Overlay Receiver
A heterodyne receiver (also superheterodyne receiver , or superhet or super ) is an electronic circuit for receiving and processing high-frequency electromagnetic radio signals . A characteristic feature is the conversion of the input frequency, which is variable depending on the desired reception frequency, to a mostly considerably lower and above all constant intermediate frequency (IF). The advantages are: The constant IF enables a permanently tuned IF amplifier; lowering the frequency reduces the requirements for the IF filter (larger relative bandwidth) and the generally lower frequencies to be processed. Furthermore, the risk of feedback is reduced, since there can no longer be an over-all feedback. Overlay receivers can be set up in several stages, i.e. they can have several intermediate frequencies (for details, see the relevant section ). It is used in many devices in radio transmission , telecommunications and HF measurement technology, from simple radio and television to GPS .
The term superposition is not to be understood in the sense of the superposition principle of physics , that is, an additive superposition; Rather, the principle can mathematically be traced back to a multiplication of the received input signal with a signal of constant frequency (from a - local - oscillator built into the receiver). The resulting difference and sum frequencies can be illustrated with the trigonometric addition theorems .
In recent years, not only auxiliary functions such as operation or LO frequency generation have been digitized in receiver technology, but increasingly larger parts of signal processing. This development led to the field of Software Defined Radio (SDR).
Basics
The first radio receivers were long-wave, straight-ahead receivers that demodulated the signal (often after amplification). The increasing number of transmitters forced a reduction in bandwidth so that several transmitters could not be received at the same time. The filter effort increases so drastically with increasing frequency that a different concept had to be invented: In the heterodyne receiver, the frequency of the RF signal is reduced at least once before it is demodulated. For this purpose, it is mixed with the signal of a so-called local oscillator (LO signal) located in the receiver in order to obtain a fixed, usually lower intermediate frequency (IF) with the same modulation content as the RF signal. The frequency of the local oscillator, together with the intermediate frequency, determines two reception frequencies, one of which can pass the input filter.
The necessary high amplification and filtering of the signal can only be achieved by reducing the frequency, which is usually considerable - the receiver becomes more sensitive and more selective. The signal filtering takes place at a constant and lower IF (intermediate frequency), which is why - in contrast to the tunable HF filter required for the straight-ahead receiver - fixed frequency filters can be used. This results in a simplified structure, significantly higher selectivity (selection) and thus a substantially improved reception quality. Receivers for very high frequencies such as radar or radio astronomy can only achieve good sensitivity in this way.
This reception principle can only be used sensibly up to about 10 10 Hz, because at even higher frequencies the strong phase noise of the mixer oscillator reduces the sensitivity too much.
The homodyne method , in which the LO and HF signals have approximately the same frequency, is not a heterodyne receiver with the features described . The amplitude-modulated received signal is converted directly (without IF) to the LF range; it is a direct receiver or direct mixer . Their main problem, the strong 1 / f noise , does not allow high sensitivity, which is why it is only used for mixing optical frequencies, where the strong phase noise of the oscillators makes heterodyne receivers based on the IF principle impossible.
history
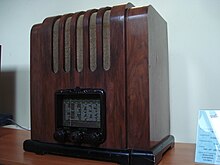
The name Heterodyne or Superheterodyne is a new word, composed of the Latin word super = "about" and the Greek words hetero = "different" and dynamis = "force", and describes the mixture of two signals of different frequencies. In contrast, the Greek word homόs = “equal” is used for the name of the homodyne recipient . The expression local oscillator means that this oscillator is at the location ( Latin locus = location), i.e. in the heterodyne receiver itself. Superhet or just super are common short forms for radio amateurs for heterodyne receivers .
Who is the inventor of the heterodyne receiver cannot be clearly determined. The fact that the invention fell during the First World War and that all warring parties involved were working on improving radio technology may have played a part in this. The English language Wikipedia names Edwin Armstrong as the inventor of the superheterodyne receiver , for which he received a patent in 1918.
Other sources indicate that Armstrong got the idea for this in 1918 while he was stationed in France. U.S. Patent No. 1,342,885 to Edwin Armstrong describes the principle of overlay. Armstrong filed for this patent in the United States in early 1919 and obtained it in mid-1920.
At almost the same time, Lucien Lévy (1917) in France and Walter Schottky (1918) in Germany are said to have developed this functional principle. Lucien Lévy received a patent in France in 1919 and 1920 (No. 493.660 and No. 506.297) for his circuit design that worked with an intermediate frequency (IF).
One of the first commercially built superhets was RCA's Radiola AR-812 , which sold over 140,000 times from 1924 to about 1927. The German company DeTeWe developed the "Ultradyn" in 1924/1925. In France in 1923 three home receivers are said to have been produced by Lucien Lévy's company "Radio LL".
