Solar thermal
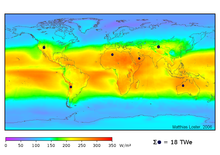
Under solar thermal (abbreviated to ST ) is defined as the conversion of solar energy into usable thermal energy . It is one of the renewable energies .
The radiation power hitting the earth's surface is around 165 W / m² on a daily average worldwide (based on 24 hours) (with considerable fluctuations depending on latitude, altitude and weather). The total amount of energy hitting the earth's surface is more than ten thousand times greater than the energy needs of mankind, the potential of solar energy is greater than that of all other renewable energies combined.
At the end of 2016, according to preliminary information, around 456 GW of solar thermal collectors were installed around the world for hot water production, which have an annual standard capacity of around 375 TWh of thermal energy, with around 325 GW a large part of it in China. In addition, there are solar thermal power plants for electricity generation with a total output of 4.8 GW.
history
The first applications of solar thermal utilization go up to the ancient (800 BC -... 600 AD.) Back when firing or concave mirror for focusing of light beams were used. The passive use of solar thermal energy was already practiced in ancient Egypt , Mesopotamia and in the early South American high cultures through the architecture of their buildings. Here, for example, doors were positioned in such a way that they were on the side facing away from the sun at lunchtime . In cold climate zones , windows and doors are preferably on the leeward side of the house, but if possible oriented towards the midday sun.
The architectural considerations of the Roman architect Vitruvius are documented . He wrote: "In the north, it seems, the buildings have to be provided with a flat vaulted ceiling as closed as possible and not open, but directed towards the warm regions of the sky."
In the 18th century, the naturalist Horace-Bénédict de Saussure invented the forerunners of today's solar panels. Triggered by the first oil crisis , useful concepts for using solar energy were developed in the mid-1970s.
The world's first patent for a solar system was granted in 1891 to the metal manufacturer Clarence M. Kemp from Baltimore. This was a simple heat collector for hot water.
The Olympic torch has been and is traditionally lit with a burning mirror since ancient times.
Theoretical foundations
Due to the nuclear fusion taking place in its interior, the sun generates a power of approx. 3.8 · 10 26 watts and emits it in the form of radiation. This radiant power is emitted approximately evenly in all directions from the spherical surface of the sun. Strictly speaking, the radiation output of the sun fluctuates both locally and temporally, see, for example, solar activity and space weather for more information. The earth orbits (actually on an elliptical orbit) at an average distance of about 150 million kilometers around the sun, this distance is also known as the astronomical unit . With regard to the radiation power, the earth moves on a spherical surface around the sun with a radius of one astronomical unit. The radiation power distributed over this spherical surface is on average
- ,
what is called the solar constant . The value fluctuates, so it is misleading to speak of a constant, but the term has become common. The solar constant allows simple calculations and estimates. While in near-earth space a surface oriented perpendicular to the sun actually receives the value given by the solar constant, the earth's atmosphere ensures significant damping. This value is considerably reduced by the atmosphere through reflection and absorption by clouds, aerosols and gases. Depending on the latitude , the radiation has to travel a longer path through the atmosphere (the extinction is proportional to the path length and density of the medium air, i.e. proportional to the mass of the air that the solar radiation traverses on its way to the earth's surface). This results in a different radiation power depending on the location on earth. From a clear midday sky in summer (higher position of the sun = shorter path through the atmosphere), the sun in Germany only shines with a maximum of 1,000 W / m 2 , in winter even with only 600 W / m 2 . There are weather stations with solar meters that allow you to observe the daily fluctuations. The solar radiation received on the earth's surface increases from sunrise to noon in a sine function, and then decreases again in the same way. At night the solar radiation is of course 0 W / m 2 , averaged over an entire year in Germany this results in about
Integrated over time , the solar radiation received results in usable solar energy . The radiated energy per year in Germany is around
- .
This corresponds to about half of the theoretically achievable value, which is mainly due to cloudiness. The Federal Statistical Office calculates that solar thermal collectors that are used for heating support will have an average annual yield of
or.
- .
This value is again significantly lower, as it takes into account other factors that also influence the yield in practice.
The targeted conversion of the incident solar radiation in the form of electromagnetic waves into thermal energy is basically carried out by the principle of absorption . This can be done by solar panels that directly absorb solar radiation. With concentrators based on the principle of reflection (concave mirror or a large number of individual mirrors tracking the sun), the sunlight can be focused , whereby an increased light intensity on the absorber and thus a higher temperature in the heat transfer medium can be achieved.
In the case of diffuse radiation from clouds or haze, thermal use of global radiation with concentrators is generally not possible. Flat-plate collectors can use the diffuse global radiation, which however typically has a lower output than direct sunlight.
Worldwide usage
Solar thermal energy is most widely used in China and Europe. In 2007, global capacity growth was 126 GW, 67% of which in China and 12% in Europe. In China, the energy is mostly used to generate hot water, in Europe it is often used for partially solar room heating. The worldwide installed capacity increased between the years 2007-2008 by 15% (19 GW, from 126 GW) to 145 GW. Between 2007 and 2008, European capacity increased by 21% (3.3 GW, from 15.7 GW) to 19 GW. The total European capacity rose to 26.3 GW even in the turbulent years 2008–2011, with some sharp drops in growth.
