Notochord

The chorda dorsalis (" back string "; from the Latin chorda " string " and dorsum "back") or notochord , axis rod , rarely the original spine and often simply chorda , is the original inner axis skeleton of all chordates (chordata) and their eponymous feature . The notochord is a long, thin and flexible rod in the back area.
All chordates create a chorda dorsalis at least as embryos , which is sometimes still covered by a connective tissue chordal sheath. Among the Chordata includes three still living animal groups: the Lanzettfischchen (Leptocardia), the skull Animals (Craniata) and tunicates (tunicates). In the lancet fish and some adult cranial animals, the notochord runs through the entire length of the body between the neural tube and the intestinal canal . In the rest of today's cranial animals, it regresses completely or apart from small remnants, because it is replaced by the spine (and the intervertebral discs ). Tunicata, on the other hand, only develop a back string in the oar tail of the larva .
The notochord has both a skeletal and developmental function. In its skeletal function, it serves as a flexible inner skeleton that stretches and stabilizes the shape of the body, as well as a point of attachment for muscles that bend the body. The developmental function is essentially to initiate the formation of certain other tissues and organs during embryonic development , including the central nervous system .
term

In 1828, the German-Baltic naturalist Karl Ernst von Baer published his observations on the development of chicken embryos . He had noticed a structure in the embryos that ran lengthways through the body on the back of the intestinal canal during the formation of the neural ridges.
"Another part is formed with the back plates, which I call the back string (chorda dorsalis)."
The term notochord found its way into the emerging research field of developmental biology . During the next 20 years, several recent chordates were also anatomically examined that retained their chordae dorsales in adulthood. Finally, in 1847, the British paleontologist Richard Owen mentioned the back string for the first time in a fossil, the Plesiosaurus .
"Viewing the subvertebral wedge-bones in the wider relations, I subsequently described them as 'detached developments of bone in the lower part of the capsule of the notochord (chorda dorsalis, auct.)'."
"Looking at the subvertebral wedge-shaped bones in the larger context, I described them below as 'independent bony formations in the lower section of the sheath of the notochord (chorda dorsalis, auct.)'."
Richard Owen had not only opened the term for paleontology, he had replaced it. Instead notochord he preferred his own expression notochord . Over the next few decades, Owen's creation of words became widely accepted in the English-speaking world (and thus internationally). Today the use of chorda dorsalis is restricted to German-language publications.
The chorda dorsalis was used 27 years later by the German zoologist Ernst Haeckel to construct the group name "chordathiere". He thus provided the word basis for the term chordata (stringed back animals), which was coined in 1875 by the British zoologist William Sweetland Dallas for a translation project. However, the term is now often and incorrectly attributed to the British geneticist William Bateson .
construction
There are four tissue variants of the chorda dorsalis among today's chordates. The variant, which occurs in almost all skull animals, is considered to be original. As a result, the last common ancestor of all chordata had a chorda dorsalis, as they are still made up by skull animals today. The other three variants then represent simplifications or specializations that have evolved independently in the respective lines of development from the original state. In all four variants, the back string always appears as a dorsally located rod-shaped structure with a pointed tip at the front and back.
Lancet fish
In the lancetfish, the notochord extends from the tip of the head to the tip of the tail and consists of a rod of epithelial muscle cell plates. The specialized muscle tissue is initially created with epithelial cells , which then transform into muscle cells with striated muscle fibers from the myofilaments paramyosin and actin . These epithelial muscle cells then dissect further to form epithelial muscle cell plates. The flat epithelial muscle cell plates have round cross-sections and lie one behind the other like money rolls within the notochord. Few of the plates contain cell nuclei . The density of the muscle fibers can differ greatly between adjacent plates, with thirty to forty epithelial muscle cell plates taken together having the width of one of the trunk muscle strips ( myomers ) adjoining outside . The plates are separated from one another by narrow, gel-filled intercellular spaces . The force transfer from the epithelial muscle cell plates to the body of the lancet fish is carried out by hemidesmosomes that run between the plates and the innermost layer of the notch sheath immediately above them. When slackened, the back string has a round cross-section, while when it is tense it has a highly oval cross-section. The muscular work of the chorda dorsalis enables the lancet fish to swim meandering and to dig backwards into the loose seabed.
Two narrow strips of network-like branched Müller cells lie dorsally and ventrally on the chordal tissue . Small cell processes that are filled with cell plasma ( plasma processes ) grow out of the dorsal Müller cells and especially the epithelial muscle cell plates . At the left and right corners of the dorsal side of the notochord, they continue to grow together and form the left and right chordal squirrels . There they pass through small pores through the thin cord sheath and finally reach the neural tube. On the neural tube, the muscle cell processes and Müller cell processes form synaptic connections with the nerve cells. Each process of the plasma forms a single synapse. The synaptic interconnection of the chordal squirrel is somewhat similar to the muscle innervation of roundworms ( e.g. Ascaris ). Their muscle cells of the skin muscle tube , however, form much wider plasma processes ( muscle arms), which also branch out like tufts and then form several synapses with different nerve cells.
Tunicates
In tunicates , the notochord occurs only in the tail and consists of a tube of epithelial cells. The cell plasma of the epithelial cells is rich in glycogen and sometimes has yolk inclusions . The epithelial cells can appear flat to cube-shaped and they enclose a cavity that is filled with a glycoprotein-rich gelatinous mass.
The chorda dorsalis, which is limited to the oar tail, is present in most species only in the larvae, which are always free-swimming, and is used for locomotion. After the larva has settled on the seabed, the back string is completely broken down. The tailed tunicates ( Copelata ) keep their back strings even when grown up, because they remain free-swimming their entire life. Your chorda dorsalis, which is bent at a right angle, is used for locomotion and for fanning food components.
Skull animals
The notochord of the cranial animals extends from the middle of the head to the tip of the tail. It consists of chordoid tissue , its own form of supporting tissue . The chordoid tissue turns out to be a long series of large epithelial cells without intercellular substance, which are connected to one another by desmosomes and gap junctions . Each cell contains many intermediate filaments and each (mostly only) one large cell vacuole , bulging with a glycogen-rich liquid. The vacuolated cells appear vesicular, the cytoplasm and the flattened cell nuclei are pushed to the narrow edges.
The chordoid tissue is first created as a series of large, flat epithelial cells ( money roll stage ). Then the vacuoles fill and the internal cell pressure ( turgor ) increases immensely. Each of the turgescent cells expands and the notochord is stretched as a whole. The high hydrostatic pressure of the turgescent epithelial cells gives the back string its stiffness with a certain flexibility. It forms a hydrostatic, resilient axis skeleton. Many of today's cranial animals only have a back string as embryos, which is then extensively or entirely replaced by a spinal column.
- Recent Hohlstachler (Actinistia) : The chorda dorsalis of the only current genus of the coelacanth , Latimeria , extends from the middle of the head to the tip of the tail and remains in the adult animal. It consists of a tube of epithelial cells, the free space of which is filled with an oily liquid. With a diameter between 35 and 40 millimeters, Latimeria has the widest back string of all recent animals.
Adult notochord
In certain chordates, the notochord persists into adulthood, so it remains there. Such an adult notochord permanently takes on the mechanical function of the axial skeleton. It is the flexible support rod that maintains the length of the torso and provides the counterpart for the torso and tail muscles, which enables bending and snaking movements. When the longitudinal muscles of the trunk contract, the back string prevents the entire side of the body from contracting at the same time.
Chord sheath

Every adult notochord is enveloped by a notochord ( vagina notochordalis , perichordal sheath ). Exclusively embryonic back strings have no chordal sheaths or only thin rims. The chordal sheath is cell-free and consists of many strong collagen fibers . Overall, it has a tight material character and is not stretchable. Contrary to popular belief, the chordal sheath does not consist of two, but three layers.
- Membrana elastica externa : The outer and thin layer of the notch sheath. The membrana elastica externa consists of elastic fibers. It passes into the notochord fiber sheath.
- Chordal Sheath : The middle and main part of the chordal sheath. The notochord fiber sheath consists of tight and non-stretchable fibers. It is responsible for the fact that the chordal sheath appears as a whole as non-stretchable.
- Membrana elastica interna : The inner and very thin layer of the chordal sheath. The membrana elastica interna consists of a fine network of elastic fibers and directly surrounds the notochord.
Almost immediately after their formation, the chordal cells secrete an enveloping, collagenous layer. A little later, more collagen fibers are released. They push themselves between the first cover and the chordal cells. When fiber formation finally stops, the cord sheath has built up completely. The first collagenous sheath becomes the external elastic membrane, the fibers inserted afterwards form the notochord fiber sheath and the internal elastic membrane.
If an adult chorda dorsalis has skeletal elements made of cartilage or bone ( vertebral elements ) or if it is constricted by vertebral bodies , its notch sheath remains rather thin. The chordal sheath is involved in the formation of the vertebral bodies, in that cartilage or bone-forming cells penetrate into it. On the other hand, the chord sheath remains thin in lancet fish, although lancet fish have neither vertebral elements nor vertebral bodies. This is due to the short length of the chorda squirrels, which have to bridge the distance between the back string and the neural tube.
Embryonic notochord
In many of today's cranial animals, the notochord is only created in the embryo. It is a transitional organ that is later often completely replaced by a spine or narrowed to small areas between and in the vertebral bodies. With the formation of the vertebral bodies, the dorsal chord begins to regress. The embryonic notochord has an inductive function for embryogenesis . It triggers the development and differentiation of other tissues in its vicinity and is considered to be the most important inducer of the other embryonic body axis organs, which also include the somites as well as the neural ridges and the neural tube .
In the model organism clawed frog ( Xenopus laevis ), the origins of the embryonic chorda dorsalis can be traced back to the bladder germ ( blastula ). The bladder germ represents an embryonic stage that is passed through by lancetfish, tunicates and modern amphibians. In the other chordates, the bladder germ corresponds to a germinal disc ( blastodiscus ). The bubble germ already has an above and a below, its shape can be divided into an animal and a vegetative hemisphere. The cells of the vegetative hemisphere express the morphogens vg-1 (synonym bmp6 ) and nodal to produce the proteins called Vg-1-related protein and nodal . Both substances are released from the cells and drift to the central area of the bladder germ, the marginal zone . The proteins induce the expression of the morphogen t (brachyury) in the marginal zone cells for the synthesis of the protein Brachyury . The mesoderm ring of the bladder germ is created. The embryo then moves to the next phase of its development, gastrulation . The notochord will soon develop:
Gastrulation begins when the bladder sprouts indent at a point just below the marginal zone and turn inward in the shape of a crescent. The invagination is called the primordial mouth ( blastopore ), which corresponds to the primitive groove in umbilical animals . The Brachyury-expressing cells of the marginal zone are also captured by the invagination movement. They migrate over the upper edge of the primordial mouth into the interior of the germ.
The upper edge of the original mouth is also called the dorsal original mouth lip ( Spemann organizer ), which corresponds to the primitive knot ( Hensen's knot ) in umbilical animals . After bottle cells and the cells of the prechordal plate, the third group of cells migrate into the interior of the germ, which is called the second field of the Spemann organizer . In addition to the morphogen t (brachyury), they express the morphogen not , which belongs to the homeobox genes ( Hox genes). These cells connect with the primeval intestine ( archenteron ), a tubular structure that grows from the primordial mouth into the interior of the germ. For the time being, the cells remain in the middle of the upper outer surface of the primitive intestine. This median of t (brachyury) - and not -expressing cells in the roof of the primitive intestine is the prechordal mesoderm , the notochord . Left and right of the median are the two side strips of the primeval gut roof. Their cells, too, originally come from the marginal zone, but were there slightly to the side of the dorsal lip of the primordial mouth and now represent the presomitic mesoderm , the somites .
