Protein misfolding disease
As protein misfolding diseases , including protein folding diseases ( Engl. Protein misfolding diseases or protein misfolding disorders or conformational diseases or proteopathies called), it refers to those diseases caused by incorrectly folded proteins caused inside and outside of cells. The misfolded proteins are either stored in the cells or broken down in the proteasome . In the first case, toxic deposits (plaques) are formed, in the second there is a loss of function due to a deficiency of the corresponding protein in the cell or in the entire organism . Both can become pathological for the person concerned over time and, depending on the protein concerned, lead to different diseases.
Biochemical mechanism
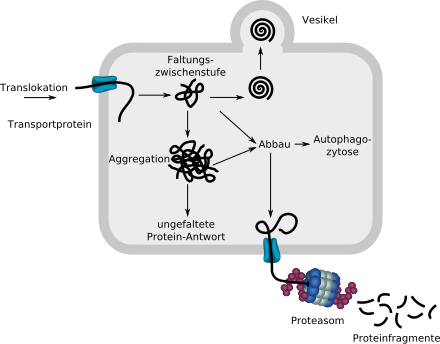
The linear, unfolded protein introduced into the ER via a transport protein begins to fold with the help of chaperones (not shown). If it is recognized as correctly folded by the protein quality control, it is removed from the ER via a vesicle. Misfolded proteins are smuggled into the cytosol via a transport protein and broken down into fragments in a proteasome. Amorphous aggregates can also be broken down by autophagocytosis .
If too many molecules are broken down due to incorrect folding, this can lead to a loss of function in the cell or the entire organism. If too many insoluble, no longer degradable aggregates are formed, deposits are toxic for the cell and the entire organism.
In most cells of all organisms , a wide variety of proteins are constantly produced in the course of protein synthesis, which fulfill a wide variety of functions in the cell and in the entire organism. For a protein to function correctly, its tertiary structure is of crucial importance. This structure is achieved through a process called protein folding. Protein folding is a complex and delicate process. Correct protein folding is monitored by the protein quality control. Statistically, around 30% of all proteins from protein biosynthesis are incorrectly folded and are normally broken down in the cell's proteasome within around ten minutes. The accumulation of incorrectly folded proteins in the endoplasmic reticulum leads to the unfolded protein response , a stress response of the cells that is associated with a suppression of translation and an increased synthesis of chaperones .
A single misfolded protein molecule is not responsible for the serious clinical pictures of protein misfolding diseases. To do this, large quantities of these proteins must be produced or the number of correctly folded molecules must be reduced. In the case of prions , this happens because a misfolded molecule on contact with a correctly folded molecule causes the correct one to unfold and ultimately incorrectly fold again. Since the first incorrectly folded protein is not changed by this process (it functions as an enzyme ), two incorrectly folded molecules are then present. These can fold further correct molecules.
The defective proteins are also called defective ribosomal products ( Engl. Defective ribosomal products , drips), respectively.
causes
The reasons for incorrect protein folding are complex. Gene mutations in exons that lead to changes in the amino acid sequence , i.e. the primary structure of the gene product , have a direct influence on the secondary and tertiary structure, or on the protein folding kinetics. Errors in transcription or translation can also lead to misfolding of the proteins. Another possible factor is the environment; In infectious prion diseases , for example, the prion protein is ingested with food or transferred using surgical instruments. There are now first indications of a toxin (BMAA) produced by cycads and cyanobacteria , which, when incorporated into proteins, leads to their misfolding and thus possibly to a form of ALS.
Gain-of-toxic function
If the DRiPs cannot be broken down in the proteasome, for example because they have previously aggregated to form aggregates, the DRiPs accumulate in the cell. There they can become pathological over time, that is, lead to specific diseases. The protein aggregates lead mainly to neurodegenerative diseases such as Parkinson's disease , Alzheimer's disease or Huntington 's disease . The aggregates have a new toxic function in the cells. The English term gain of (toxic) function is used for the toxic effect within the cells .