In the decades that followed, the circuit principle became more and more popular due to its many advantages. Many variants of heterodyne receivers have been developed and built, some with double and multiple superimposition (up to fourfold) and mixing with a constant instead of a variable mixing frequency, so-called converters , such as e.g. B. the LNB in satellite technology .
The superposition is a universal method and is also used in transmitters . Practically all wireless transmitting and receiving devices available on the market today work according to the superimposition principle ( radio , two-way radio , mobile phone , base station , relay , television , satellite ).
Working principle
First, a filter limits the bandwidth of the antenna signal to a narrow range around the desired reception frequency. This reduces the voltage level of all signals that are generated by transmitters in other frequency ranges, which means that fewer undesirable mixing products are created and the input amplifier or mixer has to process lower voltage. In particular, the image frequency must be suppressed here. High-frequency amplifiers are necessary above the short-wave range so that weak signals are raised above the noise of the mixer, and they also prevent the LO frequency from being radiated via the antenna. Medium wave receivers almost never have high frequency preamplifiers.
The band-limited and possibly amplified antenna signal arrives at the mixer, where it is mixed with the signal from the tuning oscillator f LO , a series of new frequencies being generated. The LO frequency is selected by a fixed amount above or below the desired receive frequency. The mixed signal after the mixer contains, among other things, the sum and the difference of the input and LO frequencies, whereby the modulation of the input signal is retained. A band pass with constant frequency allows one of the mixed products to pass, only this is amplified in the intermediate frequency amplifier and then demodulated . The useful signal (i.e. speech or music during radio reception) is recovered from the IF signal .
Circuit stages in detail
Used abbreviations:
- = Pass frequency range of the preselection
- = Desired reception frequency
- = Intermediate frequency
- = Tuning frequency
- = Difference frequency (s)
- = Sum frequency (s)
RF amplifier / preselection
The high frequency amplifier has several functions:
- It adjusts the impedance of the antenna to the subsequent circuit (a maximum of the power absorbed by the antenna should be passed on to the subsequent stage).
- It amplifies the weak antenna signals so that they are above the noise level of the mixer. This increases the input sensitivity of the receiver.
- It prevents the LO frequency from reaching the antenna and being radiated there (jamming transmitter).
- In this stage, a preselection is made so that only frequencies from the reception range (passband ) can pass through the amplifier.
The preselection can either run with the input frequency (typical for tube technology) or in the form of mostly switchable bandpass filters. The preselection has several tasks:
- Suppression of reception on the image frequency .
- Reduction of the maximum HF voltage of the following active components, because the voltage components of all transmitters whose frequency is far enough away are suppressed. The linear working range of transistors etc. is limited and non-linear behavior would lead to mixing effects between the input signals. Such large-signal interference can generate ghost transmitters and cause an interference level that can mask the useful signal.
- Suppression of possible signals on the intermediate frequency that would get unhindered from the antenna to the IF amplifier with the mostly primitive, asymmetrical mixer circuits.
mixer
In the heterodyne receiver, the input frequency ( ) including its modulation is converted to another frequency by a mixer by mixing with the frequency of the tuning oscillator . With an ideal mixer , you only get two new sidebands at the output with signals on and , but real mixers generate a whole frequency spectrum.
With the heterodyne receiver, the difference frequency is almost always filtered from this. With simple receivers in long, medium and short wave, this is 455 kHz, which hardly allows image frequency suppression in the short wave range because the image frequency is only 910 kHz away. For VHF, the normal intermediate frequency is 10.7 MHz. In televisions, multiple superhets and cell phones, a significantly higher frequency is usually selected as the IF for technical reasons.
The oscillator frequency is generated by an oscillator circuit. Only in the early days was it generated by the mixer itself, because active components were still very expensive. The main disadvantage of these "self-oscillating" mixer stages is the modulation-dependent frequency change.
Since the mixers were actually only overdriven amplifiers, especially in the early days of reception technology, the IF breakdown is a major problem. With every asymmetrical mixer, an antenna signal on the IF reaches the IF amplifier unhindered and mostly still amplified (exception: diode mixer) and is treated by this as the desired difference frequency. Both signals, the down-mixed received signal and the “penetrating” signal from the transmitter on the IF, can be heard at the same time, and the interfering signal can even predominate. With AM, the two carrier frequencies in the demodulator also generate very annoying interference whistling. With FM, only the stronger of the two transmitters can be heard because of the FM threshold .
Since this highly undesirable side effect can only partially be eliminated by extremely good preselection and the use of symmetrical mixers, it was internationally agreed not to operate any transmitters at a sufficient distance from the usual IF frequencies of 455 kHz and 10.7 MHz . Nothing has changed in that regard so far.