Shortly afterwards, the notochord tissue constricts again from the primitive intestine. It migrates upwards into the space between the primitive intestine and the embryonic outer wall, which here consists of animal ectoderm. The cells of the notochord grow into the rod-shaped notochord, which is initially hollow in mammalian embryos. In the specialist literature, only this very last phase of the process is often mentioned, as a result of which the notochord could appear as an endodermal structure made up of cells of the primitive intestine. The cells of the chorda dorsalis have already become mesoderm - more precisely, chordamesoderm - due to the expression of the morphogen t (brachyury) . At the same time as the notochord, the tissue of the pair of somites from the primitive intestine constricts ( enterocoelia ). It migrates laterally upwards into the space between the primitive intestine and the embryonic outer wall, slightly above the left and right to the chorda dorsalis. The cells of the somite systems initially form the paired and vesicular paraxial mesoderm .
The cells of the new notochord express several morphogens, including chrd and nog, to provide the proteins chordin and noggin . The proteins are released by the notochord cells and diffuse to the cells of the animal ectoderm that is in the immediate vicinity above the notochord. There they stop the inhibitory effect of a protein called BMP-4 . No longer inhibited, the ectoderm cells differentiate into nerve precursor cells ( neuroblasts ), from which the neural tube and neural crest are then formed. The notochord induces the onset of neurulation . At about the same time, the paired paraxial mesoderm breaks down lengthways into the somites , two rows of mesodermal vesicles. The disintegration begins behind the head system. The notochord is not involved in it, but it will largely determine the fate of the somites.
In zebrafish ( Danio rerio ) embryos , it was discovered that the cells of the notochord temporarily express four homeobox genes called hoxb1 , hoxb5 , hoxc6 and hoxc8 . However, their gene expressions do not happen simultaneously and not everywhere in the back string. Instead, they are read one after the other in terms of time and location. For example, the expression of the morphogen hoxb1 begins in the embryo ten and a half hours after fertilization. It shuts down sharply after the ninth pair of somites is formed and ends completely when the twelfth pair of somites is formed. The hoxb1 expression is locally restricted to the anterior section of the notochord . The three other homeobox genes are then also activated and deactivated one after the other, both temporally and spatially. The gene products of the Hox genes probably contribute to the fact that cells adjacent to the back string form different tissues in different parts of the body.
The cells of the notochord also express the morphogen shh , with which the protein Sonic hedgehog is synthesized. Sonic hedgehog is built into the dorsal side of the membranes of the chordal cells. As a result, the cells on the underside of the neural tube, which lie directly on the notochord, are touched by the Sonic hedgehog. In response to this signal, the cells that are touched thicken to form the base of the neural tube. The base plate cells in turn produce Sonic hedgehog. Some of it is placed inside the neural tube, with the concentration of Sonic hedgehog decreasing with distance to the base plate and disappearing entirely at the approximate center of the neural tube. Sonic hedgehog leads to different differentiations of the neuroblasts along its concentration gradient. In close proximity to the base plate, V3 interneurons arise , above them motor neurons and further to the middle of the neural tube, other interneuron types. The notochord induces the dorso-ventral division of the neural tube by means of the membrane-based Sonic hedgehog.
Sonic hedgehog, on the other hand, is given in a water-soluble form in the gap between the notochord and the somites. It reaches the inner lower parts of the somites, which are closest to the notochord. In response to this signal, the cells of those somite parts differentiate into sclerotome cells. They gradually detach from the residual somites and migrate towards the notochord. There they will soon wrap themselves around the back string and lace it into vertebral bodies (initially still cartilaginous). However, as the sclerotome cells begin to migrate, the notochord gives off further Sonic hedgehog. The substance is now involved in allowing some of the remaining somites to differentiate into muscle precursor cells ( myoblasts ). The cells of these myotomes in turn interact with sclerotome cells that have not yet migrated and convert them to syndetomes . Then the myoblasts emigrate and the dermatomes remain as the last remnants of somites . All parts of the somites will eventually migrate through the entire body and create tissue in many places: the sclerotome cells, the vertebral bodies, the myotome cells, the striated muscles, the syndetoma cells, tendon-like structures and the dermatome cells, the dermis . The notochord induces the disintegration of the somites as well as directly (sclerotome and myotome) and indirectly (syndetome and dermatome) the further differentiation of the thus cells by water-soluble Sonic hedgehog.
With the constriction by the vertebral bodies, the inductive effect of the back string ebbs.
evolution
The development of the chorda dorsalis is inextricably linked with the tribal history of the chordates. Their first traces are more than 520 million years old. Chordata are among the new mouth animals ( Deuterostomia ), which also include echinoderms ( Echinodermata ) and gill animals ( Hemichordata ). The original new mouth animals were mirror-symmetrical ( bilaterally symmetrical ) and had the tendency to form internal skeletons ( endoskeletons ). It is not clear whether they got stuck ( benthic-sessile way of life ) or crawled across the sea floor ( benthic-vagile way of life ) or whether they were free-swimming ( pelagic way of life ).
Hydroskeleton
At least the direct ancestors of the chordates preferred a vagile way of life. At first they looked very worm-like and probably had the same physique: Directly under the body surface was a skin muscle tube, which consisted of a layer of longitudinal muscles and a layer of circular muscles. The intestinal tube was located in the center of the inside of this muscle tube. The space between the muscle tube and the intestinal tube was taken up by the secondary body cavity , the coelom. The body cavity was filled with a liquid. The hydrostatic pressure of the body cavity fluid kept the skin muscle tube and intestines permanently at a distance and prevented internal injuries because the intestinal tube and skin muscle tube did not rub against each other. In addition, the body cavity fluid served as a deformable, but not compressible, hydroskeleton . The evolutionary benefit of the hydroskeleton lay in the lowering of the energy required for movement: the longitudinal muscles on one side of the skin muscle tube contract for a snaking-crawling locomotion. In animals without a hydroskeleton, the circular muscles contract at the same time so that the side of the body does not thicken like lumps and press on the internal organs. The use of the circular muscles increases the energy expenditure considerably. On the other hand, a hydroskeleton provides hydrostatic resistance to the contracting longitudinal muscles and the work of the circular muscles can be saved. The mobility of the worm-like body can, however, be increased if partition walls are drawn into the body cavity. The chambering of the hydroskeleton by such connective tissue septa improves control over the deformations that the worm-like body experiences through movements, so that crawling and digging movements are simplified. In addition, effective swimming movements were now possible. Evolutionarily the first pelagic way of life was further improved by the worm-like and chambered body being stretched and flattened laterally. This evolutionary stage may have been preserved in fossils of the genus Yunnanozoon (= Cathayamyrus , Haikouella , Zhongxiniscus ) from the Lower Cambrian .
Back string

The free-swimming, flattened worms then embarked on an evolutionary path that led to the chordata. Muscle ligaments were developed that ran parallel on both sides of the body from the back to the abdomen (segmental body muscles). These myomers enabled well-coordinated meandering swimming. However, their usefulness would be very limited if they were stretched over a wide hydroskeleton. That is why segmental body muscles needed a new and slim axial skeleton. The axial skeleton served as a starting point for the muscle ligaments and did not prevent them from working. It ensured meandering swimming and at the same time permanently maintained the streamlined body shape. However, it should not be too stiff to allow further movement: the notochord is the flexible endoskeleton that has evolved to adapt to the pelagic way of life. The evolutionary formation of the notochord was directly associated with a prolonged expression of the gene t (brachyury) in those mesodermal cells that were deposited as median strips on the upper outer surface of the urgut during early embryonic development.
With the back string, the hydroskeleton became superfluous and it was lost. The drive apparatus of the chordates was limited to the dorsal half of the body with myomers and chorda dorsalis - as a unique chorda-myomer apparatus of the chordates. The myomers were still separated by myosepta. These are thin layers of connective tissue that isolate myomers from one another. They made it more difficult to skip muscle excitement, thus improving muscle coordination and further refining mobility. Today's lancetfish ( Leptocardia ) represent a rather little modified form of such chordata . However, so far no fossils of ancestors of the small and completely hard-part-free living beings have been found.
The oldest fossilized chordates belonged to the group of the skullless Myllokunmingiida and came from Lower Cambrian and Middle Cambrian. Their genera are called Haikouichthys (= Myllokunmingia ) and Zhongjianichthys and Metaspriggina . The axial skeleton of the Myllokunmingiida consisted of the notochord. It is possible that they had collagen-free cartilage tissue , but this hardly contributed to the axial skeleton. The body structure continued to exist in a very similar way with the Pikaiidae , whose evolutionary line can be proven fossil in the Central Cambrian.
Tooth-like structures called conodonts have been found in the sub-Cambrian rock formations . In those layers they are assigned to the genus Protohertzina . Conodonts can then be found in many rocks. The last occurrences end with the Triassic . The bodies of the animals that carried the conodonts had no hard parts. The shape of the conodont animals ( Conodontophora ) is known only from extremely few prints, for example from the much later living genus Clydagnathus from the Lower Carboniferous . According to their fossils, the Conodontophora had elongated, lanceolate bodies with continuous back strings. In addition, a preform of the skull appeared. Because of the pre-shape of the skull, the front end of the notochord was shortened. It no longer began at the top of the body, but rather in the middle of the head, behind the eyes.
Back string regression
All the animals mentioned so far lived pelagic. In the Lower Cambrian, however, the tunicata, the tunicata, split off. The oldest genus of tunicates from that time is called Shankouclava . While Myllokunmingiida, Pikaiidae and Conodontophora realized the pelagic way of life using their back strings, tunicates took a different route. In its line of development, the notochord, which had only evolved a few million years ago, has already been broken down in two ways. On the one hand it was limited in time to free-swimming larval stages and on the other hand it was spatially limited to the oar tail. Much later, tunicates of the order Aplousobranchia could have become sexually mature before they became adults ( neoteny ). The tailed tunicates ( Copelata ) could have emerged from their offspring . The tiny animals remain in a kind of larval stage with their tail chorda bent at right angles for their short life.
Systematics of the chordata taking into account adult persistent chordae dorsales a | |||||||||||||||||||||||||||||||||||||||||||||||||||||||||
---|---|---|---|---|---|---|---|---|---|---|---|---|---|---|---|---|---|---|---|---|---|---|---|---|---|---|---|---|---|---|---|---|---|---|---|---|---|---|---|---|---|---|---|---|---|---|---|---|---|---|---|---|---|---|---|---|---|
|
|||||||||||||||||||||||||||||||||||||||||||||||||||||||||
|
|||||||||||||||||||||||||||||||||||||||||||||||||||||||||
Bold type : adult animals owned or have back strings instead of spinal cords . | |||||||||||||||||||||||||||||||||||||||||||||||||||||||||
a : The back strings of the animals mentioned are not completely regressed or replaced by spinal columns. So chordae dorsal occur not only in larvae or embryos instead outlast ( persist ) they (in adult adult ) forms. |
Vortex elements
Even during the Cambrian, the first skull animals ( Craniata ), the round mouths ( Cyclostomata ) , emerged from skullless preforms . Lampreys ( Petromyzontida ) and hagfish ( Myxinoidea ) belong to the group of round mouths . However, their soft, boneless bodies very rarely left fossils behind. That is why the oldest known lampreys come from the Upper Devonian ( Priscomyzon ) and Lower Carboniferous ( Hardistiella ).