In the English-language specialist literature, the terms proteinopathy and proteopathy have become established for this form of protein misfolding disease . The corresponding German terms proteopathy and proteinopathy (the prefix proteo- = ' protein ' and the suffix -pathie = 'disease'), on the other hand, have barely established themselves in the German-language specialist literature.
Currently (as of 2011) over 100 proteinopathies are known in humans and animals. They are caused by the deposition of around 20 non-homologous proteins. The amyloidoses form a large and important group .
The protein misfolding diseases with a gain of toxic function include the following diseases:
Loss-of-physiological-function
Protein misfolding diseases also include diseases in which the misfolded proteins are broken down in the proteasome, as a result of which insufficient quantities of the protein are available to the cells or the organism. This loss of function, engl. loss of (physiological) function , can lead to diseases such as cystic fibrosis . Most patients with cystic fibrosis have a ΔF508 mutation ( deletion type ) in the CFTR protein - a chloride channel . The deletion of three nucleotides causes the amino acid phenylalanine (in the one -letter code F) to be missing at position 508 of CFTR . This mutation greatly changes the folding kinetics of the highly complex CFTR, which has 21 transmembrane protein domains , among other things . The folding process of the CFTR wild type takes more than two hours and only about 30% of the synthesized CFTR molecules fold fast enough to escape ER-associated protein degradation (ERAD). The ΔF508-CFTR folds even more poorly and is completely broken down, although in principle it would be fully functional as an ion channel. The patients affected by this mutation lack the chloride channel (= loss of function), which means that the composition of the secretions of various excretory glands is drastically changed.
A loss of physiological function occurs in the following diseases, among others:
illness | defective protein / gene | Remarks |
Cystic kidneys | Polycystin-1 | |
Charcot-Marie-Tooth disease | Aminoacyl-tRNA Synthetase (AARS) | |
X-linked lymphoproliferative syndrome | SH2D1A | |
Hirschsprung's disease | Receptor Tyrosine Kinase Ret | |
Homocystinuria and methylmalonic aciduria | MMACHC | |
Patellar hypoplasia | TBX4 | |
Sclerosteosis | Sclerostin | |
Cystic fibrosis | CFTR | |
Phenylketonuria | Phenylalanine hydroxylase | |
Hand-foot-genital syndrome | Homeobox protein A13 | |
lysosomal storage diseases | various lysosomal enzymes | over 40 individual diseases, u. a. Gaucher's disease , Fabry 's disease , Tay-Sachs syndrome, or Krabbe's disease |
QT syndrome | u. a. hERG | |
Angelman Syndrome | UBE3A | |
hereditary breast cancer | BRCA1 |
Gain-of-function and loss-of-function
In addition, there are protein misfolding diseases in which both a loss of function and the toxic protein deposits can become pathological. An example of this is alpha-1 antitrypsin deficiency . A mutation in SERPINA1 gene which codes for the acute-phase protein α-1-antitrypsin - a protease inhibitor - encoded , causes misfolding of α-1-antitrypsin. α-1-antitrypsin is mainly expressed by hepatocytes in the liver . Because of the misfolding, it cannot be secreted by the heptocytes and it forms intracellular deposits. The loss of function leads to progressive pulmonary emphysema in the affected patient , as the lack of α-1-antitrypsin means that the enzyme leukocyte elastase ( human leukocyte elastase , HLE) can destroy the lung structure unchecked. The deposits of α-1-antitrypsin in the hepatocytes lead to liver cirrhosis parallel to the pulmonary emphysema .
Treatment concepts
The protein misfolding diseases are currently not curable. There is as yet no causal or curative therapy for the most common neurodegenerative diseases caused by a gain of toxic function . Treatment of the patients is usually symptomatic or purely palliative . There are some future curative treatment concepts, such as gene therapy , which are still many years away from approval .
