Erosion (geology)

The erosion (from latin erodere , nibble ') is a fundamental process in the exogenous portion of the rock circuit . It includes the removal of more or less strongly weathered rocks ( regolith ) or loose sediments including the soil (see → Soil erosion ). On the one hand, erosion creates terrain such as mountains and valleys, but ultimately destroys them completely if it continues to act, provided that it is not counteracted by any endogenous processes (especially tectonics) that cause the land surface to rise. Erosion is the opposite of sedimentation . The process that mediates between erosion and sedimentation is called transport (see → Hjulström diagram ).
In the case of erosion, a distinction is essentially made between linear (linear) and extensive erosion. Among their linear erosion is defined as the depression of the earth's surface by effluent water in small, short-lived rivulets ( rill ) or in streams (as then fluviatile or river erosion hereinafter). Another form of linear erosion, which is currently less widespread on earth, occurs through mountain glaciers ( exaration or glacial erosion). The resulting valley shapes are V-shaped ( Kerbtal ) with fluvial erosion and U-shaped ( trough valley ) with glacial erosion.
In contrast, extensive erosion occurs aeolian (through wind ), marine (through ocean surf and currents), glacial through inland ice and occasionally also directly through precipitation (see → rinsing ).
Linear and extensive erosion cannot be completely differentiated from one another and are dependent on the scale. Groove erosion has a linear effect on a small scale, but is flat on a large scale (especially in the case of soil erosion, for example). Also z. B. in ice ages or at particularly high latitudes, the linear erosion of mountain glaciers into the extensive erosion of inland ice glaciers. The large-scale removal and leveling of entire land surfaces is called denudation .
Physical basics

Natural erosion usually takes place with the participation of a flowing medium, whereby in the vast majority of cases it is either liquid water, ice or air in the form of wind. Since this medium transports the eroded material over more or less long distances (less than 100 meters to several 1000 kilometers), it is also called a transport medium . In the case of particularly steep reliefs, the material is transported solely by gravity, for example in the case of rock falls or when crawling on slopes .
The material of the subsoil, which was previously physically and chemically converted into loose material ( rock rubble , sand , soil) through weathering , is removed when the shear stress on the particles to be eroded is stronger than the static friction between the particles. The flow velocity required for this is higher, the lower the density of the medium, i. H. Erosion by wind requires higher flow velocities than erosion by water with the same erosion effect. An exception here is ice erosion. Although the density of ice is slightly lower than that of water, ice erosion occurs even at very low speeds. Rather, the size and thus the mass of an ice body, usually a glacier, are decisive for the effect of erosion. In addition, the movement of ice does not speak of "flowing", but of "flowing" or "creeping".
Eroded material that is already carried along by the flowing or flowing medium can significantly increase its erosion effect (see → Abrasives ).
The extent of the erosion, which is caused by the mechanisms mentioned above, also depends largely on the nature of the subsoil. The erosion effect is stronger, the lower the erosion resistance of the subsoil ( Petrovariance ). In particular, linear erosion starts where the subsoil has the lowest local resistance to erosion. This can be attributed to primary properties of the corresponding rock structure. The general rule here is that loose material is more easily erodible than solid rock , sand consequently more easily erodible than sandstone . Fine-grained material is easier to erode than coarse-grained material, and claystone is therefore easier to erode than sandstone. Igneous rocks such as basalt or granite are usually more difficult to erode than sedimentary rocks . Secondary modifications of the rock structure such as fissures or faults also offer preferred points of attack for erosion.
A dense vegetation cover has an adverse effect on erosion. Because there were hardly any land plants before the Devonian and virtually no land plants before the Silurian , it is assumed that the mean global erosion rate, i.e. H. the average rate at which the land surface was being eroded worldwide was significantly higher than it is today. The dependence of the erosion rate on the vegetation density is also known as phytovariance .
Forms of subaeric erosion
Under subaerial erosion , the removal of the uncovered from the sea continental land surface is understood. Depending on the eroding medium and its appearance, a distinction is made:
River erosion (fluvial erosion)

River erosion (fluvial erosion) , a linear form of erosion, is the creation of incisions in the land surface through the activity of flowing waters (streams, rivers).
The starting point for every fluvial erosion is a source outlet , from where the water, following the force of gravity , flows into deeper terrain. The erosion effect is particularly dependent on:
- the amount of water in the flowing water (not least depending on the climatic conditions)
- the water turbulence and entrained material
- the local terrain morphology (gradient) and, closely related to this, the flow velocity
- the height difference to the erosion base (height level below which its erosion effect is zero, usually this is the sea level)
The higher the water flow, the turbulence, the local gradient or the flow velocity and the difference in height to the erosion base, the stronger the erosion effect. A watercourse in a mountain range will cut a deep valley over the course of millennia far above the erosion base (typically a V-shaped, so-called notch valley ), even if its water flow is relatively small. The deepening of such a valley in the direction of the source, which inevitably takes place over time, is called retrograde erosion (see also → waterfall ). In special cases it can lead to flux tapping . On the other hand, a watercourse on level ground near the erosion base cannot cut itself deeply into the subsoil, even with high water flow.
