a direct reversal of glycolysis represents a thermodynamically unfavorable reaction:
This means that six ATP equivalents (2 GTP + 4 ATP) are required so that gluconeogenesis can take place to build up one molecule of glucose.
Gluconeogenesis and Glycolysis - Reciprocal Regulation
Gluconeogenesis and glycolysis share several enzymatic reactions, but are two completely opposing metabolic pathways. Therefore there is a need for regulation. It takes place in two places:
- in the reactions from pyruvate to PEP and
- in the conversion of fructose-1,6-bisphosphate to fructose-6-phosphate.
The first reaction: the conversion of PEP into pyruvate, which occurs in glycolysis, is catalyzed by pyruvate kinase . The activity of this enzyme is increased by fructose-1,6-bisphosphate and inhibited by ATP and alanine. The gluconeogenesis enzymes (pyruvate carboxylase and PEP carboxykinase) are activated by acetyl-CoA and inhibited by ADP. Since ATP is converted into ADP through hydrolysis , this type of regulation of two opposing reactions can be referred to as reciprocal regulation. Another example is the reaction listed under 2. The phosphofructokinase involved in glycolysis is stimulated by fructose-2,6-bisphosphate and adenosine monophosphate (AMP), but is also inhibited by citrate . Reciprocally, the regulation of the fructose-1,6-bisphosphatase involved in gluconeogenesis takes place (activated by citrate and inhibited by fructose-2,6-bisphosphate and AMP).
literature
- Geoffrey Zubay: biochemistry. 4th edition. Mcgraw-Hill Professional, 1999, ISBN 3-89028-701-8 .
- Donald Voet, Judith G. Voet: Biochemistry . Wiley-VCH, 1994, ISBN 3-527-29249-7 .
- Jeremy M. Berg, John L. Tymoczko, Lubert Stryer: Biochemistry. 6th edition. Spectrum Akademischer Verlag, Heidelberg 2007, ISBN 978-3-8274-1800-5 .
- H. Robert Horton, Laurence A. Moran, K. Gray Scrimgeour, Marc D. Perry, J. David Rawn, Carsten Biele (translator): Biochemie . 4th updated edition. Pearson Studies, 2008, ISBN 978-3-8273-7312-0 .
- Reginald Garrett, Charles M. Grisham: Biochemistry . (International Student Edition). 4th edition. Cengage Learning Services, 2009, ISBN 978-0-495-11464-2 .
- David L. Nelson, Michael M. Cox, Albert L. Lehninger (first): Lehninger Biochemie . 4th, completely revised u. exp. Edition. Springer, Berlin 2009, ISBN 978-3-540-68637-8 .
Web links
Individual evidence
- ↑ Gerd P. Püschel, Hartmut Kühn, Thomas Kietzmann, Wolfgang Höhne, Bruno Christ: Pocket Textbook Biochemistry . 1st edition. Georg Thieme Verlag, 2018, ISBN 9783132429031 , p. 252.
- ^ BH Robinson: Transport of phosphoenolpyruvate by the tricarboxylate transporting system in mammalian mitochondria. In: FEBS Lett . 14 (5), 1971, pp. 309-312. PMID 11945784 .
- ↑ S. Jitrapakdee, M. St. Maurice et al .: Structure, mechanism and regulation of pyruvate carboxylase. In: Biochem J . 413 (3), 2008, pp. 369-387. PMID 18613815 ; doi: 10.1042 / BJ20080709 .
- ^ H. Robert Horton, Laurence A. Moran, K. Gray Scrimgeour, Marc D. Perry, J. David Rawn, Carsten Biele (translator): Biochemie. 4th updated edition. Pearson Studium, 2008, ISBN 978-3-8273-7312-0 , p. 483.
- ↑ Jeremy M. Berg, John L. Tymoczko, Lubert Stryer: Biochemistry. 6 edition. Spektrum Akademischer Verlag, Heidelberg 2007, ISBN 978-3-8274-1800-5 , p. 518.