The back string always persisted in the lampreys. Arched cartilage structures called neural arches ( arcus neural ) were attached dorsally . The neural arches were probably the first vertebral elements and early forms may have existed in the Haikouichthys . Vertebral elements were skeletal elements made of cartilage or (later also) made of bone and can flank the back string on all sides ( parachordal elements or perichordal elements ). Vortex elements cause an additional strengthening of the axial skeleton. When vertebral elements join the notochord , it is called camptospondyly . Although no vertebral elements have so far been discovered in fossil lampreys and other fossil jawless fish, this is very likely because they were made of cartilage and have not been preserved.
The oldest fossils of the hagfish come from the Upper Carboniferous ( Myxinikela , Myxineidus ). The chorda dorsalis of the hagfish has no vertebral elements, there is aspondyly . The vertebral elements regressed when the hagfish's unique mobility evolved because they can knot their bodies. Only hagfish among skull animals have an aspondyle back string.
skull

The evolution of the skull displaced the notochord from the head region. From then on, the back string in all cranial animals began only behind the pituitary gland ( pituitary gland ) at the base of the posterior part of the skull, the brain skull ( neurocranium ). Original cranial animals had an axial skeleton made up of an adult chorda dorsalis, which attached to the rear end of the skull and was framed by parachordal elements, of which the neural arches were the oldest in evolution. The situation can be traced on the one hand to recent lampreys and on the other hand to fossil, also jawless shell skins ( Ostracodermi ), which are known with Anatolepis from the Upper Cambrian onwards.
This part of the body did not change for the time being when the jaw mouths ( Gnathostomata ) evolved from the shell skins. Because of certain similarities in the structure of the olfactory organ, they probably emerged from a group of shell-skins called Galeaspida . The first occurred in gnathostomes Unterordovizium the armored fish on ( Placodermi ). However, their oldest fossil occupied genres come with Shimenolepis only from the Untersilur and Silurolepis from the Upper Silurian. Shortly before the Middle Ordovician, the real jaw- mouthed fish split off ( eugnathostomata ), because the first skin scales of such fish were discovered in the Middle-Ordovician rock layers.
A split of the real jaws from the tank fish is suggested by the fossils Qilinyu and Entelognathus , which had survived into the Upper Silurian and whose skulls contained mosaics of placoderma and eugnathostome bones. Two groups emerged from the basal real jaw mouths, the cartilaginous fish ( Chondrichthyes ) and the real bone animals ( Euteleostomi ). Their basic common ancestors still possessed a mosaic of features of both successor groups, as can be seen in the fossil fish Janusiscus , which occurred as far as the Lower Devonian. The cartilaginous fish divided into barbed sharks ( Acanthodii ) and Elasmobranchomorpha . With Sinacanthus and Kannathalepis , both groups can be traced back as fossils to the Lower Silurian . The Elasmobranchomorpha emerged from barbed sharks, which can be traced back to the skull, the teeth and the shoulder girdle of the mosaic shape Doliodus from the Lower Devonian . The real bones were also divided into two lines of evolution, into ray fins ( Actinopterygii ) and meat fins ( Sarcopterygii ). Early forms of the ray fins are documented with Andreolepis / Lophosteus and Meemannia from the Upper Silurian and Lower Devonian respectively. The first fossil early forms of the meat- fin fish named Guiyu , Psarolepis , Achoania and Sparalepis date from the same time horizon . The seven genera still combined features of both groups like a mosaic, but led either a little more in the direction of ray fins or in the direction of meat fins.
Spine
It had been about 70 million years since Anatolepis . Nevertheless, the kamptospondylar back strings persisted in all tankfish and spiny sharks as well as in all other cranial animals mentioned so far. Then, however, the notochord was interrupted four times independently of each other by vertebral bodies ( centra ) by growing around it and constricting it or replacing it completely with cartilaginous or bony spinal columns ( columnae vertebrales ). Vertebral bodies developed from parachordal elements. They were switched to the notochord at regular intervals. On the one hand, the spine caused a further strengthening and stiffening of the axial skeleton and, on the other hand, offered very firm muscle anchoring and strong muscle resistance.
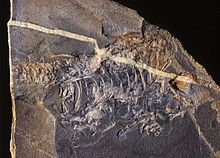
Vertebral bodies grew around and into the notochord. If there is a remnant of the back string in the center of the vertebral body, intravertebral chorda persistence is present. Likewise, some notochord could remain between the vertebral bodies in intervertebral chorda persistence . The intervertebral notochord remnants ensure the mobility of the spine. But this last function of the back string was finally given up in some lines of evolution. Then the vertebral joints evolved , with which the vertebral bodies are directly connected to one another in a movable manner. On the other hand, vertebral bodies could eventually grow together in order to evolve as much as possible. In both cases, the notochord was no longer needed at all and was completely involved:
- Neoselachii : A group of Elasmobranchomorpha from the ranks of the plate gills ( Elasmobranchii ) evolved to the new sharks ( Neoselachii ). They include all sharks and rays living today. Their fossil first representative came from the youngest Lower Devonian and is called Mcmurdodus . In the new sharks, the chorda dorsalis was replaced by a spinal column made of calcareous cartilage (calcareous cartilage). The chordal sheath became cartilaginous and overgrown with parachordal elements. Both the joint bodies and the neural arches were connected to one another by connective tissue . Such a spine would be very stiff if it did not remain flexible due to its cartilage and consist of up to 400 individual vertebrae. Chordadorsalis remnants remained inside the vertebral bodies, and there is intravertebral chorda persistence.
- Actinopterygii : From the original ray fins ( Actinopterygii ) those fishes developed in the lower Carboniferous from which all still living ray fins are descended. The raft fish ( Cladistia ) split off from this crown group of ray-finned fish , which have preserved many of the original anatomical features and whose first known fossil representatives are seen in a group called Scanilepiformes . In the raft fish it can be proven that the ray fins of the crown group developed a strong bony spine with intravertebral and intervertebral chorda resistance. Raft fish of the genus Serenoichthys from the Upper Cretaceous had spinal columns with about 30 vertebral bodies. Because of the higher tensile strength of bone tissue, the vertebral bodies of the ray fins are thinner than those of the cartilaginous fish. Often they are loosely connected with each other with connective tissue and real vertebral joints are missing. A little later, the spine of the cartilaginous organoids ( chondrostei ) was exchanged for a kamptospondyle chorda dorsalis, but overall the bony spine prevailed under radiation fins. It was passed on to the evolutionary line of the Neuflosser ( Neopterygii ), in which various genera are discussed as the first fossil representatives. Within the Neuflosser group of bone organoids ( Holostei ), the ossification of the spine progressed particularly far with intravertebral and intervertebral chorda persistence. In Lepisostes, from the group of the bonehike-like ( Lepisosteiformes ), real vertebral joints are finally formed and the vertebral bodies ossify completely - a unique finding among fish.
- Amniota : A branch of the quadruped terrestrial vertebrates ( Tetrapoda ) evolved into the umbilical animals ( Amniota ). Its first fossil-based representative from the Lower Carboniferous is called Casineria . Outside the water there was no buoyancy to support the carcasses. Instead, gravity weighed completely on the skeleton. This gave rise to the evolutionary pressure to evolve from the vertebral elements of their flesh-finned ancestors real, load-bearing spinal columns. A few broad vertebral bodies were built into the notochord and articulated to one another. Fibrous cartilaginous intervertebral discs grew in the crevices between the vertebral bodies and replaced the intervertebral notochord. In many umbilical animals living today, a remnant of the back string remains in the vertebral bodies. Only in older adult birds (Aves) and in mammals ( Mammalia ) is the intravertebral chorda persistence completely terminated.
- Lissamphibia : The modern amphibians ( Lissamphibia ) also belong to the land vertebrates and their axial skeleton was similarly adapted to carry the body weight on land. However, their spine developed independently of those of the umbilical animals. Cartilaginous intervertebral discs replace the notochord between the articulated vertebral bodies. In today's species there is often an intravertebral chorda persistence. Only among the real rigid-breasted frogs ( Firmisternia ), to which for example the real frogs ( Ranidae ) belong, the chordal tissue in the vertebral bodies is completely replaced by ossification ( holochordal ossification ). Modern amphibians appear fossil for the first time with Gerobatrachus in the lower Perm , their line of development should therefore have appeared a little earlier in the Upper Carboniferous. Within today's lissamphibiae, the embryonic or larval formation of the spine varies over time. On the one hand vertebral bodies at crawl amphibians (are Gymnophiona ) and urodeles ( caudata ) formed relatively early. On the other hand, the formation of the few vertebral bodies of frogs ( anura ) is delayed. That is why frogs retain a continuous notochord for longer during their individual development. They resemble the original and extinct group of amphibians, which are grouped together as labyrinth teeth ( Labyrinthodontia ). A continuous notochord survived into adulthood under certain labyrinthine teeth. Creeping amphibians and tail amphibians, on the other hand, tend to do the same thing as the pod vertebrae ( Lepospondyli ), which, independently of them, had also developed elongated and very slim bodies in the lower carbon.
The four independent evolution of the spine always proceeded from the same initial structures that were already present in the last common ancestor of all cranial animals: The notochord and the vertebral elements are homologous features among all cranial animals . The spine, however, evolved convergently four times from these same homologous initial features. As a result, the spine is not a homology, but a homoiology . Spinal columns brought the jaw mouths so great evolutionary advantages that most with aspondyler or kamptospondyler's notochord are now extinct. The surviving exceptions are sea cats ( Holostei ), cartilage organoids (Chondrostei), hollow spines ( Actinistia ) and lung fish ( Dipnoi ), each with a few recent species.
Chordoid structures

Organs and parts of organs that seem more or less similar to the chorda dorsalis can be called chordoid structures . However, the structures are neither evolutionary preforms ( oriments ) nor remnants ( rudiments ) of the back string. They are independent developments ( homoplasias ).
- Axochord : Annelidae and the other animals from the group of Lophotrochozoa form an axochord. It is a pair of strips of mesodermal muscle cells that lie between the digestive tract and the abdominal marrow . The axochord cells surround each other with a common shell made of tight collagenous connective tissue. Paired muscle groups that pull through the body on the stomach side attach to it. An axochord has also been discovered in arrow worms ( Chaetognatha ). Thus, this pair of abdominal muscle strips could be a structure that the last common ancestor of all present-day primeval mouth animals ( protostomia ) had around 542 million years ago.
- Buccal cirrus of the lancet fish (Leptocardia): The mouth opening of the lancet fish is surrounded by cirrus ("lip tentacle", "buccal cirrus", "mouth cirrus"). Each cirrus is traversed by a thin rod of filament-rich cells, lined up one behind the other, laterally flattened. As a result, each rod has a structure that closely resembles a tiny notochord.
- Chorda intestinalis : The chorda intestinalis occurs in flatworms ( platelets ) of the genus Nematoplana . It is located in the roof of the intestine at the front end of the body. The chorda intestinalis stiffens the body shape of the flatworm with turgescent cells.
- Hypochorda : The hypochorda ( subnotochordal rod ) is a unicellular flat strip that is created in non-amniotic cranial animals during the embryonic phase. The hypochord only separates from the roof of the primitive intestine after the mesodermal chordal and somite structures have already completely constricted. Therefore, the hypochord is endodermal tissue. The hypochord is drawn in between the roof of the primitive intestine and the chorda dorsalis. Their constriction takes place with the help of a chemical signal that emanates from the immediately adjacent notochord. The hypochord itself releases a protein from the VEGF group , which induces the formation of the dorsal aorta .