# | Country / Region | 2005 | 2006 | 2007 | 2008 | 2009 |
---|---|---|---|---|---|---|
1 |
![]() |
55.5 | 67.9 | 84.0 | 105.0 | |
2 |
![]() |
11.2 | 13.5 | 15.5 | 18.3 | |
3 |
![]() |
5.7 | 6.6 | 7.1 | 7.5 | |
4th |
![]() |
5.0 | 4.7 | 4.9 | 4.1 | |
5 |
![]() |
3.3 | 3.8 | 3.5 | 2.6 | |
6th |
![]() |
1.6 | 2.2 | 2.5 | 2.4 | |
7th |
![]() |
1.6 | 1.8 | 1.7 | 2.0 | |
8th |
![]() |
1.2 | 1.3 | 1.2 | 1.4 | |
9 |
![]() |
1.1 | 1.2 | 1.5 | 1.8 | |
world | 88 | 105 | 126 | 149 |
# | States | 2006 | 2007 | 2008 | Total 2008 |
---|---|---|---|---|---|
1 |
![]() |
1,050 | 665 | 1,470 | 7,766 |
2 |
![]() |
168 | 198 | 209 | 2,708 |
3 |
![]() |
205 | 197 | 243 | 2,268 |
4th |
![]() |
154 | 179 | 272 | 1,137 |
5 |
![]() |
130 | 172 | 295 | 1,124 |
6th |
![]() |
123 | 183 | 304 | 988 |
7th |
![]() |
42 | 46 | 48 | 485 |
8th |
![]() |
36 | 46 | 60 | 416 |
9 |
![]() |
18th | 16 | 23 | 293 |
10 |
![]() |
38 | 38 | 57 | 270 |
11 |
![]() |
10 | 14th | 18th | 254 |
12 |
![]() |
29 | 47 | 91 | 254 |
13 |
![]() |
14th | 18th | 60 | 223 |
14th |
![]() |
20th | 18th | 19th | 202 |
15th |
![]() |
25th | 30th | 64 | 188 |
16 |
![]() |
15th | 18th | 25th | 116 |
17th |
![]() |
5 | 8th | 11 | 96 |
18th |
![]() |
6th | 6th | 9 | 67 |
19th |
![]() |
0 | 0 | 6th | 66 |
20th |
![]() |
4th | 11 | 31 | 52 |
21st |
![]() |
3 | 4th | 4th | 25th |
22nd |
![]() |
2 | 2 | 3 | 22nd |
23 |
![]() |
2 | 3 | 3 | 18th |
24 |
![]() |
1 | 6th | 8th | 18th |
25th |
![]() |
2 | 2 | 3 | 16 |
26th |
![]() |
1 | 1 | 1 | 5 |
27 |
![]() |
0 | 0 | 1 | 3 |
28 |
![]() |
0 | 0 | 0 | 1 |
EU27 + Switzerland | 2,100 | 1,920 | 3,330 | 19,083 | |
* 1000 m² = 0.7 MW, air-filled collectors and vacuum tube collectors (Glazed Collectors) , 2004–2008 (kW) |
The world's largest solar system with 36,000 square meters of solar panels has been in Riyadh since the end of 2011 . It is intended to supply the campus of the Princess Nora bint Abdul Rahman University, which is currently under construction, with hot water for the 26,000 female students plus faculty .
Solar panels
Types of solar panels
A distinction is made between the following types of construction:
Solar thermal collectors without the concentration of radiation to raise the temperature
- Flat-plate collectors work at an average temperature of approx. 80 ° C. The light is not bundled in them, but directly heats a flat, heat-absorbing surface that conducts heat well and is criss-crossed with tubes in which the heat transfer medium is located. A water / propylene glycol mixture (ratio 60:40) is usually used as the heat transfer medium in these collectors . The addition of 40 percent propylene glycol provides frost protection down to −23 ° C and below freezing without increasing volume (to avoid possible frost cracking), as well as a boiling temperature that can be 150 ° C and more, depending on the pressure. There are now newer flat-plate collectors that are equipped with vacuum insulation instead of the insulating material (similar to vacuum tube collectors). This increases the efficiency through lower energy losses. The usable annual thermal energy that a non-vacuum-insulated flat-plate collector supplies is around 350 kWh / m².
- Evacuated tube collectors consist of two concentrically built glass tubes. Between these glass tubes there is a vacuum which the transmission of the radiation energy of the light to the absorber permits, but heat loss is greatly reduced. In the inner tube there is a heat transfer medium, usually a water- diethylene glycol mixture, which heats up and, driven by pumps, transports the heat. There are also so-called “open systems” that heat water directly. These collectors usually work up to an operating temperature of approx. 150 ° C. Evacuated tube collectors are more efficient than flat-plate collectors, but are typically expensive to purchase.
Solar thermal collectors with the concentration of radiation to raise the temperature
-
Evacuated tube collectors can also contain reflectors (see picture above) that concentrate the radiation on the tube with the heat transfer medium. But there are also tightly packed vacuum tube collectors without a reflector on the market. The concentration effect is, however, different in strength and should depending on the design
- on the one hand, cause less light to shine unused between the vacuum-insulated heat transfer pipes onto the roof tiles
- On the other hand, they allow the vacuum absorbers to be arranged at a greater clear distance, which saves costs and, due to the concentration of the radiation on the vacuum absorber, the temperature in the heat transfer medium rises and increases more quickly, which means that the minimum system temperature and thus the time at which the energy can be used is reached more quickly and the system becomes longer and more energetically usable because of the higher temperature.
- However, the disadvantage is that the reflectors get dirty and have to be carefully cleaned regularly, which is difficult to obtain the advantage.