Protein misfolding diseases, which are caused by a loss of protein function, can in some cases be treated curatively. In enzyme replacement therapy , the missing protein, which is genetically engineered, is artificially infused into the patient . Chaperone therapies may be future treatment options for both types of protein misfolding disorders. Molecular chaperones are proteins whose most important task is to “help” newly synthesized proteins with their correct folding. In addition, “artificial” chemical and pharmacological chaperones were identified and developed that also support the folding process. The active ingredient sapropterin for the treatment of phenylketonuria is an example of an approved pharmacological chaperone. The imino sugar 1-deoxygalactonojirimycin (DGJ), international non- proprietary name Migalastat, is another pharmacological chaperone that is currently (as of October 2011) in clinical phase III for testing its effectiveness in patients with Fabry disease.
The especially green tea occurring epigallocatechin gallate (EGCG) is obviously to support able to correct folding of proteins. In in vitro experiments , EGCG was able to inhibit fibrillogenesis (the formation of fibrils ) of huntingtin, α-synuclein and β-amyloid. EGCG ensures that harmless spherical oligomers are formed instead of fibrous toxic fibrils . Obviously, it is also able to dissolve plaques that have already formed. In colored mice , the plaque load in the cortex, hippocampus and in the entorhinal cortex could each be reduced by about 50%.
Misfolding outside of cells
Since around 2008, it has been increasingly recognized that protein misfoldings not only lead to problems within cells, but also to a significant extent in the interstitial space . The importance of the glyphatic system (waste disposal of the central nervous system ) for the removal of misfolded proteins from the brain was discovered in 2012 and has been the subject of intensive research ever since. This applies in particular to all of the known and widespread neurodegenerative diseases .
further reading
Reference books
- M. Ramírez-Alvarado, JW Kelly, CM Dobson (Eds.): Protein Misfolding Diseases. Publisher John Wiley and Sons, 2010, ISBN 0-471-79928-9 limited preview in Google Book search
- J. Ovádi, F. Orosz (Eds.): Protein Folding and Misfolding: Neurodegenerative Diseases. Verlag Springer, 2009, ISBN 1-4020-9433-7 restricted preview in the Google book search
- HJ Smith, C. Simons, RDE Sewell: Protein misfolding in neurodegenerative diseases. CRC Press, 2008, ISBN 0-8493-7310-7
- VN Uversky, AL Fink (Ed.): Protein misfolding, aggregation and conformational diseases. Verlag Springer, 2007, ISBN 0-387-36529-X restricted preview in the Google book search
- RM Murphy, AM Tsai: Misbehaving proteins - protein (mis) folding, aggregation, and stability. Verlag Springer, 2006, ISBN 0-387-30508-4 limited preview in the Google book search
- P. Bross, N. Gregersen (Ed.): Protein misfolding and disease: principles and protocols. Humana Press, 2003, ISBN 1-58829-065-4 limited preview in Google Book Search
Review article
- KF Winklhofer, J. Tatzelt, C. Haass: The two faces of protein misfolding: gain- and loss-of-function in neurodegenerative diseases. In: The EMBO journal . Volume 27, Number 2, January 2008, pp. 336-349, ISSN 1460-2075 . doi : 10.1038 / sj.emboj.7601930 . PMID 18216876 . PMC 2234348 (free full text).
- H. Naiki, Y. Nagai: Molecular pathogenesis of protein misfolding diseases: pathological molecular environments versus quality control systems against misfolded proteins. In: Journal of biochemistry . Volume 146, Number 6, December 2009, pp. 751-756, ISSN 1756-2651 . doi : 10.1093 / jb / mvp119 . PMID 19643812 .
- LM Luheshi, CM Dobson : Bridging the gap: from protein misfolding to protein misfolding diseases. In: FEBS Letters . Volume 583, Number 16, August 2009, pp. 2581-2586, ISSN 1873-3468 . doi : 10.1016 / j.febslet.2009.06.030 . PMID 19545568 .
