Pentose phosphate route
Parent |
Glucose breakdown NADPH regeneration |
Subordinate |
Oxidative route Reductive route |
Gene Ontology |
---|
QuickGO |
The pentose phosphate pathway (also hexose monophosphate cycle, hexose monophosphate shunt or 6-phosphogluconate pathway) is a metabolic pathway that occurs in most living things. It represents a way of utilizing carbohydrates , for example glucose , in which the reducing agent NADPH is formed.
It is also used to convert various carbohydrates. These processes take place in the cytosol of the cell, and in plants also in the chloroplasts .
General process and products
The pentose phosphate pathway can be divided into an oxidative, irreversible and a non-oxidative (reductive), reversible section. However, both paths do not have to follow one another. For example, in the course of CO 2 assimilation in plants ( Calvin cycle ), only the reductive route is taken.
In the pentose phosphate route, ribulose-5-phosphate is formed from D- glucose in the oxidative route, with NADPH being obtained. Ribulose-5-phosphate (a pentose phosphate) can be converted into metabolites of glycolysis or serve as a basic building block for the biosynthesis of nucleotides ( DNA , RNA ) and coenzymes ( ATP , coenzyme A , NAD , FAD ). NADPH is used as a reducing agent for anabolism and is used to maintain a reducing environment in the cytoplasm . In contrast to NADH, NADPH is not used to generate energy in the respiratory chain . Other possibilities of NADPH synthesis include the citrate shuttle and the light reactions during photosynthesis .
In the non-oxidative, reversible part, different carbohydrates can be converted into one another. For example, the pentose (C 5 sugar) resulting from the oxidative branch can be transformed into metabolites of glycolysis . C 3 sugar ( trioses ), C 4 sugar ( tetroses ), C 5 sugar (pentoses), C 6 sugar ( hexoses ) and a C 7 sugar ( heptose ) occur as intermediate products .
Oxidative part
As in glycolysis , the first step is the glucokinase reaction, in which α- D- glucose is phosphorylated to α- D- glucose-6-phosphate ( 1 ) with consumption of ATP.
The glucose-6-phosphate dehydrogenase (G6PDH) forms to generate NADPH and H + 6-Phosphoglucono-δ-lactone ( 2 ), an intramolecular ester. It is highly specific for glucose-6-phosphate and NADP + , but not for NAD + . It is an oxidation at the C 1 atom of glucose. The equilibrium of this reaction is far on the side of the products. Also, the hexose-6-phosphate dehydrogenase (X6PDH) can catalyze this reaction. However, it shows a lower specificity for glucose and can also oxidize other hexoses. In many cells, however, this catalyzes the first step of the pentose phosphate pathway.
Next, 6-phosphogluconolactonase converts 6-phosphoglucono-δ-lactone to 6-phosphogluconate ( 3 ). This is then oxidized and decarboxylated to ribulose-5-phosphate ( 4 ) using 6-phosphogluconate dehydrogenase , with NADPH, H + and CO 2 being formed. This means that an oxidation takes place at the C3 atom.
The overall balance for the oxidative part of the pentose phosphate pathway, based on glucose-6-phosphate, is thus:
Non-oxidative part

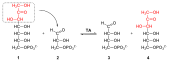
In the reversible part of the pentose phosphate pathway, the C 4 , C 5 , C 6 and one C 7 sugars are transformed into one another; it does not serve to generate further reduction equivalents. These reactions are catalyzed by two enzymes. A transketolase transfers two and a transaldolase three carbon units . The transfer of carbon atoms by transaldolase and transketolase does not consume ATP and in the case of transketolase is only dependent on the cofactor thiamine pyrophosphate (TPP, from vitamin B1 ).
As an example, the figure shows a possible fate of ribulose-5-phosphate, which arose during the oxidative path of the pentose phosphate path.
Ribulose-5-phosphate is isomerized to ribose-5-phosphate via the ribose-5-phosphate isomerase , but it can also be rearranged to xylulose-5-phosphate (X5P) with the help of the ribulose phosphate-3-epimerase . Both products take part in the following reactions. A transketolase then transfers a C 2 unit from xylulose-5-phosphate to ribose-5-phosphate, so that glyceraldehyde-3-phosphate (GAP) is formed from the former and sedoheptulose-7-phosphate (S7P) from the latter . When a C 3 unit is transferred to GAP in S7P , erythrose-4-phosphate (E4P) and fructose-6-phosphate are formed . This reaction is catalyzed by a transaldolase. Finally, a ketolase transfers two carbon units from another molecule of X5P to E4P, so that finally another molecule of fructose-6-phosphate and GAP are formed.
Balance sheet
With three molecules of ribulose-5-phosphate, the reductive path of the pentose phosphate path results in the following balance:
If the oxidative pathway is taken into account, two molecules of fructose-6-phosphate and one molecule of glyceraldehyde-3-phosphate are formed from three molecules of glucose-6-phosphate under oxidation with NADP + and decarboxylation:
Pentose phosphate reductive route
Glyceraldehyde-3-phosphate is formed in the course of the Calvin cycle. Here, five molecules are converted into three molecules of ribulose 5-phosphate using the enzymes from gluconeogenesis ( triosephosphate isomerase , aldolase , fructose-1,6-bisphosphatase ) and transketolases and aldolases. In the figure above, this corresponds to the path from right to left.