With the (inexpensive) availability of multi-grid tubes and later of dual-gate field effect transistors , the multiplicative mixture spread. Here, the two voltages are each fed to a separate input of the control element, such as the two gates of a dual-gate field effect transistor or the control grid of a vacuum tube. The output signal is controlled by both input signals, which creates a mixing effect of the two control signals. At higher frequencies (in the higher GHz range) one often uses - and today (2006) still - a diode ring mixer.
The multiplicative mixing has slight circuit advantages over the additive mixing, so the repercussions on the RF amplifier are less and there is the possibility of an additional regulation of the mixer, which is rarely used. In addition, it produces fewer intermodulation frequencies and thus fewer “phantom reception points”.
Advantages and disadvantages of additive mixing
Advantages:
- The mixer transistor can also be used as an oscillator (self-oscillating mixer stage)
Disadvantage:
- Without a bridge circuit, the oscillator frequency and input frequency can hardly be decoupled
- Creates a lot of undesirable mixed products
Advantages and disadvantages of the multiplicative mix
Advantages:
- Generates fewer unwanted mixed products
- The oscillator frequency and the input frequency are decoupled
- A regulation of the mixing gain is possible
Disadvantage:
- With transistor technology, a self-oscillating multiplicative mixer stage is not possible, while in tube technology, for example, with an octode this has been implemented.
Tuning oscillator
The task of the tuning oscillator is to generate as constant a voltage as possible at the desired frequency with high accuracy. This frequency must be adjustable over a wide range so that it can be tuned to any desired transmitter within the reception range. There are several oscillator circuits suitable for this purpose. As a rule, LC resonant circuits are used to obtain a sinusoidal oscillator signal when it comes to tuning over a non- rasterized area. However, if the grid is clearly specified (e.g. VHF with 25 kHz) or CB radio, then LC oscillators are a bad choice and PLL oscillators take their place - they are far more accurate and cheaper, s. u.
The frequency stability (which means that a station can be received over a longer period of time without having to readjust the tuning manually) and the uniqueness of the scale (the same scale position of the frequency pointer should provide the same receive frequency) depends to a large extent on the tuning oscillator.
With an HF synthesizer and automatic frequency tracking ( AFC ), independence from temperature and aging effects can be achieved. (See VFO , VCO , PLL and DDS ).
When using an analog tuning oscillator, any frequency within the reception range can be set on the receiver. With digitally tuned oscillators, the input frequency can only be set with a certain step size. For simple shortwave devices, for example, these are usually 100 Hz or 1 kHz. High-quality DDS-controlled devices now offer tuning steps of 0.1 Hz, so that you can practically no longer perceive any difference to analog tuning.
Such fine increments are not necessary for broadcast bands with a fixed channel grid ( VHF , TV ). However, since not all transmitters adhere to the standard, good VHF receivers are manufactured with a step size in half the channel spacing (the 25 kHz mentioned above).
Intermediate frequency filter
The IF filter is a band pass for a narrow frequency range, which blocks signals outside this range, but lets the frequencies inside pass as unhindered and unchanged as possible. This means that only the desired frequencies from the frequency mix at the mixer output are passed on to the IF amplifier. The IF filter thus has a significant share in the selectivity of the receiver. Depending on the information bandwidth transmitted (e.g. voice or video signal) and the type of modulation, IF filters with different bandwidths are required.
In the early days of radio and broadcast technology (see the history of radio ), band filters made up of two supercritically coupled resonant circuits were used as IF filters. These were later supplemented by mechanical filters , quartz filters and ceramic filters ( ceramic resonators ). Acoustic surface wave filters (SAW filters) were later developed , with which it was possible to realize even complex transmission curves for high frequencies in the smallest of spaces. Increasingly, however, digital filters ( digital signal processor , DSP) are also used at high frequencies, so that the AOW filters are also becoming less important for cell phones.
The usual values for the IF frequency are 10.7 MHz (bandwidth approx. 50 kHz) for FM receivers for VHF broadcasting and 455 kHz (bandwidth approx. 5 ... 9 kHz) for AM receivers on long, medium and short wave . Analog TV receivers have an intermediate frequency of around 35 MHz and a filter bandwidth of around 5 MHz for the picture signal.
Intermediate frequency amplifier
The IF amplifier amplifies the signal and limits its amplitude with frequency modulation . FM can be limited by two anti-parallel diodes and is necessary because changes in amplitude, caused by interference on the transmission path, can worsen the quality of reception. In contrast to amplitude modulation, amplitude changes in FM do not transmit any information and can therefore be removed. For this reason, IF amplifiers for FM do not require any regulation.