- Nucleus pulposus : In mammals, the central nucleus (forms the nucleus pulposus ) together with the annular fibrocartilaginous fibrous ring ( annulus fibrosus s) of the intervertebral disc . However, the core consists neither of cartilage nor of chordamesoderm, but of a viscous amorphous jelly. It contains highly water-absorbing glycosaminoglycans and collagen fibers. The jelly represents a kind of water cushion, the hydrostatic pressure of which spans the fiber ring. The core is not a remnant of the back string, but a replacing new formation. The substances of the nucleus pulposus are provided by the chordal cells. When an intervertebral disc is created in the embryo, the notochord-dorsal segment enclosed in it releases glycosaminoglycans and collagen fibers into the environment. The substances released expand the space to the dimensions of the nucleus pulposus. The enclosed chorda dorsalis then first disintegrates into compact chordal segments . They are further broken down into a network-like frayed residue called the chordareticulum . The chordareticulum consists of a few and non-turgescent epithelial cells of the chordal mesoderm. Over time, the epithelial cells become less and less, in humans the last disappear after the third year of life. During adolescence, individual chondrocytes and rounded cells that resemble fibroblasts exist in the nucleus of the gel . In adults, the interior of the gelatinous nucleus becomes largely cell-free. In addition, the proportion of glycosaminoglycans gradually decrease and the amount of collagen fibers increases. As a result, the core loses water and expansion. The gap left by its shrinking edges is filled by the fibrous cartilage of the fibrous ring that grows afterwards. In rare cases, small remnants of the notochord may remain at the anterior or posterior end of the spine. The remains can grow into lumps called chordomas .
- Stomochord : The stomochord is a stiff and elongated pouch of the digestive tract ( intestinal diverticulum ), which starts from the roof of the mouth in gill lobsters ( hemichordata ) and extends forward into the head lobe ( prosoma ). The stomochord stabilizes the transition from the head flap to the collar ( mesosoma ). The stomochord does not have the bladder-like or sac-like shape of a simple intestinal diverticulum, but can be divided into several sections that differ from one another in width and shape. The first flattened and bottle-like section rises from the roof of the mouth. Behind its bottle neck-shaped end is a middle section with pocket-like bulges. The middle section merges into a thin tube, which finally tapers to a rod-shaped extension. Only the process bears a certain resemblance to the notochord.
literature
- Thomas Stach: Chordate phylogeny and evolution: a not so simple three-taxon problem . In: Journal of Zoology . Volume 276, 2008, doi : 10.1111 / j.1469-7998.2008.00497.x , pp. 117-141.
- Dietrich Starck: Comparative Anatomy of the Vertebrates Volume 2 . Springer-Verlag, Berlin / Heidelberg 1979, ISBN 3-540-09156-4 , pp. 35-66.
- Derek L. Stemple: Structure and function of the notochord: an essential organ for chordate development . In: Development . Volume 132, 2005, doi : 10.1242 / dev.01812 , pp. 2503-2512.
Individual evidence
- ↑ a b c d Dietrich Starck: Comparative Anatomy of the Vertebrates Volume 2 . Springer-Verlag, Berlin / Heidelberg 1979, ISBN 3-540-09156-4 , p. 35.
- ↑ a b c d e f g h i j k l m Martin S. Fischer: musculoskeletal system . In: Wilfried Westheide, Gunde Rieger (Ed.): Special Zoology Part 2: Vertebrate or cranial animals . Springer-Verlag, Berlin / Heidelberg 2015, ISBN 978-3-642-55435-3 , p. 49.
- ^ Alfred Goldschmid: Deuterostomia . In: Wilfried Westheide, Gunde Rieger (Ed.): Special Zoology Part 1: Protozoa and Invertebrates . Springer-Verlag, Berlin / Heidelberg 2013, ISBN 978-3-642-34695-8 , pp. 778, 786.
- ↑ Volker Storch, Ulrich Welsch: Kükenthal zoological internship . Springer-Verlag, Berlin / Heidelberg 2014, ISBN 978-3-642-41936-2 , pp. 307, 309, 317, 515.
- ↑ a b c d Dietrich Starck: Comparative Anatomy of the Vertebrates Volume 2 . Springer-Verlag, Berlin / Heidelberg 1979, ISBN 3-540-09156-4 , p. 38.
- ↑ Karl Ernst von Baer: About the history of the development of animals • First part . Verlag Gebrüder Bornträger, Königsberg 1828, p. 15.
- ↑ Johannes Müller: Comparative anatomy of the myxinoids . Physical-mathematical treatises, Berlin 1834, pp. 138-139.
- ↑ Hermann Stannis: Vertebrates . Verlag Veit & Compagnie, Berlin 1846, p. 4.
- ^ Richard Owen: Description of the atlas, axis and subvertebral wedge bones in the Plesiosaurus, with remarks on the homologies of those bones . In: Annals and Magazine of Natural History . Volume 20, edition 133, 1847, p. 16 ( digitized version ).
- ↑ See, for example, Neil A. Campbell, Jane B. Reece, Lisa A. Urry, Michael L. Cain, Steven A. Wasserman, Peter V. Minorsky, Robert B. Jackson: Biology . Pearson Education, Harlow 2015, ISBN 978-1-292-00865-3 , p. 1090, with Neil A. Campbell, Jane B. Reece, Lisa A. Urry, Michael L. Cain, Steven A. Wasserman, Peter V. Minorsky, Robert B. Jackson: Biology . Pearson Germany, Hallbergmoos 2016, ISBN 978-3-86894-259-0 , p. 1379.
- ↑ Ernst Haeckel: Anthropogeny or human development history . Engelmann Verlag, Leipzig 1874, p. 398.
- ^ Hermann Fol: On the primary Origin of the Sexual Products . In: Annals and Magazine of Natural History . Volume 16, 1875, pp. 157, 161.
- ^ Claus Nielsen: The authorship of higher chordate taxa . In: Zoologica Scripta . Volume 41, 2012, doi : 10.1111 / j.1463-6409.2012.00536.x , p. 435.
- ^ A b Volker Storch, Ulrich Welsch: Kükenthal zoological internship . Springer-Verlag, Berlin / Heidelberg 2014, ISBN 978-3-642-41936-2 , p. 317.
- ↑ a b Dietrich Starck: Comparative Anatomy of the Vertebrates Volume 2 . Springer-Verlag, Berlin / Heidelberg 1979, ISBN 3-540-09156-4 , pp. 42-43.
- ^ Alfred Goldschmid: Deuterostomia . In: Wilfried Westheide, Gunde Rieger (Ed.): Special Zoology Part 1: Protozoa and Invertebrates . Springer-Verlag, Berlin / Heidelberg 2013, ISBN 978-3-642-34695-8 , pp. 778, 796.
- ^ Alfred Goldschmid: Deuterostomia . In: Wilfried Westheide, Gunde Rieger (Ed.): Special Zoology Part 1: Protozoa and Invertebrates . Springer-Verlag, Berlin / Heidelberg 2013, ISBN 978-3-642-34695-8 , p. 796.
- ↑ a b c d e Dietrich Starck: Comparative Anatomy of the Vertebrates Volume 2 . Springer-Verlag, Berlin / Heidelberg 1979, ISBN 3-540-09156-4 , p. 40.
- ^ Alfred Goldschmid: Deuterostomia . In: Wilfried Westheide, Gunde Rieger (Ed.): Special Zoology Part 1: Protozoa and Invertebrates . Springer-Verlag, Berlin / Heidelberg 2013, ISBN 978-3-642-34695-8 , p. 799.
- ^ A b Alfred Goldschmid: Deuterostomia . In: Wilfried Westheide, Gunde Rieger (Ed.): Special Zoology Part 1: Protozoa and Invertebrates . Springer-Verlag, Berlin / Heidelberg 2013, ISBN 978-3-642-34695-8 , pp. 796–797.
- ^ Sievert Lorenzen, Andreas Schmidt-Rhaesa: Cycloneuralia . In: Wilfried Westheide, Gunde Rieger (Ed.): Special Zoology Part 1: Protozoa and Invertebrates . Springer-Verlag, Berlin / Heidelberg 2013, ISBN 978-3-642-34695-8 , pp. 428-429.
- ^ Armin Kyrieleis: Chorda dorsalis . In: Rolf Sauermost (Red.): Lexicon of Biology, Third Volume, Leaf to Cistus Oil . Spectrum Academic Publishing House. Heidelberg 2000, ISBN 3-8274-0328-6 , p. 432.
- ↑ a b c d Volker Storch, Ulrich Welsch: Kükenthal zoological internship . Springer-Verlag, Berlin / Heidelberg 2014, ISBN 978-3-642-41936-2 , p. 309.
- ^ Alfred Goldschmid: Deuterostomia . In: Wilfried Westheide, Gunde Rieger (Ed.): Special Zoology Part 1: Protozoa and Invertebrates . Springer-Verlag, Berlin / Heidelberg 2013, ISBN 978-3-642-34695-8 , pp. 786, 795.
- ↑ a b c d e f Dietrich Starck: Comparative Anatomy of the Vertebrates Volume 2 . Springer-Verlag, Berlin / Heidelberg 1979, ISBN 3-540-09156-4 , p. 39.
- ↑ a b c d e f Alfred Goldschmid: Deuterostomia . In: Wilfried Westheide, Gunde Rieger (Ed.): Special Zoology Part 1: Protozoa and Invertebrates . Springer-Verlag, Berlin / Heidelberg 2013, ISBN 978-3-642-34695-8 , p. 778.
- ↑ Wolfgang Kummer, Ulrich Welsch: musculoskeletal system . In: Ulrich Welsch, Thomas Deller, Wolfgang Kummer: Textbook Histology . Urban & Fischer Verlag, Munich 2014, ISBN 978-3-437-44433-3 , p. 308.
- ↑ Volker Storch, Ulrich Welsch: Kükenthal zoological internship . Springer-Verlag, Berlin / Heidelberg 2014, ISBN 978-3-642-41936-2 , p. 337.
- ↑ a b c d Milton Hildebrand, George E. Goslow: Comparative and functional anatomy of the vertebrates . Springer-Verlag, Berlin / Heidelberg 2004, ISBN 3-540-00757-1 , p. 166.
- ^ A b Volker Storch, Ulrich Welsch: Kükenthal zoological internship . Springer-Verlag, Berlin / Heidelberg 2014, ISBN 978-3-642-41936-2 , p. 306.
- ↑ a b Dietrich Starck: Comparative Anatomy of the Vertebrates Volume 2 . Springer-Verlag, Berlin / Heidelberg 1979, ISBN 3-540-09156-4 , p. 37.
- ↑ Linda K. Gont, Abraham Fainsod, Sung-Hyun Kim, Eddy M. De Robertis: Overexpression of the Homeobox Gene Xnot-2 Leads to Notochord Formation in Xenopus . In: Developmental Biology . Volume 174, 1996, doi : 10.1006 / dbio.1996.0061 , p. 176.
- ↑ Victoria E. Prince, Alivia L. Price, Robert K. Ho: Hox gene expression reveals regionalization along the anteroposterior axis of the zebrafish notochord . In: Development Genes and Evolution . Volume 208, 1998, doi : 10.1007 / s004270050210 , p. 520.
- ↑ Stefan Stein, Michael Kessel: A homeobox gene involved in node, notochord and neural plate formation of chick embryos . In: Mechanisms of Development . Volume 49, 1995, doi : 10.1016 / 0925-4773 (94) 00300-C , pp. 38, 41.
- ↑ a b c d Lothar fights, Rolf Kittel, Johannes Klapperstück: Guide to the anatomy of vertebrates . Gustav Fischer Verlag, Jena / Stuttgart / New York 1993, ISBN 3-334-60454-3 , p. 45.