The deepening of the river bed associated with the erosion effect of a river is known as deep erosion (or bed erosion , as the bed , the deepest area of the flow channel, is preferably eroded), its widening to the side as side erosion . In fast-flowing mountain rivers with steep slopes, deep erosion predominates. Side erosion predominates in sluggishly flowing rivers with a slight gradient, which then usually leads to the formation of meanders .
An ideal river has its source in the high mountains , then crosses a low plain and finally flows into the sea. The local gradient towards the mouth tends to decrease and with it the flow velocity. Can such flow initially rubble and gravel or crushed stone carry, it is in the mouth area usually only clay particles ( suspended load ). All material that can no longer be transported due to the decrease in flow velocity is deposited in the river bed on the way from the source to the mouth ( sedimentation ). In the event of flooding , deposits also take place outside the actual river bed. Depressions crossed by the upper reaches can act as local erosion bases with an increased sedimentation rate.
Over a given geological period, an area can experience multiple changes between erosion and sedimentation phases, caused either by tectonic uplift and subsidence processes of the earth's crust and their effect on the river gradient or by the increase or decrease in the absolute erosion base due to fluctuations in sea level . If more sediment is deposited than eroded, these periodic changes in the sediment sequence of the corresponding area are documented by the occurrence of erosion discordances . If more is eroded than sedimented, there is no sediment sequence for the period under consideration.
The erosion effect of a river can be subject to strong fluctuations in relatively short periods of time. This applies primarily to mountain rivers, which do most of their erosion work when they carry a multiple of their usual amount of water due to heavy or persistent rainfall.
Small forms of fluvial erosion, which can be traced back to turbulence in the flowing water, are pools and pools .
Anthropogenic influence on natural river erosion
The straightening and embankment of streams and rivers, the drainage and backfilling of floodplain areas, the additional discharge of water into flowing waters (e.g. through sewage treatment plants) or, in some cases, the artificial change of the bank vegetation lead to numerous flowing waters at least locally a change in the flow regime, which is primarily expressed in an increase in the flow velocity. This causes an increase in the depth or bed erosion rate and thus an accelerated deepening of the flow channel of the water bodies concerned (in this context the term bed erosion is also specifically defined as anthropogenically caused, accelerated deep erosion). The consequences are damage to the ecosystems associated with the respective waters, including a. due to the drying up of the remaining natural floodplain areas, which are increasingly above water level, as well as a greater risk of flooding in the regions on the lower reaches due to the faster draining floods of the upper reaches (see also → Anthropocene ).
Groove erosion
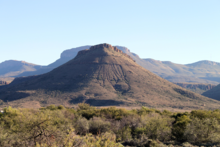
Groove erosion is a form of erosion that is linear on a small scale and extensive on a large scale, in which precipitation water flows superficially in small, short-lived rivulets ( rinsing channels ) down the slope, carrying material from the subsoil with it. Where the water collects, it usually washes out gullies (see also → Runse ). Often the discharged material flows together with the water through the channels into the nearest body of water. Groove erosion plays a role in widening valleys and leveling mountains. The removal of easily erodible material in the interaction of groove erosion and fluvial erosion and the associated formation of valleys in mountain regions is known as clearing .
Groove erosion is also an important mechanism in soil erosion.
A special erosion channel that only occurs in arid areas is the wadi (arroyo). The earth pyramids are a special form of advanced groove erosion in weakly solidified sediments .
So-called piping also occurs in weakly consolidated sediments and soils . This is not about superficial erosion, but about internal, “underground” erosion. Piping can also occur in earthworks such as dams and levees, which leads to the weakening and ultimately to the rupture of such a structure.
Glacier erosion (glacial erosion)
Glaciers form in areas with a correspondingly cold climate (high mountains, polar regions) . These move down into the valley just like the water of the rivers, but only a few meters per year, which leads to equally clear signs of erosion. In contrast to the mostly V-shaped river valleys (notch valleys), the glaciers create U-shaped valley cross-sections ( trough valleys ), the typical shape of which still suggests their glacial formation long after melting.
Glacier erosion is also primarily linear. During ice ages or in today's polar regions ( Antarctica , Greenland ), glaciers, combined to form ice sheets , cover huge areas, so that their erosion effect is flat there.
Abrasion (marine erosion)

This form of erosion attacks the mainland on a broad front and can be observed particularly well on cliffs . There, the work of the surf on the cliff leads to the formation of surf caves and other, similar cavities in the rock, which collapse over time. As a result, the coastline is pushed back inland and an ever-widening area is created at sea level, the abrasion plate (also: surf platform or rock chute).
Abrasion is linear to flat.