The amplification of the individual stages in AM or SSB IF amplifiers, on the other hand, must be controllable in order to be able to process a large dynamic range. Otherwise the reception volumes of weak and very strong signals would differ too much.
Especially in tube and discrete transistor technology, the IF selection was not arranged as a compact unit in front of the IF amplifier. Instead, band filters, usually two magnetically coupled resonant circuits, were used to decouple the signal from the mixer or amplifier output and to adjust the power to the following amplifier input.
There are some receivers on the market that supplement one of the IF stages (usually the lowest frequency) with digital technology or replace it entirely. The analog signals that are fed to the IF stage are converted into digital signals in real time (see analog-digital converter ) and then processed by a signal processor . This has the advantage that many functions that are difficult or impossible to implement in hardware can be implemented in software . These include quality, among other things, in the bandwidth IF filter or variable notch filter (Engl. Notch filter ) which automatically follow the interfering frequency, to name just a few applications.
Demodulator
The demodulator separates the message content from the high- frequency carrier frequency . The demodulator circuits differ depending on the operating mode:
- The useful signal of amplitude-modulated transmissions is recovered with an envelope demodulator . This is basically a diode with a subsequent RC element as a low pass . In operating modes with suppressed carrier, such as SSB , the missing carrier frequency must be mixed in in a mixer - for example a ring modulator . This is generated in the receiver by a BFO ( Beat Frequency Oscillator ). In both cases, the control signals for the automatic gain control ( AGC ) in the demodulator are obtained from the demodulated voltage .
- Frequency-modulated signals are mostly demodulated by comparing the phase position of the signal with the phase position of a loosely coupled resonator. This resonator can be an oscillating circuit ( ratio discriminator or ratio detector), a ceramic resonator or a PLL circuit. If the resonance frequency and the useful signal frequency match, there is a 90 ° phase shift. If the useful signal frequency is lower, the phase angle decreases; if the useful frequency is higher, it increases. The voltage for the automatic frequency control ( AFC ) is also generated as a by-product .
- The numerous special forms of pulse modulation and in particular frequency spreading each require specially adapted demodulators that can only be implemented using digital signal processing .
NF amplifier
The audio amplifier raises the demodulated signals again to such an extent that a loudspeaker , headphones or external amplifier (hi-fi component) can be controlled. (Note: Traditionally, the connection for the amplifier on a radio was connected to the demodulator diode; hence the names “diode plug”, “cable” or “socket” for the corresponding connection components.) The audio amplifier can use the Affect sound properties , such as raising or lowering the highs and lows.
Automatic gain control
The automatic gain control , abbreviated to AVR in German ( automatic gain control , AGC), compensates for fluctuations in the received field strength . For this purpose, the control voltage that is obtained from the demodulator is fed to the HF / IF stages (reverse regulation) or the LF amplifier (forward regulation). There, the gain of the stage is increased or decreased accordingly. This makes it possible to reproduce weak and strong stations at the same volume or to compensate for the loss of shortwave reception.
Automatic frequency control
The automatic frequency control , abbreviated to AFR in German ( automatic frequency control , AFC), compensates for fluctuations in the reception frequency .
Consideration of the stages discussed so far on a circuit diagram
The VHF tuner shown in the picture has a controllable HF amplifier (yellow), a multiplicative mixer (green) and a VCO (red). The tuner is equipped with dual-gate FETs, which are characterized by high input resistance and low inherent noise. The main advantage over other designs is that these components contain two transistors in the form of a cascode circuit , which is why the capacitive feedback from the output (drain) to gate 1 is so low that the amplifier works stably even without neutralization.
RF amplifier / preselection
In order to transfer as much received energy as possible from the 75 ohm coaxial cable to the first resonant circuit, the antenna impedance is coupled to the RF amplifier stage via the transformer L1 / L2 . L2, C2, C3, D1, D2 form the first pre- circuit (parallel resonant circuit ), the frequency of which can be tuned via the capacitance diodes D1, D2. The necessary tuning voltage comes from the series resistor R8. C3 is used to adjust the first pre-circuit (intended for the manufacturer or service technician).
The pre-selected input frequency is sent via C4 to gate 1 (G1) of Q1, which amplifies the input frequency. Its gain can be changed via gate 2 (G2). C7 ensures that G2 is "earthed" in terms of AC voltage (ie connected to zero potential), because this is the only way to secure the internal shielding between input and output. The amplified input frequency is inductively transferred to the next pre-circuit, which forms another parallel resonant circuit with C9, C10, D3, D4 and can be tuned by D3, D4.