- ↑ a b c Arno Hermann Müller: Textbook of Palaeozoology Volume III Vertebrates Part 1 Fish - Amphibians . Gustav Fischer Verlag, Jena 1985, ISBN 3-334-00222-5 , p. 18.
- ↑ Anonymous: Chord sheath . In: Rolf Sauermost (Red.): Lexicon of Biology, Third Volume, Leaf to Cistus Oil . Spectrum Academic Publishing House. Heidelberg 2000, ISBN 3-8274-0328-6 , p. 433.
- ↑ Werner A. Müller, Monika Hassel: Developmental and reproductive biology . Springer-Verlag, Berlin / Heidelberg 2012, ISBN 978-3-642-28382-6 , pp. 136, 179.
- ↑ Werner A. Müller, Monika Hassel: Developmental and reproductive biology . Springer-Verlag, Berlin / Heidelberg 2012, ISBN 978-3-642-28382-6 , pp. 116–117, 124–125.
- ↑ Werner A. Müller, Monika Hassel: Developmental and reproductive biology . Springer-Verlag, Berlin / Heidelberg 2012, ISBN 978-3-642-28382-6 , pp. 134-135, 150, 156-157.
- ↑ Werner A. Müller, Monika Hassel: Developmental and reproductive biology . Springer-Verlag, Berlin / Heidelberg 2012, ISBN 978-3-642-28382-6 , p. 129.
- ↑ Werner A. Müller, Monika Hassel: Developmental and reproductive biology . Springer-Verlag, Berlin / Heidelberg 2012, ISBN 978-3-642-28382-6 , pp. 124–125.
- ↑ Werner A. Müller, Monika Hassel: Developmental and reproductive biology . Springer-Verlag, Berlin / Heidelberg 2012, ISBN 978-3-642-28382-6 , pp. 124, 129.
- ↑ a b Werner A. Müller, Monika Hassel: Developmental Biology and Reproductive Biology . Springer-Verlag, Berlin / Heidelberg 2012, ISBN 978-3-642-28382-6 , pp. 116-117.
- ↑ Werner A. Müller, Monika Hassel: Developmental and reproductive biology . Springer-Verlag, Berlin / Heidelberg 2012, ISBN 978-3-642-28382-6 , pp. 139, 150.
- ↑ Werner A. Müller, Monika Hassel: Developmental and reproductive biology . Springer-Verlag, Berlin / Heidelberg 2012, ISBN 978-3-642-28382-6 , p. 123.
- ↑ Werner A. Müller, Monika Hassel: Developmental and reproductive biology . Springer-Verlag, Berlin / Heidelberg 2012, ISBN 978-3-642-28382-6 , p. 289.
- ↑ a b Werner A. Müller, Monika Hassel: Developmental Biology and Reproductive Biology . Springer-Verlag, Berlin / Heidelberg 2012, ISBN 978-3-642-28382-6 , p. 160.
- ^ Scott F. Gilbert: Developmental Biology . Sinauer Associates, Sunderland 2003, ISBN 0-87893-258-5 , p. 310.
- ↑ Werner A. Müller, Monika Hassel: Developmental and reproductive biology . Springer-Verlag, Berlin / Heidelberg 2012, ISBN 978-3-642-28382-6 , p. 124.
- ^ William S. Talbot, Bill Trevarrow, Marnie E. Halpern, Anna E. Melby, Gist Farr, John H. Postlethwait, Trevor Jowett, Charles B. Kimmel, David Kimelman: A homeobox gene essential for zebrafisch notochord development . In: Nature . Volume 378, 1995, doi : 10.1038 / 378150a0 , pp. 150, 156.
- ↑ Werner A. Müller, Monika Hassel: Developmental and reproductive biology . Springer-Verlag, Berlin / Heidelberg 2012, ISBN 978-3-642-28382-6 , p. 255.
- ↑ Werner A. Müller, Monika Hassel: Developmental and reproductive biology . Springer-Verlag, Berlin / Heidelberg 2012, ISBN 978-3-642-28382-6 , pp. 116-117, 119.
- ↑ Werner A. Müller, Monika Hassel: Developmental and reproductive biology . Springer-Verlag, Berlin / Heidelberg 2012, ISBN 978-3-642-28382-6 , p. 117.
- ↑ Hanaa Ben Abdelkhalek, Anja Beckers, Karin Schuster-Gossler, Maria N. Pavlova, Hannelore Burkhardt, Heiko Lickert, Janet Rossant, Richard Reinhardt, Leonard C. Schalkwyk, Ines Müller, Bernhard G. Herrmann, Marcelo Ceolin, Rolando Rivera-Pomar , Achim Gossler: The mouse homeobox gene Not is required for caudal notochord development and affected by the truncate mutation . In: Genes & Development . Volume 18, 2004, doi : 10.1101 / gad.303504 , p. 1733.
- ↑ Martin S. Fischer: Musculoskeletal system . In: Wilfried Westheide, Gunde Rieger (Ed.): Special Zoology Part 2: Vertebrate or cranial animals . Springer-Verlag, Berlin / Heidelberg 2015, ISBN 978-3-642-55435-3 , p. 50.
- ↑ a b c Dietrich Starck: Comparative Anatomy of the Vertebrates Volume 2 . Springer-Verlag, Berlin / Heidelberg 1979, ISBN 3-540-09156-4 , p. 36.
- ^ Rüdiger Wehner, Walter Gehring: Zoologie . Georg Thieme Verlag, Stuttgart / New York 2013, ISBN 978-3-13-367425-6 , p. 177.
- ↑ Werner A. Müller, Monika Hassel: Developmental and reproductive biology . Springer-Verlag, Berlin / Heidelberg 2012, ISBN 978-3-642-28382-6 , pp. 124, 184.
- ↑ Werner A. Müller, Monika Hassel: Developmental and reproductive biology . Springer-Verlag, Berlin / Heidelberg 2012, ISBN 978-3-642-28382-6 , p. 23.
- ↑ Werner A. Müller, Monika Hassel: Developmental and reproductive biology . Springer-Verlag, Berlin / Heidelberg 2012, ISBN 978-3-642-28382-6 , pp. 288-289.
- ↑ Werner A. Müller, Monika Hassel: Developmental and reproductive biology . Springer-Verlag, Berlin / Heidelberg 2012, ISBN 978-3-642-28382-6 , pp. 129, 131-132, 149.
- ↑ Werner A. Müller, Monika Hassel: Developmental and reproductive biology . Springer-Verlag, Berlin / Heidelberg 2012, ISBN 978-3-642-28382-6 , p. 190.
- ↑ Werner A. Müller, Monika Hassel: Developmental and reproductive biology . Springer-Verlag, Berlin / Heidelberg 2012, ISBN 978-3-642-28382-6 , pp. 117–118, 288–289.
- ↑ Victoria E. Prince, Alivia L. Price, Robert K. Ho: Hox gene expression reveals regionalization along the anteroposterior axis of the zebrafish notochord . In: Development Genes and Evolution . Volume 208, 1998, doi : 10.1007 / s004270050210 , pp. 518-519, 521.
- ↑ Victoria E. Prince, Alivia L. Price, Robert K. Ho: Hox gene expression reveals regionalization along the anteroposterior axis of the zebrafish notochord . In: Development Genes and Evolution . Volume 208, 1998, doi : 10.1007 / s004270050210 , p. 522.
- ↑ Monica R. Rohrschneider, Gina E. Elsen, Victoria E. Prince: Zebrafish Hoxb1a regulates multiple downstream genes including prickle1b . In: Developmental Biology . Volume 309, 2007, doi : 10.1016 / j.ydbio.2007.06.012 , p. 358.
- ↑ Werner A. Müller, Monika Hassel: Developmental and reproductive biology . Springer-Verlag, Berlin / Heidelberg 2012, ISBN 978-3-642-28382-6 , p. 260.
- ↑ James D. Watson, Tania A. Baker, Stephen P. Bell, Alexander Gann, Michael Levine, Richard Losick: Watson Molecular Biology . Pearson Studium, Munich 2011, ISBN 978-3-86894-029-9 , p. 735.
- ↑ Werner A. Müller, Monika Hassel: Developmental and reproductive biology . Springer-Verlag, Berlin / Heidelberg 2012, ISBN 978-3-642-28382-6 , pp. 184, 260, 290.
- ↑ Werner A. Müller, Monika Hassel: Developmental and reproductive biology . Springer-Verlag, Berlin / Heidelberg 2012, ISBN 978-3-642-28382-6 , pp. 118, 187, 260.
- ↑ Chris S. Blagden, Peter D. Currie, Philip W. Ingham, Simon M. Hughes: Notochord induction of zebrafish slow muscle mediated by Sonic hedgehog . In: Genes & Development . Volume 11, 1997, doi : 10.1101 / gad.11.17.2163 , p. 2163.
- ^ Ava E. Brent, Ronen Schweitzer: A Thusic Compartment of Tendon Progenitors . In: Cell . Volume 113, 2003, doi : 10.1016 / S0092-8674 (03) 00268-X , p. 240.
- ^ Ava E. Brent, Ronen Schweitzer: A Thusic Compartment of Tendon Progenitors . In: Cell . Volume 113, 2003, doi : 10.1016 / S0092-8674 (03) 00268-X , p. 235.
- ↑ Werner A. Müller, Monika Hassel: Developmental and reproductive biology . Springer-Verlag, Berlin / Heidelberg 2012, ISBN 978-3-642-28382-6 , p. 395.
- ↑ Werner A. Müller, Monika Hassel: Developmental and reproductive biology . Springer-Verlag, Berlin / Heidelberg 2012, ISBN 978-3-642-28382-6 , pp. 118, 121.
- ↑ Werner A. Müller, Monika Hassel: Developmental and reproductive biology . Springer-Verlag, Berlin / Heidelberg 2012, ISBN 978-3-642-28382-6 , pp. 184, 260.
- ↑ Shou Wang, Tomasz Furmanek, Harald Kryvi, Christel Krossøy, Geir K Totland, Sindre Grotmol, Anna Wargelius: Transcriptome sequencing of Atlantic salmon (Salmo salar L.) notochord prior to development of the vertebrae provides clues to regulation of positional fate, chordoblast lineage and mineralization . In: BMC Genomics . Volume 15, 2014, Article 141, doi : 10.1186 / 1471-2164-15-141 , p. 2.
- ↑ Reinhard Rieger, Gerhard Haszprunar, Bert Hobmayer: Bilateria . In: Wilfried Westheide, Gunde Rieger (Ed.): Special Zoology Part 1: Protozoa and Invertebrates . Springer-Verlag, Berlin / Heidelberg 2013, ISBN 978-3-642-34695-8 , pp. 165, 179.
- ^ Alfred Goldschmid: Deuterostomia . In: Wilfried Westheide, Gunde Rieger (ed.): Special Zoology Part 1: Protozoa and invertebrates . Springer-Verlag, Berlin / Heidelberg 2013, ISBN 978-3-642-34695-8 , p. 715.
- ^ A b Alfred Goldschmid: Deuterostomia . In: Wilfried Westheide, Gunde Rieger (Ed.): Special Zoology Part 1: Protozoa and Invertebrates . Springer-Verlag, Berlin / Heidelberg 2013, ISBN 978-3-642-34695-8 , p. 717.
- ↑ Noriyuki Satoh, Daniel Rokhsar and Teruaki Nishikawa: Chordate evolution and the three-phylum system . In: Proceedings of the Royal Society B , Volume 281, doi : 10.1098 / rspb.2014.1729 , p. 4.