- M. Stoppini, L. Obici, et al. a .: Proteomics in protein misfolding diseases. In: Clinical chemistry and laboratory medicine. Volume 47, Number 6, 2009, pp. 627-635, ISSN 1434-6621 . doi : 10.1515 / CCLM.2009.164 . PMID 19527136 .
- H. Ecroyd, JA Carver: Unraveling the mysteries of protein folding and misfolding. In: IUBMB life. Volume 60, Number 12, December 2008, pp. 769-774, ISSN 1521-6551 . doi : 10.1002 / iub.117 . PMID 18767168 . (Review).
- GB Irvine, OM El-Agnaf et al. a .: Protein aggregation in the brain: the molecular basis for Alzheimer's and Parkinson's diseases. In: Molecular medicine (Cambridge, Mass.). Volume 14, Numbers 7-8, 2008 Jul-Aug, pp 451-464, ISSN 1076-1551 . doi : 10.2119 / 2007-00100.Irvine . PMID 18368143 . PMC 2274891 (free full text). (Review).
- E. Laskowska, E. Matuszewska, D. Kuczynska-Wisnik: Small heat shock proteins and protein-misfolding diseases. In: Current Pharmaceutical Biotechnology . Volume 11, Number 2, February 2010, pp. 146-157, ISSN 1873-4316 . PMID 20166966 .
- N. Gregersen: Protein misfolding disorders: pathogenesis and intervention. In: Journal of inherited metabolic disease. Volume 29, Numbers 2-3, 2006 Apr-Jun, pp. 456-470, ISSN 1573-2665 . doi : 10.1007 / s10545-006-0301-4 . PMID 16763918 . (Review).
- M. Stefani: Protein misfolding and aggregation: new examples in medicine and biology of the dark side of the protein world. In: Biochimica et biophysica acta . Volume 1739, Number 1, December 2004, pp. 5-25, ISSN 0006-3002 . doi : 10.1016 / j.bbadis.2004.08.004 . PMID 15607113 . (Review).
Individual evidence
- ↑ DM Cyr, DN Hebert: Protein quality control - linking the unfolded protein response to disease. Conference on 'From Unfolded Proteins in the Endoplasmic Reticulum to Disease'. In: EMBO Reports. Volume 10, Number 11, November 2009, pp. 1206-1210, ISSN 1469-3178 . doi : 10.1038 / embor.2009.224 . PMID 19851332 . PMC 2775177 (free full text).
- ↑ U. Schubert, LC Antón and a .: Rapid degradation of a large fraction of newly synthesized proteins by proteasomes. In: Nature . Volume 404, Number 6779, April 2000, pp. 770-774, ISSN 0028-0836 . doi : 10.1038 / 35008096 . PMID 10783891 .
- ↑ SB Qian, JR Bennink, JW Yewdell: Quantitating defective ribosome products. In: Methods in Molecular Biology. Volume 301, 2005, pp. 271-281, ISSN 1064-3745 . doi : 10.1385 / 1-59259-895-1: 271 . PMID 15917638 .
- ↑ JW Yewdell, LC Antón, JR Bennink: Defective ribosomal products (DRiPs): a major source of antigenic peptides for MHC class I molecules? In: Journal of Immunology. Volume 157, Number 5, September 1996, pp. 1823-1826, ISSN 0022-1767 . PMID 8757297 . (Review).
- ↑ Rachael Anne Dunlop, Paul Alan Cox et al. a .: The Non-Protein Amino Acid BMAA Is Misincorporated into Human Proteins in Place of l-Serine Causing Protein Misfolding and Aggregation. In: PLoS ONE. 8, 2013, p. E75376, doi : 10.1371 / journal.pone.0075376 .
- ^ KF Winklhofer, J. Tatzelt, C. Haass: The two faces of protein misfolding: gain and loss of function in neurodegenerative diseases. In: The EMBO Journal. Volume 27, Number 2, January 2008, pp. 336-349, ISSN 1460-2075 . doi : 10.1038 / sj.emboj.7601930 . PMID 18216876 . PMC 2234348 (free full text). (Review).