Mixer and first IF filter
The input frequency is taken via a tap (inductive voltage divider) from L4 via C11 to G1 of Q2. Since the conductance of the upper transistor of the cascode circuit Q2 can be changed by the oscillator voltage, mixing takes place in which the intermediate frequency - usually 10.7 MHz - is also produced. This is filtered by the first IF bandpass and passed on via connections 5 and 6 to the following intermediate frequency amplifier.
Tuning Oscillator (VCO)
The oscillator transistor Q3 operates in common base . The operating voltage reaches the collector of the transistor via R25, L5, R23. C26, C25 are used to block the operating voltage and are not frequency-determining for the oscillator circuit L5, C24, D6, D7, as they only represent a short circuit at this frequency (C26 = C25 = 560 pF). C22 creates positive feedback to make the oscillator oscillate. Together with C20, the phase condition of 0 ° is fulfilled in this oscillator circuit.
R26, R21 and D5 form the base voltage divider, with D5 being used for temperature compensation. This is necessary so that the oscillator frequency changes only slightly with temperature fluctuations.
C19 eliminates HF interference on the operating voltage. The same applies to C16, C17 for the tuning voltage. The AFC input leads to a capacitance diode, which can change the oscillator frequency by a few kilohertz so that the intermediate frequency 10.7 MHz is maintained. Only then do the bandpasses produce the least amount of distortion in the modulation content.
The vote
As already mentioned in the explanation of the tuning oscillator, its frequency can be set by the user. Possible reception frequencies are always higher or lower than the frequency of the tuning oscillator by the amount of the IF frequency :
- with upmixing:
- with downward mixing:
One of these is desirable, the other is called the image frequency and must be suppressed by band filters in front of the mixer or by the IQ method .
If, for example, a station from the frequency range from 800 to 1200 kHz is required, you can set it to 1455 kHz. Then these frequencies and their sums and differences are available at the output of the mixer . The IF filter only allows 455 kHz through. The only frequency in the range from that can meet this condition is the receiving frequency of 1000 kHz. An addition of the input frequency with the tuning frequency is always ≥2255 kHz; so there is only the difference:
In practice, it is not only this single frequency that is allowed to pass through the filter, as the sidebands containing the modulation are cut off in this way. A suitable bandwidth of the IF filter of, for example, 10 kHz (which then results in the usable bandwidth of the useful audio signal) is selected in order to allow all frequencies between approximately 450 and 460 kHz to pass. This corresponds to the total signal of an amplitude-modulated medium wave transmitter, which occupies a range between 995 and 1005 kHz.
In the example above, only 800 to 1200 kHz was allowed. Removing this preselection reveals a disadvantage of the superhet and the need to limit the reception bandwidth in the RF preamplifier (or in front of the mixer) using a filter.
If even higher reception frequencies could reach the mixer, there would be a difference frequency from and , which results in 455 kHz:
- .
In addition to the desired reception frequency of 1000 kHz, the frequency 1910 kHz would also be mixed down to the IF, passed into the IF amplifier and demodulated. This second, unwanted reception frequency is called the image frequency. It is mirrored with the interval between the IF frequency and the tuning frequency.
For receivers with insufficient image frequency suppression, each transmitter is received twice (if the frequency of the tuning oscillator can be changed far enough): once on the actual transmission frequency and a second time as the image frequency of this transmitter on the frequency . This is unpleasant, but often not very annoying. The problem of the mirror-frequency reception, when the reception frequency and the image frequency is occupied by a transmitter, which is very common in high band occupancy. Then both transmitters are demodulated at the same time and audible interference occurs.
Advantages and disadvantages
Advantages:
- The superhet is the best way of stable processing of very high reception frequencies, such as those that occur with FM or satellite reception. A straight-ahead receiver is unsuitable for this because it has too little gain and too large a bandwidth. A direct mix receiver is too insensitive because of its high 1 / f noise .
- Frequency modulation can only be demodulated well if the ratio of frequency deviation / frequency is as large as possible. Therefore, the circuit is simplified considerably by reducing the frequency.
- The IF filter is set to a fixed frequency, which is usually lower than the receiving frequency. It is therefore easier to design the filter with a higher quality factor .
- The IF amplifier can be set up electrically more stable at low frequencies than at higher frequencies. A high overall gain can be achieved without the risk of feedback and with a few amplifier stages, since the signal is amplified at different frequencies.
- This high overall gain is specifically reduced ( automatic gain control ) so that approximately the same signal strength is measured at the output, even if the antenna voltage changes by several orders of magnitude. See also shrinkage compensation .
- A filter that works directly on the receiving frequency must be tunable (the frequency can be changed) so that different frequencies (transmitters) can be received. High-quality, narrow-band, tunable filters are difficult to implement at high frequencies; they also change their bandwidth with the reception frequency.