Wind erosion (aeolian erosion)
Wind has an erosive effect when it carries a lot of material (dust, sand) with it ( aeolian transport ), which then gnaws at the rock of the subsoil like a sandblasting fan (see e.g. → mushroom rocks ). This occurs preferentially in arid areas ( deserts ) with little vegetation and strong physical weathering. Wind erosion is complete.
In wind erosion, a distinction is made between deflation (denotes the blowing away of fine particles that have accumulated during weathering) and corrosion (denotes active erosion, i.e. the abrasive effect on rocks and stones by particles carried along).
Submarine erosion
In addition to all of the above-mentioned erosion processes that take place above sea level, erosion also takes place below sea level. The best known is the linear erosion of the continental slope by turbidity currents (see → underwater canyon ).
Erosion on other terrestrial planets
![]() |
![]() |
|
Landscape marked by wind erosion ( deflation level with stone paving ) in the Ahaggar region in Algeria (left) and on Chryse Planitia , Mars (right).
|
Wherever there are physical conditions similar to those on Earth, corresponding erosion processes can also take place on other planets. This is particularly well known from the neighboring planet Mars , where erosion channels have been observed that are clearly due to flowing water. However, there is no real fluvial erosion there, because the water currents that formed these channels were very short-lived due to the thin atmosphere of Mars.
Probably the only form of erosion currently active on both Earth and Mars is wind erosion, whereby the wind speeds that can generate sufficient shear stress for material transport must be many times higher on Mars than on Earth due to the low density of the atmosphere .
Bioerosion
Bioerosion is understood as the destructive effect of living organisms on hard substrates . Bioerosion thus differs fundamentally from abiogenic erosion. Primarily carbonate substrates in the sea are affected . The destruction takes place mechanically, e.g. B. by the feeding activity of parrot fish and sea urchins on hard corals , and / or chemically, such. B. through the drilling activity of the drilling sponge Cliona and the mussel Lithophaga in the skeletons of various marine invertebrates or in limestone cliffs in the intertidal zone (see also → Palichnology ). In the case of mechanical bioerosion, the substrate is crushed immediately; in the case of chemical bioerosion, it is only structurally weakened and thus promoted a subsequent abiogenic crushing and erosion.
Field studies have shown that the rate of bioerosion in modern coral reefs is approximately as high as their mean growth rate. In individual cases it is even significantly higher.
literature
- Hans-Rudolf Bork, Helga Bork, Claus Dalchow: Landscape development in Central Europe. Effects of humans on landscapes. Klett-Perthes, Gotha et al. 1998, ISBN 3-623-00849-4 .
- Roland Brinkmann : Outline of the geology. Volume 1: General Geology. 14th edition, revised. by Werner Zeil. Enke, Stuttgart 1990, ISBN 3-432-80594-2 .
- Christiane Martin, Manfred Eiblmaier (Ed.): Lexicon of Geosciences. 6 volumes. Spektrum, Akademischer Verlag, Heidelberg et al. 2000–2002, ISBN 3-8274-1655-8 .
- Andreas Heitkamp: Erosion and Weathering - Changing Landscape. In: Nadja Podbregar; Dieter Lohmann: In focus: geological knowledge. How does our planet work? Springer Verlag, Berlin / Heidelberg, 2013, e- ISBN 978-3-642-34791-7 , pp. 121-133.
- Hans Murawski : Geological Dictionary. 7th, revised and expanded edition. Enke, Stuttgart 1977, ISBN 3-432-84107-8 .
- Frank Press , Raymond Siever : General Geology. An introduction. Spectrum, Akademischer Verlag GmbH, Heidelberg et al. 1995, ISBN 3-86025-390-5 .
- Gerold Richter (Ed.): Soil erosion. Analysis and assessment of an environmental problem. Scientific Book Society, Darmstadt 2001, ISBN 3-534-12574-6 .
Web links
- Geological portrait weathering and erosion , mineral atlas
- Surface shapes on Mars
Individual evidence
- ↑ Klaus Kern: Sole erosion and floodplain landings. Recommendations for water maintenance. DVWK Non-profit training company for water management and landscape development. Mainz 1998, online (PDF; 17.7 MB)
- ^ V. Paul Wright: Reef dynamics In: Maurice E. Tucker, V. Paul Wright: Carbonate Sedimentology. Blackwell Scientific, Oxford 1990, ISBN 0-632-01472-5 , pp. 195 ff.
- ↑ TP Scoffin, CW Stearn, D. Boucher, P. Frydl, CM Hawkins, IG Hunter, JK MacGeachy: calcium carbonate budget of a fringing reef on the west coast of Barbados. Part II. Erosion, sediments and internal structure. Bulletin of Marine Science, Vol. 30, No. 2, 1980, pp. 475-508.
- ↑ Kelly Lee Acker, Michael J. Risk: Substrate Destruction and Sediment Production by the Boring Sponge Cliona caribbaea on Grand Cayman Island. Journal of Sedimentary Petrology. Vol. 55, No. 5, 1985, pp. 705-711, doi : 10.1306 / 212F87C4-2B24-11D7-8648000102C1865D