- ↑ R. Kisilevsky: amyloid: tombstones or triggers? In: Nature medicine . Volume 6, Number 6, June 2000, pp. 633-634, ISSN 1078-8956 . doi : 10.1038 / 76203 . PMID 10835676 . (Review).
- ↑ a b c Y. L. Lyubchenko, BH Kim u. a .: Nanoimaging for protein misfolding diseases. In: Wiley interdisciplinary reviews. Nanomedicine and nanobiotechnology. Volume 2, Number 5, 2010 Sep-Oct, pp. 526-543, ISSN 1939-0041 . doi : 10.1002 / wnan.102 . PMID 20665728 . (Review).
- ↑ L. Schöls, O. Riess, T. Schmidt: Autosomal dominant inherited spinocerebellar ataxias: Clinic, genetics and pathogenesis. In: Dtsch Arztebl. Volume 98, Number 23, 2001, pp. A-1546 / B-1319 / C-1233.
- ^ V. Tarlac, E. Storey: Role of proteolysis in polyglutamine disorders. In: Journal of Neuroscience Research. Volume 74, Number 3, November 2003, pp. 406-416, ISSN 0360-4012 . doi : 10.1002 / jnr.10746 . PMID 14598317 . (Review).
- ↑ M. Ramírez-Alvarado, JN Buxbaum: Systemic Amyloidose. In: M. Ramírez-Alvarado, JW Kelly, CM Dobson (Eds.): Protein Misfolding Diseases. John Wiley and Sons Publisher, 2010, ISBN 0-471-79928-9 , pp. 325-346. limited preview in Google Book search
- ^ VL Sim, B. Caughey: Prion Disease Therapy: Trials and Tribulations. In: M. Ramírez-Alvarado, JW Kelly, CM Dobson (Eds.): Protein Misfolding Diseases. John Wiley and Sons Publisher, 2010, ISBN 0-471-79928-9 , pp. 259-304. limited preview in Google Book search
- ↑ J. Wang, JA King: Cataract as a Protein-Aggregation Disease. In: M. Ramírez-Alvarado, JW Kelly, CM Dobson (Eds.): Protein Misfolding Diseases. John Wiley and Sons Publisher, 2010, ISBN 0-471-79928-9 , pp. 487-516. limited preview in Google Book search
- ↑ PB Stathopulos, YES Rumfeldt u. a .: Cu / Zn superoxide dismutase mutants associated with amyotrophic lateral sclerosis show enhanced formation of aggregates in vitro. In: Proceedings of the National Academy of Sciences . Volume 100, Number 12, June 2003, pp. 7021-7026, ISSN 0027-8424 . doi : 10.1073 / pnas.1237797100 . PMID 12773627 . PMC 165823 (free full text).
- ↑ L. Wang, KJ Colodner, MB Feany: Protein misfolding and oxidative stress promote glial-mediated neurodegeneration in an Alexander disease model. In: The Journal of Neuroscience. Volume 31, Number 8, February 2011, pp. 2868-2877, ISSN 1529-2401 . doi : 10.1523 / JNEUROSCI.3410-10.2011 . PMID 21414908 . PMC 3082397 (free full text).
- ↑ D. Hervé, H. Chabriat: CADASIL. In: Journal of geriatric psychiatry and neurology. Volume 23, Number 4, December 2010, pp. 269-276, ISSN 0891-9887 . doi : 10.1177 / 0891988710383570 . PMID 21045164 . (Review).
- ↑ AA Amato, RJ Barohn: Inclusion body myositis: old and new concepts. In: Journal of Neurology, Neurosurgery, and Psychiatry. Volume 80, Number 11, November 2009, pp. 1186-1193, ISSN 1468-330X . doi : 10.1136 / jnnp.2009.173823 . PMID 19864656 . (Review).
- ↑ L. Skora: High-resolution characterization of structural changes involved in prion diseases and dialysis-related amyloidosis. (PDF; 4.6 MB) Dissertation, Georg-August-Universität Göttingen, 2009, p. Iii.