- ↑ Jun-Yuan Chen: Early crest animals and the insight they provide into the evolutionary origin of craniates. In: Genesis . No. 46, 2008, doi : 10.1002 / dvg.20445 , p. 625.
- ↑ Pei-Yun Cong, Xian-Guang Hou, Richard J. Aldridge, Mark A. Purnell, Yi-Zhen Li: New data on the palaeobiology of the enigmatic yunnanozoans from the Chengjiang Biota, Lower Cambrian, China . In: Palaeontology . No. 58, 2014, doi : 10.1111 / pala.12117 , pp. 65–66, 68.
- ↑ Jun-Yuan Chen: Early crest animals and the insight they provide into the evolutionary origin of craniates. In: Genesis . No. 46, 2008, doi : 10.1002 / dvg.20445 , pp. 625, 627.
- ↑ Pei-Yun Cong, Xian-Guang Hou, Richard J. Aldridge, Mark A. Purnell, Yi-Zhen Li: New data on the palaeobiology of the enigmatic yunnanozoans from the Chengjiang Biota, Lower Cambrian, China . In: Palaeontology . No. 58, 2014, doi : 10.1111 / pala.12117 , p. 67.
- ↑ Pei-Yun Cong, Xian-Guang Hou, Richard J. Aldridge, Mark A. Purnell, Yi-Zhen Li: New data on the palaeobiology of the enigmatic yunnanozoans from the Chengjiang Biota, Lower Cambrian, China . In: Palaeontology . No. 58, 2014, doi : 10.1111 / pala.12117 , p. 46.
- ↑ Jun-Yuan Chen: Early crest animals and the insight they provide into the evolutionary origin of craniates. In: Genesis . No. 46, 2008, doi : 10.1002 / dvg.20445 , pp. 623-624.
- ↑ Degan Shu: A paleontological perspective of vertebrate origin . In: Chinese Science Bulletin . No. 48, 2003, doi : 10.1007 / BF03187041 , p. 733.
- ↑ Michael J. Benton: Paleontology of the vertebrates . Publishing house Dr. Friedrich Pfeil, Munich 2007, ISBN 978-3-89937-072-0 , pp. 21-22.
- ^ Robert L. Caroll: Paleontology and evolution of the vertebrates. Georg Thieme Verlag, Stuttgart / New York 1993, ISBN 3-13-774401-6 , p. 19.
- ↑ Nori Satoh, Kuni Tagawa, Hiroki Takahashic: How was the notochord born? . In: Evolution & Development . No. 14, 2012, doi : 10.1111 / j.1525-142X.2011.00522.x , pp. 63, 69.
- ↑ Dietrich Starck: Comparative Anatomy of the Vertebrates Volume 2 . Springer-Verlag, Berlin / Heidelberg 1979, ISBN 3-540-09156-4 , p. 44.
- ↑ Jun-Yuan Chen: Early crest animals and the insight they provide into the evolutionary origin of craniates . In: Genesis . No. 46, 2008, doi : 10.1002 / dvg.20445 , pp. 625, 627-628.
- ↑ Degan Shu: A paleontological perspective of vertebrate origin . In: Chinese Science Bulletin . No. 48, 2003, doi : 10.1007 / BF03187041 , p. 725.
- ↑ Hou Xian-gang, Richard J. Aldridge, David J. Siveter, Derek J. Siveter, and Feng Xiang-hong: New evidence on the anatomy and phylogeny of the earliest vertebrates . I. Proceedings of the Royal Society B: Biological Sciences . No. 269, 2002, doi : 10.1098 / rspb.2002.2104 , p. 1865.
- ↑ Degan Shu: A paleontological perspective of vertebrate origin . In: Chinese Science Bulletin . No. 48, 2003, doi : 10.1007 / BF03187041 , p. 727.
- ^ Simon Conway Morris, Jean-Bernard Caron: A Primitive Fish from the Cambrian of North America . In: Nature . No. 512, 2014, doi : 10.1038 / nature13414 , p. 419.
- ↑ Michael J. Benton: Paleontology of the vertebrates . Publishing house Dr. Friedrich Pfeil, Munich 2007, ISBN 978-3-89937-072-0 , p. 55.
- ↑ Michael J. Benton: Paleontology of the vertebrates . Publishing house Dr. Friedrich Pfeil, Munich 2007, ISBN 978-3-89937-072-0 , pp. 11, 51.
- ^ Simon Conway Morris, Jean-Bernard Caron: Pikaia gracilens Walcott, a stem-group chordate from the Middle Cambrian of British Columbia . In: Biological Reviews . No. 87, 2012, doi : 10.1111 / j.1469-185X.2012.00220.x , p. 480.
- ↑ Vladimir V. Missarzhevsky: Conodont-shaped organisms from the Precambrian-Cambrian boundary strata of the Siberian Platform and Kazakhstan. In: IT Zhuravleva (Ed.): Palaeontological and Biostratigraphic Problems in the Lower Cambrian of Siberia and the Far East . Nauka, Novosibirsk 1973, p. 53.
- ↑ Michael J. Benton: Paleontology of the vertebrates . Publishing house Dr. Friedrich Pfeil, Munich 2007, ISBN 978-3-89937-072-0 , pp. 57-59.
- ↑ a b Zdzislaw Belka: Conodonta . In: Wilfried Westheide, Gunde Rieger (Ed.): Special Zoology Part 2: Vertebrate or cranial animals . Springer-Verlag, Berlin / Heidelberg 2015, ISBN 978-3-642-55435-3 , p. 175.
- ↑ Philip CJ Donoghue, Joseph N. Keating: Early vertebrate evolution . In: Palaeontology . No. 57, 2014, doi : 10.1111 / pala.12125 , p. 883.
- ↑ Michael J. Benton: Paleontology of the vertebrates . Publishing house Dr. Friedrich Pfeil, Munich 2007, ISBN 978-3-89937-072-0 , pp. 11, 19, 21, 51, 59.
- ↑ Michael J. Benton: Paleontology of the vertebrates . Publishing house Dr. Friedrich Pfeil, Munich 2007, ISBN 978-3-89937-072-0 , p. 20.
- ↑ Jun-Yuan Chen, Di-Ying Huang, Qing-Qing Peng, Hui-Mei Chi, Xiu-Qiang Wang, Man Feng: The first tunicate from the Early Cambrian of South China . In: Proceedings of the National Academy of Sciences . No. 100, 2003, doi : 10.1073 / pnas.1431177100 , pp. 8314-8316.
- ↑ Michael J. Benton: Paleontology of the vertebrates . Publishing house Dr. Friedrich Pfeil, Munich 2007, ISBN 978-3-89937-072-0 , pp. 13, 20.
- ^ Alfred Goldschmid: Deuterostomia . In: Wilfried Westheide, Gunde Rieger (ed.): Special Zoology Part 1: Protozoa and invertebrates . Springer-Verlag, Berlin / Heidelberg 2013, ISBN 978-3-642-34695-8 , p. 795.
- ↑ Michael J. Benton: Paleontology of the vertebrates . Publishing house Dr. Friedrich Pfeil, Munich 2007, ISBN 978-3-89937-072-0 , p. 21.
- ^ Alfred Goldschmid: Deuterostomia . In: Wilfried Westheide, Gunde Rieger (Ed.): Special Zoology Part 1: Protozoa and Invertebrates . Springer-Verlag, Berlin / Heidelberg 2013, ISBN 978-3-642-34695-8 , p. 786.
- ↑ Systematics according to (1a) Diego C. García-Bellido, Michael SY Lee, Gregory D. Edgecombe, James B. Jago, James G. Gehling, John R. Paterson: A new vetulicolian from Australia and its bearing on the chordate affinities of to enigmatic Cambrian group . In: BMC Evolutionary Biology . No. 14, 2014, doi : 10.1186 / s12862-014-0214-z , p. 8, updated with (1b) Jian Han, Simon Conway Morris, Qiang Ou, Degan Shu, Hai Huang: Meiofaunal deuterostomes from the basal Cambrian of Shaanxi (China) . In: Nature . Volume 542, 2017, doi : 10.1038 / nature21072 , p. 230; (2) Philip CJ Donoghue, Joseph N. Keating: Early vertebrate evolution . In: Palaeontology . No. 57, 2014, doi : 10.1111 / pala.12125 , p. 880; (3) Min Zhu, Xiaobo Yu, Per Erik Ahlberg, Brian Choo, Jing Lu, Tuo Qiao, Qingming Qu, Wenjin Zhao, Liantao Jia, Henning Blom, You'an Zhu: A Silurian placoderm with osteichthyan-like marginal jaw bones . In: Nature . Volume 502, 2013, doi : 10.1038 / nature12617 , p. 192; (4) Sam Giles, Matt Friedman, Martin D. Brazeau: Osteichthyan-like cranial conditions in an Early Devonian stem gnathostome . In: Nature . Volume 520, 2015, doi : 10.1038 / nature14065 , p. 84; (5) Thomas J. Near, Alex Dornburg, Masayoshi Tokita, Dai Suzuki, Matthew C. Brandley, Matt Friedman: Boom and Bust: ancient and recent Diversification in Bichirs (Polypteridae: Actinopterygii), a relictual lineage of ray-finned Fishes . In: evolution . Volume 68, 2014, doi : 10.1111 / evo.12323 , p. 1020; (6) Michael J. Benton: Paleontology of the vertebrates . Publishing house Dr. Friedrich Pfeil, Munich 2007, ISBN 978-3-89937-072-0 , pp. 21, 53, 416-418.
- ↑ Michael J. Benton: Paleontology of the vertebrates . Publishing house Dr. Friedrich Pfeil, Munich 2007, ISBN 978-3-89937-072-0 , p. 68.
- ↑ Benedict King, Tuo Qiao, Michael SY Lee, Min Zhu: Bayesian Morphological Clock Methods Resurrect Placoderm Monophyly and Reveal Rapid Early Evolution in Jawed Vertebrates . In: Systematic Biology . Volume 66, 2016, doi : 10.1093 / sysbio / syw107 , p. 514.
- ^ Martin D. Brazeau, Matt Friedman, The origin and early phylogenetic history of jawed vertebrates . In: Nature . Volume 520, 2015, doi : 10.1038 / nature14438 , p. 496.
- ↑ Lauren Sallan, Matt Friedman, Robert S. Sansom, Charlotte M. Bird, Ivan J. Sansom: The nearshore cradle of early vertebrate diversification . In: Science . Volume 362, 2018, doi : 10.1126 / science.aar3689 , p. 460.
- ^ Gavin C. Young: Placoderms (Armored Fish): Dominant Vertebrates of the Devonian Period . In: Annual Review of Earth and Planetary Sciences . Volume 38, 2010, doi : 10.1146 / annurev-earth-040809-152507 , p. 527.
- Jump up ↑ John G. Maisey, Randall Miller, Alan Pradel, John SS Denton, Allison Bronson, Philippe Janvier: Pectoral morphology in Doliodus: bridging the 'acanthodian'-chondrichthyan divide . In: American Museum Novitates . No. 3875, 2017, doi : 10.1206 / 3875.1 , p. 2, 12.
- ^ Gavin F. Hanke, Mark VH Wilson: New teleostome fishes and acanthodian systematics . In: Gloria Arratia, Mark VH Wilson, Richard Cloutier (eds.): Recent Advances in the Origin and Early Radiation of Vertebrates . Publishing house Dr. Friedrich Pfeil, Munich 2004, ISBN 978-3-899-37052-2 , p. 189.