- Parabolic trough collectors use the focusing of the light rays on a centrally running absorbing heat conduction. It should be noted here that the working temperature is significantly higher, between 200 and 500 ° C. Oils are therefore used as the heat transfer medium.
- Solar towers in which individual flat mirrors track the sun so that the light at the top of a tower is concentrated on the actual absorber. This process can generate very high temperatures of more than 1,000 ° C. The theoretical limit here is the sun's radiation temperature of around 5,500 ° C. Air, oils or liquid sodium are used as the heat transfer medium.
The goal is therefore a targeted absorption of as much as possible of all solar energy hitting the collector.
Problems are the reflections of the solar radiation in the absence of an anti-reflective coating. As a result, only part of the solar radiation reaches the absorber. Newer collectors use z. Partly anti-reflective special glasses, which reduce the reflected and thus unusable radiation.
Higher temperatures, which exceed the intended operating temperature, can possibly lead to thermal cracking of the antifreeze and thus to damage and permanent inoperability of the collector. Reaching such temperatures is avoided by a corresponding technical design of the collector itself and by integrating a correspondingly powerful circulating pump.
The quotient of the heat energy gained and the radiation energy arriving at the collector is the efficiency . With the current state of research, this is between 60 and 75% for home applications.
Areas of application in everyday life

In the private sector, solar thermal energy is primarily used for heating and air-conditioning buildings. In connection with good thermal insulation and the passive use of solar radiation, the need for additional heating energy is already greatly reduced. A well thought-out passive use of solar energy can also go so far in Central Europe that an active heating system is no longer necessary. The most typical examples of passive use of solar radiation are the greenhouse and winter garden . Roof overhangs over large double-glazed south-facing windows can have a cooling effect in summer, and in winter (due to the lower position of the sun) use the thermal radiation coming through the window to heat the room. A similar effect can be achieved with absorber wall surfaces , where sunlight hits a black absorber surface behind a transparent layer of insulation material and heats the wall behind. These passive techniques are used in so-called solar architecture . Since modern office buildings (for example the Commerzbank Tower in Frankfurt am Main and the Post Tower in Bonn ) often have an almost fully glazed exterior facade , there is an excess of solar heat in summer. This is where special glasses can help, which in summer block the thermal rays of the high midday sun, but are transparent for lower rays, such as occur in winter and also in summer outside of midday. Such special glasses can also be selectively controlled electrically. Often there is also an atrium with a fountain extending over several floors in order to maintain a cooling natural thermal.
In the context of building technology, collector-based solar thermal systems are classified as "active" technology due to the use of active, i.e. mostly electrically operated, circulation pumps within the heat cycle. However, passive use is also conceivable, for example for rooftop systems in frost-free climate zones that work according to the passive thermosiphon principle, or for collectors operated according to the same principle in small systems, for example to heat the water for outdoor showers.
Collectors can be used for hot water preparation, as an independent and fully-fledged heating system, or to support an existing other heating system. Any other type of additional heating is only required in old buildings where either the insulation is insufficient or the roof size is too small in relation to the volume of the room for the heat demand to be completely covered by collectors. Another reason can be permanent shading of the building or (in the case of a pitched roof) an unsuitable roof alignment. Orientation of the collectors to the south is ideal, whereby regional differences must be taken into account when installing the roof so that the system is not in the shade at any time of the day.
If these points are taken into account, another heating system can always be completely replaced. This is particularly desirable because of the better environmental and operational properties of solar thermal energy compared to other forms of heating, such as the various forms of CHP pellet heating . In this case, solar thermal and passive solar building technologies are less maintenance-sensitive and have significant advantages due to the lack of fuel. For the system operator, there are no running costs (apart from low electricity costs of around € 8 per year for the operation of the electric circulation pump with 7 W electrical power and a running time of 5,300 h / a, based on an average single-family house and a green electricity price of 21.5 ct / kWh). For the same reason, the plants and construction processes do not generate any additional traffic volume for society as a whole and do not compete with agriculture for valuable arable land, which would be the case with the widespread use of fuel-operated combined heat and power plants based on renewable raw materials. In addition, they generate neither fine dust emissions nor residual ash that has to be removed manually.
The storage of the thermal energy obtained in our latitudes, predominantly in summer, over long periods of time is ensured by thermochemical heat storage , in which the heat is chemically bound and released again with almost no seasonal loss, by buffer heat storage , such as water, or by latent heat storage , e.g. B. on the basis of paraffin, in which a large part of the thermal energy is stored in the phase transition from solid to liquid, and due to this, among other things, results in a heat capacity that is 1.5 times higher than that of simple water tanks. The use of seasonal storage tanks based on water or passive heat mass has the disadvantage that it takes up considerably more space to achieve the same heat capacity. This disadvantage can, however, be superfluous with a skilful design of new buildings (e.g. by thermal activation of the earth enclosed by the foundation), so that in particular passive heat mass or water can be desirable solutions for heat storage for reasons of cost .
When the sun's rays hit the collector, they typically transfer 60–75% of their energy to the heat transfer fluid, depending on the collector's absorption capacity. This is then pumped into the heat exchanger of the storage tank with a circulation pump . A controller (also known as a solar controller) connected to a temperature sensor starts the circulation pump as soon as the temperature of the heat transfer fluid in the collector exceeds a certain threshold value. The control settings affect the efficiency of the overall system and are dependent on the power consumption of the circulation pump and the pump output. When using a buffer storage tank, the control can be set up so that the pump runs when the temperature of the heat transfer fluid is above the temperature of the lower (which tends to be the coolest) buffer water in the storage tank. There it gives off its heat to the colder buffer water of the storage tank. The buffer water heats up as a result, rises in the storage tank, and can then be used via the heat exchanger to heat separate circuits for drinking and heating water, the drinking water circuit for showering, washing and the like. The like. Can serve more, so ultimately in the area of domestic use covers the complete need for heated "utility water".