- ^ PJ Waters: Degradation of mutant proteins, underlying "loss of function" phenotypes, plays a major role in genetic disease. (PDF; 537 kB) In: Current issues in molecular biology. Volume 3, Number 3, July 2001, pp. 57-65, ISSN 1467-3037 . PMID 11488412 . (Review).
- ^ A b D. N. Hebert, M. Molinari: In and out of the ER: protein folding, quality control, degradation, and related human diseases. In: Physiological reviews . Volume 87, Number 4, October 2007, pp. 1377-1408, ISSN 0031-9333 . doi : 10.1152 / physrev.00050.2006 . PMID 17928587 . (Review).
- ↑ RR Kopito: Biosynthesis and degradation of CFTR. In: Physiological reviews. Volume 79, Number 1, 1999, pp. S167-S173, ISSN 0031-9333 . PMID 9922380 . (Review).
- ↑ F. Melchior: Intracellular protein degradation. ( Memento of the original from May 23, 2014 in the Internet Archive ) Info: The archive link was inserted automatically and has not yet been checked. Please check the original and archive link according to the instructions and then remove this notice. (PDF; 3.5 MB) Center for Molecular Biology, WS 2009/2010, p. 30.
- ↑ L. Al-Bhalal, M. Akhtar: Molecular basis of autosomal dominant polycystic kidney disease. In: Advances in Anatomic Pathology. Volume 12, Number 3, May 2005, pp. 126-133, ISSN 1072-4109 . PMID 15900113 . (Review).
- ↑ HM McLaughlin, R. Sakaguchi et al. a .: A recurrent loss-of-function alanyl-tRNA synthetase (AARS) mutation in patients with charcot-marie-tooth disease type 2N (CMT2N). In: Human Mutation. [electronic publication before printing] October 2011, ISSN 1098-1004 . doi : 10.1002 / humu.21635 . PMID 22009580 .
- ↑ C. Li, C. Iosef et al. a .: Disease-causing SAP mutants are defective in ligand binding and protein folding. In: Biochemistry . Volume 42, Number 50, December 2003, pp. 14885-14892, ISSN 0006-2960 . doi : 10.1021 / bi034798l . PMID 14674764 .
- ↑ S. Kjaer, CF Ibáñez: Intrinsic susceptibility to misfolding of a hot-spot for Hirschsprung disease mutations in the ectodomain of RET. In: Human Molecular Genetics . Volume 12, Number 17, September 2003, pp. 2133-2144, ISSN 0964-6906 . doi : 10.1093 / hmg / ddg227 . PMID 12915470 .
- ↑ JP Lerner-Ellis, JC Tirone u. a .: Identification of the gene responsible for methylmalonic aciduria and homocystinuria, cblC type. In: Nature Genetics . Volume 38, Number 1, January 2006, pp. 93-100, ISSN 1061-4036 . doi : 10.1038 / ng1683 . PMID 16311595 .
- ↑ EM Bongers, PH Duijf u. a .: Mutations in the human TBX4 gene cause small patella syndrome. In: American Journal of Human Genetics . Volume 74, Number 6, June 2004, pp. 1239-1248, ISSN 0002-9297 . doi : 10.1086 / 421331 . PMID 15106123 . PMC 1182087 (free full text).
- ↑ E. Piters, C. Culha et al. a .: First missense mutation in the SOST gene causing sclerosteosis by loss of sclerostin function. In: Human Mutation. Volume 31, Number 7, July 2010, pp. E1526-E1543, ISSN 1098-1004 . doi : 10.1002 / humu.21274 . PMID 20583295 .