- ↑ Jason D. Pardo, Bryan J. Small, and Adam K. Huttenlocker: Stem caecilian from the Triassic of Colorado sheds light on the origins of Lissamphibia . In: Proceedings of the National Academy of Sciences . Volume 114, 2017, doi : 10.1073 / pnas.1706752114 , p. E5389
- ^ David S. Berman: Diadectomorphs, Amniotes or Not? In: Spencer G. Lucas et al. (Ed.): The Carboniferous-Permian Transition . New Mexico Museum of Natural History & Science, Albuquerque 2013, ISSN 1524-4156 , p. 33.
- ↑ Zhikun Gai, Philip CJ Donoghue, Min Zhu, Philippe Janvier, Marco Stampanoni: Fossil jawless fish from China foreshadows early jawed vertebrate anatomy . In: Nature . Volume 476, 2011, doi : 10.1038 / nature10276 , p. 324.
- ↑ Michael J. Benton: Paleontology of the vertebrates . Publishing house Dr. Friedrich Pfeil, Munich 2007, ISBN 978-3-89937-072-0 , p. 53.
- ^ Robert W. Gess, Michael I. Coates, Bruce S. Rubidge: A lamprey from the Devonian period of South Africa . In: Nature . Volume 443, 2006, doi : 10.1038 / nature05150 , p. 981.
- ^ Philippe Janvier, Richard Lund: Hardistiella montanensis n. Gen. Et sp. (Petromyzontida) from the Lower Carboniferous of Montana, with remarks on the affinities of the lampreys . In: Journal of Vertebrate Paleontology . Volume 02, 1983, doi : 10.1080 / 02724634.1983.10011943 , p. 407.
- ↑ a b c d e f Milton Hildebrand, George E. Goslow: Comparative and functional anatomy of the vertebrates . Springer-Verlag, Berlin / Heidelberg 2004, ISBN 3-540-00757-1 , p. 167.
- ↑ Milton Hildebrand, George E. Goslow: Comparative and functional anatomy of the vertebrates . Springer-Verlag, Berlin / Heidelberg 2004, ISBN 3-540-00757-1 , p. 163.
- ↑ Michael J. Benton: Paleontology of the vertebrates . Publishing house Dr. Friedrich Pfeil, Munich 2007, ISBN 978-3-89937-072-0 , pp. 51-52.
- ↑ Martin S. Fischer: Musculoskeletal system . In: Wilfried Westheide, Gunde Rieger (ed.): Special Zoology Part 2: Vertebrate or skull animals . Springer-Verlag, Berlin / Heidelberg 2015, ISBN 978-3-642-55435-3 , pp. 49-50.
- ↑ a b c Dietrich Starck: Comparative Anatomy of the Vertebrates Volume 2 . Springer-Verlag, Berlin / Heidelberg 1979, ISBN 3-540-09156-4 , p. 46.
- ↑ a b Dietrich Starck: Comparative Anatomy of the Vertebrates Volume 2 . Springer-Verlag, Berlin / Heidelberg 1979, ISBN 3-540-09156-4 , p. 57.
- ↑ Dietrich Starck: Comparative Anatomy of the Vertebrates Volume 2 . Springer-Verlag, Berlin / Heidelberg 1979, ISBN 3-540-09156-4 , p. 48.
- ↑ David Bardack: First Fossil Hagfish (Myxinoidea): A record from the Pennsylvanian of Illinois . In: Science . Volume 254, 1991, doi : 10.1126 / science.254.5032.701 , p. 701.
- ^ Cécile Poplin, Daniel Sotty, Philippe Janvier: A hagfish (Craniata, Hyperotreti) from the Late Carboniferous Conservate Deposit of Montceau-les-Mines (Allier, France) . In: Comptes Rendus de l'Académie des Sciences - Series IIA - Earth and Planetary Science . Volume 332, 2001, doi : 10.1016 / S1251-8050 (01) 01537-3 , p. 345.
- ↑ Kinya G. Ota, Satoko Fujimoto, Yasuhiro Oisi, Shigeru Kuratani: Identification of vertebra-like elements and their possible differentiation from sclerotomes in the hagfish . In: Nature Communications . Volume 2, 2011, Article 373, doi : 10.1038 / ncomms1355 , p. 1.
- ^ Gunde Rieger, Wolfgang Maier: Cyclostomata . In: Wilfried Westheide, Gunde Rieger (Ed.): Special Zoology Part 2: Vertebrate or cranial animals . Springer-Verlag, Berlin / Heidelberg 2015, ISBN 978-3-642-55435-3 , p. 181.
- ↑ Milton Hildebrand, George E. Goslow: Comparative and functional anatomy of the vertebrates . Springer-Verlag, Berlin / Heidelberg 2004, ISBN 3-540-00757-1 , p. 136.
- ^ Gerhard Mickoleit: Phylogenetic systematics of vertebrates . Publishing house Dr. Friedrich Pfeil, Munich 2004, ISBN 3-89937-044-9 , p. 33.
- ^ M. Paul Smith, Ivan I. Sansom: The affinity of Anatolepis . In: Geobios . Volume 28, 1995, doi : 10.1016 / S0016-6995 (95) 80088-3 , p. 61.
- ↑ EL Jordan, PS Verma: Chordate Zoology . S. Chand & Company, New Delhi 2014, ISBN 978-8-121-91639-4 , p. 10.
- ↑ ZhiKun Gai, Min Zhu: The origin of the vertebrate jaw: Intersection between developmental biology-based model and fossil evidence . In: Chinese Science Bulletin . Volume 57, 2012, doi : 10.1007 / s11434-012-5372-z , p. 3819.
- ↑ Zhikun Gai, Philip CJ Donoghue, Min Zhu, Philippe Janvier, Marco Stampanoni: Fossil jawless fish from China foreshadows early jawed vertebrate anatomy. In: Nature . Volume 476, 2011, doi : 10.1038 / nature10276 , pp. 324, 326.
- ↑ Junqing Wang: The Antiarchi from Early Silurian of Hunan . In: Vertebrata PalAsiatica . Volume 29, 1991, p. 240 ( PDF file ).
- ↑ Guo-Rui Zhang, Shi-Tao Wang, Jun-Qing Wang, Nian-Zhong Wang, Min Zhua: A basal antiarch (placoderm fish) from the Silurian of Qujing, Yunnan, China . In: Palaeoworld . Volume 19, 2010, doi : 10.1016 / j.palwor.2009.11.006 , p. 129.
- ↑ Ivan J. Sansom, Neil S. Davies, Michael I. Coates, Robert S. Nicoll, Alex Ritchie: Chondrichthyan-like scales from the Middle Ordovician of Australia . In: Palaeontology . Volume 55, 2012, doi : 10.1111 / j.1475-4983.2012.01127.x , p. 243.
- ↑ Min Zhu, Per E. Ahlberg, Zhaohui Pan, Youan Zhu, Tuo Qiao, Wenjin Zhao, Liantao Jia, Jing Lu: A Silurian maxillate placoderm illuminates jaw evolution . In: Science . Volume 354, 2016, doi : 10.1126 / science.aah3764 , p. 334.
- ↑ Min Zhu, Xiaobo Yu, Per Erik Ahlberg, Brian Choo, Jing Lu, Tuo Qiao, Qingming Qu, Wenjin Zhao, Liantao Jia, Henning Blom, You'an Zhu: A Silurian placoderm with osteichthyan-like marginal jaw bones . In: Nature . Volume 502, 2013, doi : 10.1038 / nature12617 , p. 188.
- ↑ Sam Giles, Matt Friedman, Martin D. Brazeau: Osteichthyan-like cranial conditions in an Early Devonian stem gnathostome . In: Nature . Volume 520, 2015, doi : 10.1038 / nature14065 , p. 82.
- ^ Zhu Min: Early Silurian sinacanths (Chondrichthyes) from China . In: Palaeontology . Volume 41, 1998, p. 157 ( PDF file ).
- ↑ Tiiu Märss, Pierre-Yves Gagnier: A New Chondrichthyan from the Wenlock, Lower Silurian, of Baillie-Hamilton Iceland, the Canadian Arctic . In: Journal of Vertebrate Paleontology . Volume 21, 2001, doi : 10.1671 / 0272-4634 (2001) 021 [0693: ANCFTW] 2.0.CO; 2 , p. 693.
- Jump up ↑ John G. Maisey, Randall Miller, Alan Pradel, John SS Denton, Allison Bronson, Philippe Janvier: Pectoral morphology in Doliodus: bridging the 'acanthodian'-chondrichthyan divide . In: American Museum Novitates . No. 3875, 2017, doi : 10.1206 / 3875.1 , p. 13
- ^ Hector Botella, Henning Blom, Markus Dorka, Per Erik Ahlberg, Philippe Janvier: Jaws and teeth of the earliest bony fishes . In: Nature . Volume 448, 2007, doi : 10.1038 / nature05989 , p. 583.
- ↑ Jing Lu, Sam Giles, Matt Friedman, Jan L. den Blaauwen, Min Zhu: The Oldest Actinopterygian Highlights the Cryptic Early History of the Hyperdiverse Ray-Finned Fishes . In: Current Biology . Volume 26, 2016, doi : 10.1016 / j.cub.2016.04.045 , p. 1602.
- ↑ Min Zhu, Wenjin Zhao, Liantao Jia, Jing Lu, Tuo Qiao, Qingming Qu: The oldest articulated osteichthyan reveals mosaic gnathostome characters . In: Nature . Volume 458, 2009, doi : 10.1038 / nature07855 , p. 469.
- ↑ Xiaobo Yu: A new porolepiform-like fish, Psarolepis romeri, gen. Et sp. nov. (Sarcopterygii, Osteichthyes) from the Lower Devonian of Yunnan, China . In: Journal of Vertebrate Paleontology . Volume 18, 1998, doi : 10.1080 / 02724634.1998.10011055 , p. 261.
- ↑ Brian Choo, Min Zhu, Qingming Qu, Xiaobo Yu, Liantao Jia, Wenjin Zhao: A new osteichthyan from the late Silurian of Yunnan, China . In: Public Library of Science ONE . Volume 12, 2017, doi : 10.1371 / journal.pone.0170929 , pp. 1, 9.
- ↑ Robert Denison: Placodermi . Gustav Fischer Verlag, Stuttgart / New York 1978, ISBN 0-89574-027-3 , p. 7.
- ↑ Arno Hermann Müller: Textbook of Paleozoology Volume III Vertebrates Part 1 Fish - Amphibians . Gustav Fischer Verlag, Jena 1985, ISBN 3-334-00222-5 , p. 184.
- ↑ Dietrich Starck: Comparative Anatomy of the Vertebrates Volume 2 . Springer-Verlag, Berlin / Heidelberg 1979, ISBN 3-540-09156-4 , pp. 46, 50.
- ^ Rüdiger Wehner, Walter Gehring: Zoologie . Georg Thieme Verlag, Stuttgart / New York 2013, ISBN 978-3-13-367425-6 , p. 663.
- ↑ Dietrich Starck: Comparative Anatomy of the Vertebrates Volume 2 . Springer-Verlag, Berlin / Heidelberg 1979, ISBN 3-540-09156-4 , p. 47.
- ^ Lothar fights, Rolf Kittel, Johannes Klapperstück: Guide to the anatomy of vertebrates . Gustav Fischer Verlag, Jena / Stuttgart / New York 1993, ISBN 3-334-60454-3 , pp. 45-46.
- ↑ Carole J. Burrow, Dirk C. Hovestadt, Maria Hovestadt-Euler, Susan Turner, Gavin C. Young: New Information on the Devonian Shark Mcmurdodus, based on material from western Queensland, Australia. In: Acta Geologica Polonica . Volume 58, 2008, p. 155 ( PDF file ).