Modern washing machines and dishwashers sometimes have separate hot water connections, through which solar thermal building technology can also contribute to improving the efficiency of household appliances by providing already heated drinking water .
The buffer store traditionally fulfills the function of time-shifted heat absorption and release. When using a thermochemical seasonal storage tank, from which heat can be extracted promptly and as required, the use of a buffer storage tank is no longer necessary. The seasonal storage tank can completely take over the function of the buffer storage tank (time-shifted heat absorption and release). Any latency times when starting the heat extraction can be absorbed by a very small internal buffer storage that is integrated into the seasonal storage. However, this can also be avoided from the outset by designing the device with a correspondingly low latency. The heat transfer fluid is fed directly to the seasonal storage tank to chemically bind the thermal energy, and on the heating side, the seasonal storage tank takes on the role of a flow heater. This eliminates the need for a separate buffer storage tank, which lowers acquisition costs and avoids ongoing heat losses that occur continuously with buffer storage tanks (despite insulation) in contrast to thermochemical heat storage tanks that are almost loss-free in the long term.
Alignment
Large solar thermal power plants are usually equipped with flexible tracking technology so that the question of alignment does not arise. In parabolic trough power plants , mostly single-axis tracking systems are used, in parabolic power plants and other horizontally tracking concentrator designs, typically two-axis. With statically mounted collector systems in the field of building technology, however, an optimal installation angle is essential in order to guarantee a high yield. A distinction is made between vertical inclination angle and, depending on the hemisphere, south or north deviation ( azimuth angle)
In Europe the best yields can be achieved with a collector inclination of 30 to 45 degrees and a direct orientation to the south (azimuth 0 °). A slight deviation from the south of up to 20 ° can still be accepted. Due to the following relationships, a deviation can even be advantageous in some cases: In the morning hours, the humidity is usually higher, which results in greater cloudiness of the air. It should also be borne in mind that the maximum air temperatures are usually reached between 1:00 p.m. and 3:00 p.m. The higher ambient temperature increases the efficiency of many systems . This may speak in favor of an alignment to the south-southwest . On the other hand, in many places there is increased cloud formation in the afternoon hours . This counteracts this or speaks in favor of an orientation to the south-south-east if the ambient conditions remain otherwise .
The optimal set-up angle should be calculated on a case-by-case basis using regional weather data. Various manufacturers offer corresponding simulation software for this purpose.
The installation angles of collector systems, which are primarily intended for heating, are traditionally often optimized for the greatest possible yield during the winter months, instead of the greatest possible annual total yield. This is done with the intention of immediately and completely covering the heating energy demand, which mainly arises in winter , without the integration of a seasonal storage tank, with the smallest possible and thus cost-optimized design of the collector. Here, the collectors are set up at about 40-45 °, so much steeper than it is z. B. would be the case with grid-connected photovoltaic systems, which are optimized at 30–35 ° for the highest possible annual total yield. With simple systems without a seasonal storage tank, the procedure enables the yield curve to be adapted to the temporal distribution of the heating energy requirement between summer and winter months, and with the same collector surface, more heating energy is directly available in winter, in order to compensate for a higher yield loss in summer. which is more likely to be accepted due to the very low heating energy requirement. Accordingly, for heating systems in which the heating energy demand occurs mainly during the summer and transition periods (e.g. when heating outdoor showers), the optimization of the irradiation during the transition periods can be useful, as the solar irradiation during the consumption period transition time-summer transition period during the transition periods on least is.
When integrating a seasonal storage tank into the system , static installation with a steeper installation angle is counterproductive from the point of view of the most favorable cost / benefit ratio, as the storage facility's ability to generate and release heat at different times delays the use of the highest solar radiation in summer during the winter, and the problem of the highest heating demand in winter is no longer a decisive factor in the design of the system. Instead, the solar radiation that is two to three times higher in summer outweighs the only slightly higher yield in winter due to a steeper installation angle.
On the other hand, flexible tracking of the collector is ideal (regardless of whether a seasonal storage tank is used) so that solar radiation is optimally used in both summer and winter. A two-axis tracking system can be installed on flat roofs, but on pitched roofs only one (mostly single-stage) adjustment of the vertical angle of inclination is possible anyway. In practice, such solutions are generally rarely used on buildings due to the mechanics' susceptibility to maintenance - often the advantage of easier handling and lower acquisition costs of statically mounted collectors predominates. Tracked collectors are also not suitable for in-roof installation, which is often used, especially in new buildings with a pitched roof.
An installation angle greater than 45 ° is generally not advisable, as this reduces the yield. The importance of the installation angle in the winter half-year> or <45 ° plays a rather minor role anyway, because the solar yield is much more limited by the dense clouds in Central Europe in the winter half-year. A flat installation angle, on the other hand, can lead to thermal surpluses in summer with the risk of system downtime. An angle of 90 ° in relation to the height of the sun on March 21 or September 23 is ideal.
The argument to avoid hail damage can possibly speak in favor of an installation angle greater than 45 ° if dangerous hail is to be expected in the region.
Energetic amortization time
The energetic amortization period of a solar thermal system is 12 to 24 months, i.e. H. During this time, the collectors fed the same amount of energy into the heating system that had to be used for production etc. of the system . The service life of the collectors is at least 30 years.