- A filter with a fixed, low frequency increases the production-related reproducibility of the receiver quite decisively compared to other concepts such as the straight-ahead receiver or the audion . For a large part of the complex adjustment work that is required for a multi-circuit audion, a single setting during production is sufficient for the Superhet.
- If surface acoustic wave filters (in ancient devices: several band filters) are used, an almost rectangular transmission curve is achieved, which allows a high degree of selectivity through high edge steepness without clipping the high frequencies.
- In contrast to the straight-ahead receiver, the oscillator frequency can be set and stabilized digitally with a PLL , for example . This means that the receiving frequency can also be controlled remotely.
- Finally, the ease of use ("one-button operation") that the superimposition principle entails should be mentioned.
Disadvantage:
- The superimposition principle creates a secondary receiving point (image frequency), which can be suppressed either through increased filter effort in front of the mixer or with special circuits such as the phase method (IQ method).
- Using a mixer that is too simple can lead to undesirable by-products and “whistling points” due to intermodulation .
- The background noise of the receiver is increased by the additional tuning oscillator and the mixer compared to a straight-ahead receiver. At very high frequencies above about 5 GHz, the phase noise of the oscillator is so high that the sensitivity of a heterodyne receiver is noticeably reduced. With tricky circuits, this frequency limit can be stretched to around 50 GHz in special cases.
- With optical frequencies ( 10-14 Hz) a heterodyne receiver can no longer be built because no known oscillator ( laser ) has the necessary frequency accuracy and constancy. In this area, insensitive direct mix receivers are necessarily used.
- Parts of the local oscillator signal are transmitted via the receiving antenna and thus allow the receiving system to be located by other receivers with directional antenna , provided the frequency is known. However, this disadvantage is largely insignificant for civil use. However, in extreme cases, it can also interfere with other receivers.
Circuit variants
At high frequencies, single superposition has the disadvantage that at a low intermediate frequency (455 kHz) the image frequency can hardly be separated from the desired reception frequency. If you choose a high intermediate frequency (10.7 MHz), the bandwidth of the IF filters also increases significantly. For this reason and in response to special requirements, variants of the heterodyne receiver have been developed.
Double and multiple overlay receivers
With single superhet, the selected intermediate frequency is always a compromise. On the one hand, it should be as low as possible, because the IF filter can be built with steep edges and a high quality factor for low frequencies . On the other hand, a low IF exacerbates the problem of the image frequency. The lower the IF frequency, the smaller the distance between the receiving frequency and a signal on the image frequency (distance = ).
A low IF therefore requires a narrow-band preselection in order to effectively suppress the image frequency. This becomes more and more difficult the higher the reception frequencies are, since the filter in the preselection must have a higher quality with the same bandwidth.

To circumvent this problem, the double conversion works with two intermediate frequencies. In shortwave and amateur radio receivers, the first IF is often selected in the range from 40 to 70 MHz and the second IF is 455 kHz or lower. The tuning oscillator ( VCO in Figure 1) oscillates here by the 1st IF center frequency higher than .
Due to the high first IF, the image frequencies are very far from the received usable frequency in the range of 40 ... 100 MHz. In principle, a 30 MHz low pass was sufficient as a preselection in the RF pre-stage. In most cases, however, these receivers have several switchable bandpass filters to enable as many powerful transmitters as possible, e.g. B. in the medium or low shortwave range to hide.
At this high first IF, crystal filters are expensive and have limited selection. This is why the same filter (“roofing filter”) with typically 12 kHz usable bandwidth is used for all operating modes and converted to a significantly lower second intermediate frequency with a second oscillator signal (crystal oscillator in Fig. 1). The further selection can then, as with a single super, on z. B. 455 kHz can be realized. The roofing filter greatly narrows the frequency window that the other stages have to process and thus keeps a large number of strong external signals away. Weak point: If an unwanted transmitter is close enough that its signal can be passed through by the wide crystal filter, but not by the narrower filter of the 2nd IF, the second mixer can be overdriven.
A variant that is now popular is to use a significantly lower 2nd (or 3rd) IF frequency, digitize this IF signal with an analog-to-digital converter and process it digitally - including demodulation.
It is possible to make more than one oscillator tunable. This principle is used, for example, in the Barlow Wadley XCR-30 shortwave receiver . With this receiver, the desired input signal is mixed up into the first IF range of 44.5–45.5 MHz with an adjustable oscillator. This first oscillator is used to select the MHz range. The first IF is then mixed with an oscillator signal of a constant 42.5 MHz into the second IF range between 2 and 3 MHz. The desired reception frequency in the kilohertz range is then set from the second IF using a normal single superheating circuit and mixed down to the third IF of 455 kHz. This principle requires two tuning processes: the selection of the MHz frequency range with the first tuning wheel (MHz SET) and then the selection of the receiving frequency within this MHz section with a second tuning wheel (kHz SET).