- ^ WE Balch, I. Braakman et al. a .: Folding Biology of Cystic Fibrosis: A Consortium-Based Approach to Disease. In: M. Ramírez-Alvarado, JW Kelly, CM Dobson (Eds.): Protein Misfolding Diseases. John Wiley and Sons, 2010, ISBN 0-471-79928-9 , pp. 403-424. limited preview in Google Book search
- ↑ DM Hutt, D. Herman u. a .: Reduced histone deacetylase 7 activity restores function to misfolded CFTR in cystic fibrosis. In: Nature Chemical Biology . Volume 6, Number 1, January 2010, pp. 25-33, ISSN 1552-4469 . doi : 10.1038 / nchembio.275 . PMID 19966789 . PMC 2901172 (free full text).
- ↑ SW Gersting, KF Kemter u. a .: Loss of function in phenylketonuria is caused by impaired molecular motions and conformational instability. In: American Journal of Human Genetics . Volume 83, Number 1, July 2008, pp. 5-17, ISSN 1537-6605 . doi : 10.1016 / j.ajhg.2008.05.013 . PMID 18538294 . PMC 2443833 (free full text).
- ↑ B. Utsch, CD McCabe et al. a .: Molecular characterization of HOXA13 polyalanine expansion proteins in hand-foot-genital syndrome. In: American Journal of Medical Genetics. Volume 143A, Number 24, December 2007, pp. 3161-3168, ISSN 1552-4833 . doi : 10.1002 / ajmg.a.31967 . PMID 17935235 .
- ↑ F. Wang, W. Song et al. a .: Inhibition of ER-associated degradation rescues native folding in loss of function protein misfolding diseases. In: The Journal of biological chemistry . [electronic publication before printing] October 2011, ISSN 1083-351X . doi : 10.1074 / jbc.M111.274332 . PMID 22006919 .
- ↑ T. Edmunds et al. a .: Gaucher Disease. In: M. Ramírez-Alvarado, JW Kelly, CM Dobson (Eds.): Protein Misfolding Diseases. John Wiley and Sons, 2010, ISBN 0-471-79928-9 , pp. 403-424. limited preview in Google Book search
- ↑ G. Hin-Fai Yam et al. a .: Fabry disease: on the molecular pathogenesis of a lysosomal storage disease and the use of a chemical chaperone for therapeutic intervention. ( Memento of February 20, 2007 in the Internet Archive ) University of Zurich, April 2, 2007
- ↑ F. Wang, W. Song et al. a .: Inhibition of ER-associated degradation rescues native folding in loss of function protein misfolding diseases. In: The Journal of biological chemistry. [electronic publication before printing] October 2011, ISSN 1083-351X . doi : 10.1074 / jbc.M111.274332 . PMID 22006919 .
- ↑ DA Wwnger: Krabbe disease. In: RA Pagon, TD Bird et al. a .: (Ed.): GeneReviews. PMID 20301416
- ^ VE Walker, MJ Wong et al. a .: Hsp40 chaperones promote degradation of the HERG potassium channel. In: The Journal of Biological Chemistry. Volume 285, Number 5, Jan 2010, pp. 3319-3329, ISSN 1083-351X . doi : 10.1074 / jbc.M109.024000 . PMID 19940115 . PMC 2823420 (free full text).
- ↑ RS Williams, DI Chasman et al. a .: Detection of protein folding defects caused by BRCA1-BRCT truncation and missense mutations. In: The Journal of Biological Chemistry. Volume 278, Number 52, December 2003, pp. 53007-53016, ISSN 0021-9258 . doi : 10.1074 / jbc.M310182200 . PMID 14534301 .
- ↑ DA Lomas, DH Perlmutter: Alpha-1-Antitrypsin Deficiency. In: M. Ramírez-Alvarado, JW Kelly, CM Dobson (Eds.): Protein Misfolding Diseases. John Wiley and Sons, 2010, ISBN 0-471-79928-9 , pp. 403-424. limited preview in Google Book search
- ↑ CM Greene, SD Miller et al. a .: Alpha-1 antitrypsin deficiency: a conformational disease associated with lung and liver manifestations. In: Journal of inherited metabolic disease. Volume 31, Number 1, February 2008, pp. 21-34, ISSN 1573-2665 . doi : 10.1007 / s10545-007-0748-y . PMID 18193338 . (Review).