However, it has been criticized that the energetic amortization time is only partially meaningful with regard to the energetic overall efficiency. A short amortization period suggests high efficiency and a long amortization period low efficiency. The total energetic yield over the service life of a system is not taken into account. This can mean that a highly efficient system with a high energy production effort has a relatively late amortization time, but nevertheless has a significantly higher net yield over its entire service life than a system with a comparatively short amortization period.
economics
Goods solar thermal systems today in the 1980s and 1990s in Central Europe mathematically hardly represented and were mostly used by ecologically interested, then (2013) a solar system for heating oil prices by 90 cents per liter and the generated therefrom thermal energy of 10 ct / kWh most economic sense to operate. The amortization period of a solar thermal system depends, in addition to solar radiation, mainly on the durability of the components, since the system only pays for itself through the fuel energy saved. When using solar heat to support heating, it is essential to have a south-facing orientation, high-quality solar collectors and a good storage tank in layers, along with well-balanced hydraulics. Low flow temperatures increase the solar gain enormously.
In contrast to photovoltaics , German manufacturers of solar thermal systems are successful in the world markets and benefit from the enormous growth in solar heat generation in China.
Funding in Germany
Research and Development
In order to support the emerging renewable energy technology market in Germany, the Federal Ministry for the Environment, Nature Conservation and Nuclear Safety (BMU for short) has set up extensive funding measures. In the newly developing world market for solar thermal power plants, German companies have excellent opportunities thanks to the technologies developed with BMU funding. The first power plants in Nevada and Spain were built with significant German participation. In 2007 alone, the BMU approved a new funding volume of 5.9 million euros, in addition to the support of a further 5.9 million euros, which flow into ongoing projects. From 2008, the promotion of renewable energies in the heating market will be continued with a new focus. A total of up to EUR 350 million is available for the so-called market incentive program. That is significantly more than in previous years. As part of the federal government's integrated energy and climate program, the BMU has issued a new funding guideline for the market incentive program, which is to apply indefinitely from 2008. From 2009 onwards, up to 500 million euros will be available for the program. The funds are increased from the proceeds from the auctioning of emission certificates. A central aim of the funding according to these guidelines is to strengthen the sales of renewable energy technologies in the heating market through investment incentives and thus to contribute to reducing their costs and improving their profitability. As a result, from 2008 onwards, the newly introduced innovation funding for new or particularly innovative technologies in accordance with these guidelines will provide special incentives for market development.
Current usage
In general, the use of solar thermal systems is financially supported by the German state, depending on the size and area of application. With funding in the area of low-temperature solar thermal energy, the BMU aims to significantly increase the proportion of solar thermal energy used in heating and cooling. It should increasingly contribute to the substitution of fossil fuels and thus to a reduction in CO 2 . The plan is to achieve a tenfold increase in the installed thermal solar power by 2020. In order to be able to realize this, the funding measure: "Solarthermie2000plus" was launched. It is aimed at owners of correspondingly large existing or newly constructed buildings or properties for the integration of solar thermal systems into the construction plan.
Background: The energy policy in Germany aims at equally
- profitability for producers and consumers,
- the protection of the environment and resources, in particular the reduction of CO 2 emissions,
- the security of the energy supply.
In addition to state funding from KfW or BAFA, federal states, municipal utilities and municipal energy providers also offer funding for the installation of solar thermal systems . These are offered in the form of loans or grants and, in some cases, can also be combined. Often, the granting of loans or grants is linked to the implementation of further modernization measures.
Specific figures (as of BAFA 3/2013)
Solar collectors for combined hot water preparation and heating support
- In the case of an initial installation, BAFA ( Federal Office of Economics and Export Control ) subsidizes each laid ( or part thereof ) of up to 40 m² with 90 euros for combination systems. Pure drinking water systems are not funded in single and two-family houses.
- For the initial installation of solar collector systems of more than 20 m² to 100 m² gross collector area in apartment buildings with at least 3 units and in large non-residential buildings for combined hot water preparation and heating support, funding of 180 euros / m² gross collector area can be applied for.
- Process heat is supported with up to 50% of the net investment amount.
In addition, bonus grants are granted for the following measures:
- Boiler exchange bonus: The bonus of 500 euros is granted if, at the same time as installing a solar collector system for combined hot water preparation and heating support, an existing boiler without condensing technology is installed against a boiler with condensing technology (oil and gas).
- Regenerative combination bonus: simultaneous construction of an eligible biomass system or an eligible heat pump system. The bonus is 500 euros.
- Efficiency bonus: Plus 50% on the basic subsidy for the construction of an eligible solar collector system for combined hot water preparation and heating support or an eligible biomass system in a particularly well-insulated building. A building is considered to be particularly well insulated if the permissible transmission heat loss HT 'determined according to EnEV 2009 is undercut by at least 30%.
- Bonus for particularly efficient solar pumps (maximum 50 euros per pump).
Solar thermal power plants
→ Main article: Solar thermal power plant → See also: updraft power plant
In solar thermal power plants, solar energy is bundled on an absorber by mirror systems and the heat generated there is used to generate electricity with the help of conventional technology (e.g. steam turbines). Depending on the type of the focusing mirror system, a distinction is made between channel power plants, tower power plants and dish systems.