The advantages of this circuit are a good reading and repeat accuracy for an analog receiver and a very high image frequency suppression. This works without a PLL, i.e. without the associated potential high-frequency interference sources, but it suffers from poor large-signal behavior. Since the selection only takes place in the fifth stage, the previous stages can be overridden by neighboring stations without being able to hear these stations.
Converter, frequency converter
Converters or frequency converters are ballasts that convert one frequency range to another. They are mostly used to "open up" new frequency ranges to existing devices. For this purpose, the frequency range to be received is mixed with a constant frequency in the first mixer and a whole frequency band is shifted to another frequency range. Within this frequency range, a single or multiple super is then tuned to the desired station.
One example is the LNB in satellite technology. This reduces the reception frequency from around 10.7–12.7 GHz to around 1–2 GHz and sends this first intermediate frequency to the satellite receiver via a longer cable . Here the first IF filter is not a fixed frequency filter as in a conventional receiver, but the satellite receiver is itself a superhet that converts the frequency range coming from the LNB (usually 950 to 2150 MHz) to 480 MHz.
Frequency converters are still used when converting the 70 cm amateur radio band to the 2 m amateur radio band (historical) and when converting UHF transmitters to the VHF band (historical). For older TV sets there are converters that convert the frequency range of the special cable channels into the UHF range, and for car radios there were converters that relocated parts of the HF bands to the MW range.
Measuring receiver
Similar to a spectrum analyzer , a measuring receiver is used to determine the magnitude spectrum of an electromagnetic signal . The principle used is not unlike that of a spectrum analyzer. The demodulation takes place here with the detectors with which the signal levels are evaluated. However, before the signal is mixed, the HF signal is preselected. A measuring receiver does not sweep continuously over a frequency range ( span ) like the analyzer , but rather discrete frequencies are selected at which the level is to be measured.
As a counterpart to the analyzer's “frequency sweep”, modern test receivers have a “frequency scan” (which is often called a “sweep”). Here, in a certain frequency range for a defined time is measured at a long frequency before the device an automatic step (engl. Step executes) to the next measurement frequency and measured again. The step size depends on the respective resolution bandwidth, which in turn is prescribed in standards. The measurement time or dwell time is to be selected depending on the signal to be measured. In the case of narrow-band signals, the time can be selected to be comparatively small; in the case of periodically occurring transient (interference) signals, however, the measurement time must be adapted to the repetition frequency.
In modern test receivers, the IF filtering and the detectors are partially or completely digital. Requirements for measuring receivers and their detectors are specified internationally in CISPR 16-1-1.
Today there arise more and more processes which use the fast Fourier transform (Engl. Fast Fourier Transformation, FFT) empathize with the functionality and accuracy of a measuring receiver. The main aim is to shorten long measurement times, as are necessary for measurements for electromagnetic compatibility . Measurements of this type are called time domain measurements or time domain methods in specialist circles. In Germany in particular, a lot of research has been carried out in recent years, and solutions have emerged, both implemented in commercial test receivers and from individual components (test receiver, digital oscilloscope, PC) for self-construction.
Terms
- Uniqueness
- An input signal must clearly match the scale or frequency display. In the other case one speaks of ambiguity.
- sensitivity
- The sensitivity of a receiver indicates how much stronger a useful signal (a radio broadcast or similar) has to be compared to the noise so that the reception of this useful signal is still possible. The input signal should still be easy to pick up despite the noise of the receiver stages and the external noise fed in via the antenna - regardless of the operating mode.
- fine tune
- Regardless of the type of broadcast (WFM - VHF radio, SSB, CW, etc.), there must be good coordination with the same selectivity.
- Compression, overload resistance
- Analogous to the sensitivity, it represents the upper limit of the dynamic range. It is indicated by the 1 dB compression point.
- Cross modulation, plugging effect
- If the information / modulation of a strong neighbor transmitter is taken over by the received transmitter, this is called cross modulation . In the case of keyed HF carriers, one speaks of the stuffing effect in the same case.
- selectivity
- The selectivity or selectivity refers to the ability of the receiver to filter out the desired one of a plurality of closely spaced in frequency transmitters.
- Image rejection
- The value of the image frequency suppression indicates how well image frequencies are attenuated, the value is in decibels , higher values are better.
- stability
- The useful signal should always be easy to receive, regardless of thermal and / or electrical influences.
- Overlay
- The addition of two vibrations is called superposition. The term is actually not correct for the heterodyne receiver, since a mixture (i.e. a multiplication) takes place here. What is meant, however, is the addition of the amount in decibels , which is equivalent to a multiplication.