- ^ FE Cohen, JW Kelly: Therapeutic approaches to protein-misfolding diseases. In: Nature. Volume 426, Number 6968, December 2003, pp. 905-909, ISSN 1476-4687 . doi : 10.1038 / nature02265 . PMID 14685252 . (Review).
- ↑ TK Chaudhuri, S. Paul: Protein-misfolding diseases and chaperone-based therapeutic approaches. In: The FEBS Journal . Volume 273, Number 7, April 2006, pp. 1331-1349, ISSN 1742-464X . doi : 10.1111 / j.1742-4658.2006.05181.x . PMID 16689923 . (Review).
- ↑ Clinical study (phase III): Study of the Effects of Oral AT1001 (Migalastat Hydrochloride) in Patients With Fabry Disease at Clinicaltrials.gov of the NIH
- ^ BE Roberts, J. Shorter: Escaping amyloid fate. In: Nature Structural & Molecular Biology . Volume 15, Number 6, June 2008, pp. 544-546, ISSN 1545-9985 . doi : 10.1038 / nsmb0608-544 . PMID 18523464 .
- ↑ DE Ehrnhoefer, M. Duennwald u. a .: Green tea (-) - epigallocatechin-gallate modulates early events in huntingtin misfolding and reduces toxicity in Huntington's disease models. In: Human Molecular Genetics . Volume 15, Number 18, September 2006, pp. 2743-2751, ISSN 0964-6906 . doi : 10.1093 / hmg / ddl210 . PMID 16893904 .
- ↑ a b D. E. Ehrnhoefer, J. Bieschke u. a .: EGCG redirects amyloidogenic polypeptides into unstructured, off-pathway oligomers. In: Nature structural & molecular biology. Volume 15, Number 6, June 2008, pp. 558-566, ISSN 1545-9985 . doi : 10.1038 / nsmb.1437 . PMID 18511942 .
- ↑ J. Bieschke, J. Russ u. a .: EGCG remodels mature alpha-synuclein and amyloid-beta fibrils and reduces cellular toxicity. In: Proceedings of the National Academy of Sciences . Volume 107, Number 17, April 2010, pp. 7710-7715, ISSN 1091-6490 . doi : 10.1073 / pnas.0910723107 . PMID 20385841 . PMC 2867908 (free full text).
- ↑ Substance EGCG in green tea prevents fatal plaque formation in Parkinson's and Alzheimer's - first results in the test tube. ( Memento of the original from April 7, 2012 in the Internet Archive ) Info: The archive link was inserted automatically and has not yet been checked. Please check the original and archive link according to the instructions and then remove this notice. Press release of the Max Delbrück Center for Molecular Medicine from May 30, 2008
- ↑ K. Rezai-Zadeh, GW Arendash et al. a .: Green tea epigallocatechin-3-gallate (EGCG) reduces beta-amyloid mediated cognitive impairment and modulates tau pathology in Alzheimer transgenic mice. In: Brain research. Volume 1214, June 2008, pp. 177-187, ISSN 0006-8993 . doi : 10.1016 / j.brainres.2008.02.107 . PMID 18457818 .
- ^ A. Schneider, M. Simons: Exosomes: vesicular carriers for intercellular communication in neurodegenerative disorders. In: Cell and tissue research. Volume 352, number 1, April 2013, pp. 33-47, doi : 10.1007 / s00441-012-1428-2 , PMID 22610588 , PMC 3602607 (free full text) (review).
- ^ NA Jessen, AS Munk, I. Lundgaard, M. Nedergaard: The Glymphatic System: A Beginner's Guide. In: Neurochemical research. Volume 40, number 12, December 2015, pp. 2583-2599, doi : 10.1007 / s11064-015-1581-6 , PMID 25947369 , PMC 4636982 (free full text) (review).
Web links
- L. Walker: Proteopathies: Protein Conformational Diseases. ( Memento from April 14, 2010 in the Internet Archive ) September 18, 2008