Solar tower power plant
A field of heliostats ( burning mirrors ) is arranged around a 50 to 150 meter high tower , which are computer-controlled and track the sun and bundle the reflected rays onto an absorber (“receiver”) attached to the top of the tower. A heat medium flows through this, which can be heated up to 1,000 ° C by the bundled solar energy. The generated thermal energy is used via a heat exchanger system to generate steam which, as in the already known power plant systems, drives a turbine coupled to a generator and thus generates electrical energy.
There are currently various technological approaches that are based on different heat transfer media (air, water or steam or molten salt) and receivers (tube bundle heat exchangers, atmospheric or pressure-charged volumetric structures).
In this way, relatively high power densities of ~ 37 W / m² mirror surface and around 25 W / m² floor space can be achieved in Spain. Based on a global radiation of 126 W / m² in Germany instead of the prevailing global radiation of 205 W / m² at the location in Spain, a yield of around 17 W / m² of floor space would be expected in Germany.
The currently largest solar tower power plant is the Ivanpah Solar Electric Generating System in the Mojave Desert in California / USA with a nominal output of 392 MW. In Germany, a test and demonstration facility was built in Jülich in cooperation with the Aachen University of Applied Sciences and put into operation at the end of 2008. The aim here is to research whether such a technology makes sense in Germany. The 60 meter high receiver converts the sun's rays reflected by 2,000 mirrors into 700 ° C to generate steam. The test facility, which cost 23.2 million euros, produces an electrical output of 1.5 MW.
Fall wind power plant
In contrast to the above Solar tower need Energy Tower no solar panels on the ground that concentrate the sun's energy to a certain point. With this principle, only a tall chimney is used, in the upper part of which water is sprayed. The evaporating water removes heat from the air, which cools down by around 12 ° C compared to the outside air and falls inside the chimney at speeds of up to 20 m / s. At the foot of the chimney, as with the solar tower KW, a turbine coupled with a generator is installed, which is driven by the artificially generated wind. The best and steadiest conditions for this type of power plant can be found in the area of the Rossbreiten , since dry and warm air is available here all year round. Due to the indirect use of the sun, the technology also works at night. Towers with a height of around 1,200 m and a diameter of 400 m should achieve outputs of up to 900 MW at suitable locations or provide a net output of approx. 600 MW for around 2/3 of the year. Fall wind power plants would then have an output and operating time comparable to conventional fossil and nuclear power plants. The efficiency reaches only around 2.5%, but is financially insignificant due to the "infinite" and free resource "warm air", but a larger area is required. About 1/3 of the gross electricity generated is required as pump energy to transport the water to be evaporated to the top of the chimney. Since the efficiency deteriorates significantly with smaller systems, this means that only large systems appear economically viable. Fall wind power plants currently only exist as a concept. A realization is being pushed ahead in Israel, but is currently failing due to a lack of financial resources.
Parabolic trough power plant
Here concave mirrors are used to concentrate the solar radiation on one point and thus to amplify it many times over. Mirrors with a parabolic cross-section are particularly suitable for this because they can also focus the edge radiation on the center. If the mirrors are constructed in the form of a channel, the solar radiation, concentrated around forty times, can be directed onto an absorber tube with a heat-conducting liquid. To increase the output, the parabolic troughs are arranged in a north-south direction and an adjustable longitudinal axis can be used to track the sun from east to west over the course of the day. The heat transfer fluid is heated up to 400 ° C in its circulating system and produces electricity via the turbine and generator . A well-known large-scale plant is the parabolic trough power plant in California's Mojave Desert. It has a total of 2.3 million square meters (2.3 km²) of mirror surface and generates 354 MW of electrical power. Similar large-scale plants are planned in Crete, Egypt and India, among others. So-called Fresnel mirror collectors are a further development of the parabolic troughs . With them, the sunlight is bundled onto an absorber tube via several parallel, non-curved mirror strips arranged on the ground (based on the principle of a Fresnel lens ). The strips are tracked uniaxially. An additional secondary mirror behind the tube directs the radiation onto the focal line. This concept is currently in the practical testing phase.
Paraboloid Power Plant (Dish)

In a parabolic power plant, a parabolic mirror that tracks the sun on two axes concentrates the solar energy directly on an absorber that is installed at the focal point of the mirror. The working gas (helium, air) in it heats up to 900 ° C and drives a Stirling engine or a turbine next to the absorber. The Stirling engine converts the thermal energy directly into mechanical work. Such systems achieve the highest levels of efficiency when converting sunlight into electrical energy. In an experiment in France with a parabolic mirror with a diameter of 8.5 m (area 56.7 m²) a net power of 9.2 kW was achieved, which corresponds to an efficiency of 16%. The modules are suitable for decentralized energy supply in remote regions and allow any number of these modules to be interconnected to form a large solar power plant. This can cover a power range of up to a few megawatts.
Solar pond power plants
In solar pond power plants, shallow salt lakes form a combination of solar collectors and heat storage . The water at the bottom is much more salty and therefore denser than at the surface. If solar radiation is absorbed in the deeper layers, these heat up to 85 to 90 ° C. Due to the density gradient due to the different salt content, the heated water cannot rise, there is no convection and the heat is stored in the lower water layer. The stored heat can be used to generate electricity in a turbine-generator block and is available 24 hours a day if configured accordingly.
In addition to the end product of electricity, there is also the option of using the thermal energy in solar chemistry . An important research result for the solar hydrogen economy is the recent thermal splitting of water vapor into hydrogen and oxygen at the DLR (see also hydrogen production ). With the help of a catalyst , the temperature required for this process was reduced from a few thousand degrees Celsius to below 1,400 ° C.