- ↑ Dietrich Starck: Comparative Anatomy of the Vertebrates Volume 2 . Springer-Verlag, Berlin / Heidelberg 1979, ISBN 3-540-09156-4 , p. 52.
- ↑ Michael J. Benton: Paleontology of the vertebrates . Publishing house Dr. Friedrich Pfeil, Munich 2007, ISBN 978-3-89937-072-0 , p. 181.
- ↑ a b Dietrich Starck: Comparative Anatomy of the Vertebrates Volume 2 . Springer-Verlag, Berlin / Heidelberg 1979, ISBN 3-540-09156-4 , p. 53.
- ↑ Milton Hildebrand, George E. Goslow: Comparative and functional anatomy of the vertebrates . Springer-Verlag, Berlin / Heidelberg 2004, ISBN 3-540-00757-1 , pp. 167-168.
- ↑ Dietrich Starck: Comparative Anatomy of the Vertebrates Volume 2 . Springer-Verlag, Berlin / Heidelberg 1979, ISBN 3-540-09156-4 , pp. 50-52.
- ↑ Sam Giles, Guang-Hui Xu, Thomas J. Near, Matt Friedman: Early members of 'living fossil' lineage imply later origin of modern ray-finned fishes . In: Nature . Volume 548, 2017, doi : 10.1038 / nature23654 , pp. 265, 267.
- ↑ Sam Giles, Guang-Hui Xu, Thomas J. Near, Matt Friedman: Early members of 'living fossil' lineage imply later origin of modern ray-finned fishes . In: Nature . Volume 548, 2017, doi : 10.1038 / nature23654 , pp. 264–265.
- ^ David B. Wake: The Endoskeleton . In: Marvalee H. Wake (Ed.): Hyman's Comparative Vertebrate Anatomy . University of Chicago Press, Chicago / London 1979, ISBN 0-226-87013-8 , p. 204.
- ↑ Didier B. Dutheil: The first articulated fossil Cladistian: Serenoichthys kemkemensis, gen. Et sp. nov., from the Cretaceous of Morocco . In: Journal of Vertebrate Paleontology . Volume 19, 1999, doi : 10.1080 / 02724634.1999.10011138 , p. 244.
- ↑ Dietrich Starck: Comparative Anatomy of the Vertebrates Volume 2 . Springer-Verlag, Berlin / Heidelberg 1979, ISBN 3-540-09156-4 , p. 55.
- ^ Gerhard Mickoleit: Phylogenetic systematics of vertebrates . Publishing house Dr. Friedrich Pfeil, Munich 2004, ISBN 3-89937-044-9 , pp. 90-91.
- ↑ Volker Storch, Ulrich Welsch: Systematic Zoology . Spektrum Akademischer Verlag, Heidelberg / Berlin 2004, ISBN 3-8274-1112-2 , p. 590.
- ↑ Lauren C. Sallan: Major issues in the origins of ray-finned fish (Actinopterygii) biodiversity . In: Biological Reviews . Volume 89, 2014, doi : 10.1111 / brv.12086 , pp. 963-966.
- ↑ a b Lothar fights, Rolf Kittel, Johannes Klapperstück: Guide to the anatomy of vertebrates . Gustav Fischer Verlag, Jena / Stuttgart / New York 1993, ISBN 3-334-60454-3 , p. 47.
- ↑ Dietrich Starck: Comparative Anatomy of the Vertebrates Volume 2 . Springer-Verlag, Berlin / Heidelberg 1979, ISBN 3-540-09156-4 , p. 56.
- ^ Roberta L. Paton, Tim R. Smithson, Jennifer A. Clack: An amniote-like skeleton form the Early Carboniferous of Scotland . In: Nature . Volume 398, 1999, doi : 10.1038 / 19071 , p. 508.
- ↑ Milton Hildebrand, George E. Goslow: Comparative and functional anatomy of the vertebrates . Springer-Verlag, Berlin / Heidelberg 2004, ISBN 3-540-00757-1 , p. 169.
- ^ Lothar fights, Rolf Kittel, Johannes Klapperstück: Guide to the anatomy of vertebrates . Gustav Fischer Verlag, Jena / Stuttgart / New York 1993, ISBN 3-334-60454-3 , p. 49.
- ↑ Dietrich Starck: Comparative Anatomy of the Vertebrates Volume 2 . Springer-Verlag, Berlin / Heidelberg 1979, ISBN 3-540-09156-4 , pp. 65-66.
- ^ Lothar fights, Rolf Kittel, Johannes Klapperstück: Guide to the anatomy of vertebrates . Gustav Fischer Verlag, Jena / Stuttgart / New York 1993, ISBN 3-334-60454-3 , p. 46.
- ↑ Volker Storch, Ulrich Welsch: Systematic Zoology . Spektrum Akademischer Verlag, Heidelberg / Berlin 2004, ISBN 3-8274-1112-2 , p. 511.
- ↑ Michael J. Benton: Paleontology of the vertebrates . Publishing house Dr. Friedrich Pfeil, Munich 2007, ISBN 978-3-89937-072-0 , p. 107.
- ↑ Dietrich Starck: Comparative Anatomy of the Vertebrates Volume 2 . Springer-Verlag, Berlin / Heidelberg 1979, ISBN 3-540-09156-4 , p. 63.
- ^ Gerhard Mickoleit: Phylogenetic systematics of vertebrates . Publishing house Dr. Friedrich Pfeil, Munich 2004, ISBN 3-89937-044-9 , p. 255.
- ^ Gerhard Mickoleit: Phylogenetic systematics of vertebrates . Publishing house Dr. Friedrich Pfeil, Munich 2004, ISBN 3-89937-044-9 , pp. 254, 268.
- ↑ Jason S. Anderson, Robert R. Reisz, Diane Scott, Nadia B. Fröbisch, Stuart S. Sumida: A stem batrachian from the Early Permian of Texas and the origin of frogs and salamanders . In: Nature . Volume 453, 2008, doi : 10.1038 / nature06865 , p. 515.
- ^ Michael Laurin: Recent progress in paleontological methods for dating the Tree of Life . In: Frontiers in Genetics . Volume 3, 2012, doi : 10.3389 / fgene.2012.00130 , p. 12.
- ^ A b Robert L. Carroll, Andrew Kuntz, and Kimberley Albright: Vertebral development and amphibian evolution . In: Evolution & Development . Volume 1, 1999, doi : 10.1111 / j.1525-142X.1999.t01-2-.x , pp. 36, 39, 43.
- ^ Robert L. Carroll, Andrew Kuntz, and Kimberley Albright: Vertebral development and amphibian evolution . In: Evolution & Development . Volume 1, 1999, doi : 10.1111 / j.1525-142X.1999.t01-2-.x , p. 37.
- ↑ Ralf Werneburg: Timeless design - colored pattern of skin in Early Permian branchiosaurids (Temnospondyli: Dissorophoidea) . In: Journal of Vertebrate Paleontology . Volume 27, 2007, doi : 10.1671 / 0272-4634 (2007) 27 [1047: TDCPOS] 2.0.CO; 2 , pp. 1048-1051.
- ^ Robert L. Carroll, Andrew Kuntz, and Kimberley Albright: Vertebral development and amphibian evolution . In: Evolution & Development . Volume 1, 1999, doi : 10.1111 / j.1525-142X.1999.t01-2-.x , pp. 38-39,47.
- ↑ Maximilian J. Telford, Graham E. Budd, and Herve 'Philippe: Phylogenomic Insights into Animal Evolution . In: Current Biology . Volume 25, 2015, doi : 10.1016 / j.cub.2015.07.060 , p. R884.
- ^ Antonella Lauri, Thibaut Brunet, Mette Handberg-Thorsager, Antje HL Fischer, Oleg Simakov, Patrick RH Steinmetz, Raju Tomer, Philipp J. Keller, Detlev Arendt: Development of the annelid axochord: Insights into notochord evolution . In: Science . Volume 345, 2014, doi : 10.1126 / science.1253396 , p. 1365.
- ↑ Andreas Hejnol, Christopher J. Lowe: Animal Evolution: Stiff or Squishy Notochord Origins? In: Current Biology . Volume 24, 2014, doi : 10.1016 / j.cub.2014.10.059 , pp. 1131–1133.
- ↑ Dietrich Starck: Comparative Anatomy of the Vertebrates Volume 1 . Springer-Verlag, Berlin / Heidelberg 1978, ISBN 3-540-08889-x , p. 48.
- ↑ Hermann Augener: Ceylon polychetes . In: Jenaische Zeitschrift für Naturwissenschaft . Volume 62, 1926, p. 445.
- ^ Hermann Friedrich Stannius: Textbook of Comparative Anatomy • Second part. Vertebrates. Verlag von Veit und Company, Berlin 1846, p. 31.
- ↑ Volker Storch, Ulrich Welsch: Kükenthal zoological internship . Springer-Verlag, Berlin / Heidelberg 2014, ISBN 978-3-642-41936-2 , pp. 318, 322.
- ↑ Jan Boeke: The money roll stage of the vertebrate chorda and the skeleton of the oral cirrus of Branchiostoma lanceolatum, and its cytomechanical significance . In: Anatomischer Anzeiger . Volume 33, 1908, pp. 541-556, 574-580.
- ↑ Dietrich Starck: Comparative Anatomy of the Vertebrates Volume 2 . Springer-Verlag, Berlin / Heidelberg 1979, ISBN 3-540-09156-4 , pp. 35-36.
- ↑ Dietrich Starck: Comparative Anatomy of the Vertebrates Volume 2 . Springer-Verlag, Berlin / Heidelberg 1979, ISBN 3-540-09156-4 , pp. 36, 45.
- ^ Ondine Cleaver, Daniel W. Seufert, Paul A. Krieg: Endoderm patterning by the notochord: development of the hypochord in Xenopus . In: Development . Volume 127, 2000, pp. 869, 877 ( PDF file ).
- ↑ Krzysztof A. Tomaszewski, Karolina Saganiak, Tomasz Gładysz, Jerzy A. Walocha: The biology behind the human intervertebral disc and its endplates . In: Folia Morphologica . Volume 74, 2015, doi : 10.5603 / FM.2015.0026 , pp. 158–159.
- ↑ Thomas Liebscher, Mathias Haefeli, Karin Wuertz, Andreas G. Nerlich, Norbert Boos: Age-Related Variation in Cell Density of Human Lumbar Intervertebral Disc . In: Spine . Volume 36, 2011, doi : 10.1097 / BRS.0b013e3181cd588c , p. 157.
- ^ Radivoj V. Krstic: Illustrated Encyclopedia of Human Histology . Springer-Verlag, Berlin / Heidelberg / New York / Tokyo 1984, ISBN 3-540-13142-6 , p. 295.
- ↑ Wolfgang Kummer, Ulrich Welsch: musculoskeletal system . In: Ulrich Welsch, Thomas Deller, Wolfgang Kummer: Textbook Histology . Urban & Fischer Verlag, Munich 2014, ISBN 978-3-437-44433-3 , pp. 305-307, 309.
- ^ Luiz Carlos U. Junqueira, José Carneiro: Histology . Springer Medizin Verlag, Heidelberg 2005, ISBN 3-540-21965-X , pp. 88-89.
- ^ Arthur Willey: Remarks on some Recent Work on the Protochorda, with a Condensed Account of some Fresh Observations on the Enteropneusta. In: Journal of Cell Science . Volume 42, 1899, p. 235 ( PDF file ).