Mathematical appendix
The formation of the two sidebands during mixing (ideal mixer; multiplier) can be explained mathematically as follows:
The input signal is
- ,
is the signal of the ideal tuning oscillator
- .
The output of the multiplier is thus
- .
Applying the addition theorems results in
- .
The part corresponds to
- the upper sideband ( )
and
- the lower sideband ( ).
Others
Block diagram of a conventional stereo -Empfängers ( receiver ) with VCO, PLL and microcomputer control:
View of the circuit board of a heterodyne receiver:
The FM tuner (1) contains the HF stages, the oscillator ( VCO ) and the mixer. The HF stages and the oscillator are tuned using capacitance diodes . The tuner has, among other things, an input for the tuning voltage and an output for the oscillator frequency (for PLL). The three 10.7 MHz ceramic filters for the IF can be seen under (2). The IF is fed to the IC (3), which contains the FM demodulator, among other things. The frequently used 7.1 MHz quartz in (4) is responsible for the reference frequency of the PLL. The PLL-IC (usually an LM 7000, LM 7001) is an SMD component on the back of the circuit board and cannot be seen.
An application of the heterodyne principle in the infrared was realized with the Infrared Spatial Interferometer , in which the captured radiation is mixed with that from infrared lasers and converted into HF.
In general, the heterodyne detection principle is used in optical arrangements, e.g. B. by modulating very narrow-band monochromatic laser radiation by acousto-optical modulators (= local oscillator) and thus generating light frequencies that are slightly shifted up and down, which can then be easily separated from the output frequency by interference filters or Fabry-Perot etalons .
literature
- Jens Heinich: A short chronicle of radio history. Hein, Dessau 2002. ISBN 3-936124-12-4 .
- Martin Gerhard Wegener: Modern radio reception technology. Franzis, Munich 1985, ISBN 3-7723-7911-7 .
- Ferdinand Jacobs: Course in radio technology. Franzis, Munich 1951, ISBN 3-7723-5362-2 (2 volumes).
- Philips GmbH (Ed.): Philips Lessons. Volume 1. Introduction and Basics. Philips specialist books. Hamburg 1987.
- Otto Limann, Horst Pelka: Radio technology without ballast. Introduction to the circuit technology of radio receivers. 16th edition. Franzis, Munich 1984, ISBN 3-7723-5266-9 .
- Dieter Nührmann: The large work book electronics. Franzis, Poing 2001. ISBN 3-7723-5575-7
- Heinrich Hübscher (ed.) U. a .: Electrical engineering. Specialized training in communication electronics. Volume 2. Radio, television, radio technology. Westermann, Braunschweig 1989, ISBN 3-14-221330-9 .
- W. Rohländer: The Superhete. In: radio amateur. Theuberger, Berlin 1977, ISSN 0016-2833 , p. 193.
- Christoph Rauscher: Basics of spectrum analysis. 3rd edition, Rohde & Schwarz, Munich 2007, ISBN 978-3-939837-00-8 .
- Ralf Rudersdorfer: radio receiver compendium. 1st edition, Elektor, Aachen 2010, ISBN 978-3-89576-224-6 .
Web links
- Radiomuseum.org: "Mixing and frequency conversion" (very detailed article about mixer stages in radio receivers)
- Jogis Roehrenbude: Overlay receiver for AM (very detailed explanation)
- Funkschau No. 49, 1932 (Superhet special issue): pp. 385–388 pp. 389–392 (pdf, digitized from www.radiomuseum.org)
- Edwin Armstrong: Pioneer of the Airwaves - Edwin Armstrong pioneered the airwaves (English)
- Lucien Levy - Brief biography of Lucien Lévy (French)
- Barlow Wadley - Everything about the Barlow Wadley
- Script on the subject of receiver technology
- Radar receiver
- Requirements for measuring receivers according to CISPR 16-1-1 (English) (PDF; 365 kB)
Individual evidence
- ↑ Article about Edwin Armstrong in the English language Wikipedia
- ^ Alan Douglas: Who Invented the Superheterodyne ?. , Original article : The Legacies of Edwin Howard Armstrong. In: Proceedings of the Radio Club of America No. 3, 1990, Vol. 64
- ↑ Patent US1342885 : Method of Receiving High Frequency Oscillations. Inventor: Edwin H. Armstrong.
- ↑ Patent FR493660 .
- ↑ Patent FR506297 .
- ↑ Radiola AR-812 (English) → Website no longer available → Archive (September 14, 2005 9:35 am) ( Memento from September 14, 2005 in the Internet Archive )
- ↑ Old alarm clocks radio interference in aircraft traffic (heise.de)