Solar district heating
In addition to the use of rooftop systems, there is also the option of installing solar collectors in the same way as solar parks in open-space systems, for example to supply local heating and district heating networks .
An early example is the Marstal heating network , which, starting in the 1990s, was expanded in several steps to a thermal output of a good 23 MW. In 2016, the most efficient system was in the Danish municipality of Silkeborg . With a collector area of approx. 157,000 m², this has a thermal output of 110 MW . An even larger system with an annual heat output of 230 GWh is in operation or in planning in Graz (as of 2016).
As of September 2016, Germany's largest solar thermal system using tube collectors is at Senftenberg . The system, consisting of 1680 collectors with a total area of approx. 8000 m², went into operation in August 2016. It should provide around 4 GWh of heat per year and cover the entire heat demand of the Senftenberg district heating network on summer days.
Solar cooling
Overview
Analogous to the generation of heating energy, solar thermal energy can also be used for cooling. This eliminates the need to convert primary energy into secondary energy (e.g. electrical energy), which simplifies the design of the cooling system and saves costs. Since the highest cooling requirement in typical applications often coincides with the time of the highest solar radiation , solar heat is ideally suited as driving energy for cooling systems even without intermediate storage. Night storage and seasonal heat storage also enable solar thermal cooling at times when there is no or too little solar radiation.
In principle, solar cooling can be used anytime and anywhere where solar thermal energy can be used technically. The prerequisite for this is the structural suitability of the building for the operation of solar collectors , i.e. H. No shading, suitable roof alignment and simply enough space on the roof. Whether solar cooling alone is sufficient, or whether it has to be supplemented by other processes, is ultimately decided only by the collector area that can be attached in relation to the cooling requirement.
Procedure
In general, there are a number of technical processes for converting heat into cold, i.e. ultimately the extraction of heat driven by heat. The graphic on the right is intended to clarify these relationships. Many processes can already be used in practice (marked in green in the graphic), others are still under development (marked in gray). The use of absorption chillers, which have been tried and tested for a long time, is particularly promising .
application
Solar cooling takes place e.g. B. Application in the following areas:
- solar air conditioning of buildings at high outside temperatures
- Cooling of food, beverages
- industrial process cooling, e.g. B. in the chemical industry
In the air conditioning of event rooms, machines, data centers and industrial companies, in which the operation itself generates a lot of heat, in addition to the heat from solar thermal energy, this internal heat can often be used for cooling at other points. So z. For example, in newer cars, the waste heat from the engine is used in conjunction with a thermoelectric element to recover electricity. In stationary applications, this same heat could be used to operate the air conditioning system. It should therefore be checked in each individual case whether other heat sources are already available whose heat energy can be used for cooling.
Since solar thermal systems in our latitudes can be used for heating in winter, it makes sense to use the same system in summer for cooling and in winter for heating. A correspondingly larger dimensioning of the collector system is sufficient for this, and an existing seasonal heat storage tank can then also be used in summer for intermediate storage for night-time operation of the cooling. This results in a cost advantage compared to the operation of heating and cooling with separate intermediate storage.
- The conversion stage to secondary energy (electrical current) is saved, which would be necessary with electrically operated cooling. This increases the efficiency and simplifies the system structure. The waiver of secondary energy intermediate stages goes hand in hand with a reduction in the requirements for the relevant infrastructure, such as power grids.
- In the area of solar building air conditioning, the primary energy is mainly available when the need for cooling is greatest, as solar radiation directly causes the need for air conditioning. Even refrigerators and cooling devices in the context of industrial processes must work against the increased heat at the time of the highest outside temperature and then consume correspondingly more energy, which can be made available immediately without further intermediate storage due to the high solar radiation.
- Solar cooling does not cause any electrical or magnetic fields and therefore, in contrast to electrical cooling systems, also no electrosmog. Therefore it is harmless to health in this regard.
Web links
- Research on solar thermal energy in Germany - Research Association for Renewable Energies
- Combination of condensing technology and solar thermal energy - article from IHKS-Fachjournal (edition 2008)
- Brochure "Solare Wärme" from the consumer centers
- E-book advice on solar thermal energy and information and tips on solar energy , free e-book from solaranlage-ratgeber.de.
- Christian Geinitz: "Where you can still earn money with solar energy" , report from June 14, 2013 in the FAZ about the current boom in solar thermal energy in China.
literature
- Thomas Delzer et al .: Solar heat for household use , A guide for choosing and buying your own solar system, Solarpraxis / Beuth, Berlin 2009, ISBN 978-3-934595-90-3 (Solarpraxis) / ISBN 978-3-410-20038 -3 (Beuth).
- Ursula Eicker : Solar Technologies for Buildings. Basics and practical examples , 2nd, completely revised edition, Vieweg + Teubner, Wiesbaden 2012, ISBN 978-3-8348-1281-0 .
- Martin Kaltschmitt , Wolfgang Streicher, Andreas Wiese (eds.): Renewable energies. System technology, economy, environmental aspects . Springer Vieweg, Berlin / Heidelberg 2013, ISBN 978-3-642-03248-6 .
- Bernd-Rainer Kasper, Bernhard Weyres-Borchert: Guide to solar thermal systems , 8th edition, German Society for Solar Energy , Munich 2008, ISBN 978-3-00-025562-5 .
- Klaus Oberzig: Solar heat. Heating with the sun. Stiftung Warentest, Berlin 2012, ISBN 978-3-86851-047-8 .
- Volker Quaschning : Renewable energies and climate protection. 4th edition. Hanser, Munich 2018, ISBN 978-3-446-45703-4 .
- Volker Quaschning: Regenerative Energy Systems. 9th edition, Hanser, Munich 2015, ISBN 978-3-446-44267-2 .
- Norbert Schreier et al .: Optimal use of solar heat . 17th, updated edition, Wagner, Cölbe 2007, ISBN 978-3-923129-36-2 .
- R. Stieglitz, V. Heinzel: Thermal solar energy: Fundamentals, technology, applications . Springer-Vieweg, Berlin / Heidelberg 2012, ISBN 978-3-642-29474-7 .
- Viktor Wesselak , Thomas Schabbach , Thomas Link, Joachim Fischer: Handbuch Regenerative Energietechnik . 3rd updated and expanded edition, Berlin / Heidelberg 2017, ISBN 978-3-662-53072-6 .
Individual evidence
- ↑ a b c Desertec Whitebook , Clean Power from Deserts (PDF document; 3.0 MB)
- ↑ Global Status Report 2017 . REN21 website . Retrieved July 26, 2017.
- ↑ Vitruvius. De architectura libri decem. Ten books on architecture. Translated and annotated by Curt Fensterbusch, 2nd revised edition, Verlag Wissenschaftliche Buchgesellschaft, Darmstadt 1976
- ↑ Global radiation in the Federal Republic of Germany, mean annual totals, period: 1981 - 2010. German Weather Service, accessed on April 18, 2016 .
- ↑ http://www.solarthermie.net/wissen/diffuse-strahl Diffuse radiation: Effects on the yield
- ^ Renewables Global Status Report: Energy Transformation Continues Despite Economic Slowdown. ( Memento of May 23, 2010 in the Internet Archive ) REN 21 Pariisi May 13, 2009.
- ↑ a b Solar thermal market grows strongly in Europe (PDF; 966 kB) Trends and Market Statistics 2008, ESTIF 5/2009
- ↑ Solar Thermal Markets in Europe - Trends and Market Statistics 2011 (PDF; 9.8 MB)
- ↑ Solar thermal markets in europe - trends and market statistics 2011. ( Memento of January 2, 2013 in the Internet Archive ) English-language page with tables and charts
- ↑ Renewables 2010. Globus status report. ( Memento of August 5, 2012 in the Internet Archive ) ren21.net, REN 21 Pariisi September 2010.
- ↑ The world's largest solar system comes from Carinthia , kaernten.orf.at, April 10, 2012.
- ↑ Solarturm-Jülich ( Memento from November 7, 2009 in the Internet Archive )
- ↑ Warm water from the sun tank . In: Main-Netz , July 5, 2013. Accessed May 10, 2014.
- ↑ http://www.energiesparen-im-haushalt.de/energie/tipps-zum-energiesparen/strom-sparen-im-haushalt/stromkonsum-pump.html
- ↑ http://www.heiz-tipp.de/ratgeber-564-neue_speicherarten.html
- ↑ http://solartechnikberater.de/technik_css/glossar2.cfm?id=161 ( Memento from February 20, 2007 in the Internet Archive )
- ↑ Ursula Eicker, Solar Technologies for Buildings. Basics and practical examples , 2nd completely revised edition, Wiesbaden 2012, p. 94.
- ↑ E. Strreicher, u. a .: "Methodology for determining the energetic amortization period of thermal solar systems" , University of Stuttgart, Institute for Thermodynamics and Thermal Engineering. 2002. ( Memento from July 15, 2014 in the Internet Archive )
- ^ V. Handke u. a .: "Feasibility studies for new environmental labels based on ISO 14024 for the product group: Thermal solar systems" . Final report on the UFOPLAN project 201 95 311/1. May 2003 on behalf of the Federal Environment Agency. ( Memento from October 26, 2007 in the Internet Archive )
- ↑ Volker Handke, Phillip Spitzmüller: “The amendment of the“ Blue Angel ”environmental label for solar thermal technology” 13th Symposium on Thermal Solar Energy 14. – 16. May 2003 Education Center of the Hanns Seidel Foundation, Banz Monastery, Bad Staffelstein Ed. Ostbayerische Technologie-Transfer-Institut eV (OTTI) Regensburg, ISBN 3-934681-26-3
- ↑ "Where you can still earn money with solar energy" report in the FAZ about solar thermal in China
- ↑ Overview of all current funding programs for solar thermal systems , provided by the non-profit co2online GmbH, accessed on July 25, 2014.
- ↑ Notes on the BAFA funding guidelines for the use of renewable energies
- ↑ Solar power plants for cloudy skies, FAZ June 14, 2010, p. 16
- ↑ California: World's largest solar power plant has started operations , Spiegel Online from February 15, 2014
- ↑ Pilot project in Jülich / Aachen, Germany ( Memento from April 27, 2008 in the Internet Archive )
- ↑ Viktor Wesselak , Thomas Schabbach , Thomas Link, Joachim Fischer: Handbuch Regenerative Energietechnik , Berlin / Heidelberg 2017, p. 419f.
- ↑ Database of large-scale solar systems. solar-district-heating.eu, archived from the original on December 14, 2017 ; Retrieved November 5, 2015 .
- ↑ Arcon-Sunmark builds the largest solar heating plant in the world . In: Sonne Wind & Wärme , October 13, 2016. Accessed October 19, 2016.
- ↑ Big Solar. The world's largest solar storage system is supposed to heat Grazern. In: Small newspaper . February 27, 2016. Retrieved July 15, 2016.
- ↑ Germany's largest vacuum tube solar system in operation . Solarify. Retrieved September 29, 2016.