In the literature, this regeneration is also referred to as the reductive pentose phosphate route .
Variations
The pentose phosphate route produces reducing agents in the form of NADPH, while glycolysis produces NADH. If, for example, NADPH and pentoses are increasingly used, glucose flows into this metabolic pathway. This is especially the case in tissues where fatty acid or steroid synthesis takes place. To generate NADH and ATP, the intermediates of the pentose phosphate pathway can flow back into the glycolysis. The pentose phosphate pathway can thus be viewed as a molecular hub, in which the different needs of the cell for NADPH, riboses, ATP, NADH and pyruvate are addressed. He thus links the anabolic and catabolic glucose metabolism. Various cases are discussed below:
Much NADPH should be generated
In the event that as many NADPH molecules as possible are needed, the following variant of the pentose phosphate route can be used (see figure):
First, six molecules of glucose-6-phosphate are broken down into six molecules of ribulose-5-phosphate via the oxidative part of the PP pathway. This creates 12 molecules of NADPH. Ribulose-5-phosphate can then - as shown - be transformed into five molecules of fructose-6-phosphate via several interconversions in the reductive part of the path. This takes place with the involvement of the enzymes of gluconeogenesis. Since these are in equilibrium with glucose-6-phosphate, they flow back into the cycle. The balance sheet is therefore:
In the overall balance, one molecule of glucose-6-phosphate is totally oxidized to six molecules of CO 2 , resulting in 12 molecules of NADPH:
However, this variant of the pentose phosphate pathway, in which only NADPH is produced, occurs to a rather small extent in mammalian cells. In the literature, this complete oxidation of glucose is also referred to as the “oxidative pentose phosphate route” or, according to its discoverers, the “Warburg-Dickens-Horecker degradation route”.
Ribose-5-phosphate is required
Cells in the bone marrow , skin , intestinal mucosa and tumor cells divide quickly and consequently require a lot of DNA and RNA. The construction of DNA and RNA requires many riboses. In an alternative to the PP route, the generation of ribose-5-phosphate can be accentuated (see figure).
In the example above, six molecules of ribose-5-phosphate are produced from five molecules of glucose-6-phosphate. Here, the oxidative part of the PP route is bypassed, only conversions by ketolases and aldolases take place. However, a molecule of ATP is also consumed in this process.
Balance:
NADH, NADPH and ATP are needed
In the event that the cell would like to generate energy from the breakdown of glucose in addition to NADPH, a combination of the PP route and glycolysis can be used (see figure).
In the oxidative part of the PP pathway, three molecules of glucose-6-phosphate are oxidatively broken down into three molecules of ribulose-5-phosphate, which results in six molecules of NADPH. Ribulose-5-phosphate is then converted into five GAP molecules via various reactions. Although two molecules of ATP are consumed here, GAP is metabolized to pyruvate in the further sequence of glycolysis. This creates two molecules of ATP and one molecule of NADH per pyruvate molecule.
The overall balance results in:
regulation
The availability of the substrate in the non-oxidative part of the pentose phosphate pathway is controlled by the steady state and metabolic needs of the cell (see section Variations). In the oxidative part of the pathway, glucose-6-phosphate dehydrogenase, the key enzyme, represents the main regulatory point. The competitive inhibition of the enzyme takes place in the course of an end product inhibition: NADPH and intermediate products of fatty acid synthesis ( acyl-CoA ) inhibit glucose-6-phosphate Dehydrogenase. In contrast, NADP + is an allosteric activator of dehydrogenase.
Occurrence
The enzymes of the pentose phosphate pathway are present in the cytoplasm of almost all cells. Since NADPH is required for the synthesis of fatty acids and steroids, the PP route takes place particularly in liver cells (fatty acid and cholesterol synthesis) and in adipose tissue (fatty acid synthesis). Other tissues in which fatty acids and steroids are synthesized also require NADPH and thus the pentose phosphate pathway. These are, for example, the milk-producing female breast ( glandula mammaria ), the testes , the ovary and the adrenal cortex (steroid synthesis).
In muscle cells, however, the enzymes for this metabolic pathway are largely missing. This is because glucose-6-phosphate is metabolized there in the glycolysis and citric acid cycle for energy production.
Dependence of erythrocytes on the pentose phosphate pathway
Erythrocytes are special because they are completely dependent on the pentose phosphate route. For them it is the only way that reducing agents can be produced in the form of NADPH.
Tissues are at risk of oxidative damage from reactive oxygen species (ROS). Erythrocytes are particularly at risk from the transport of oxygen. ROS, for example hydrogen peroxide (H 2 O 2 ), damage proteins, DNA and cell wall lipids. Either H 2 O 2 is split into water and oxygen by a catalase, or oxidative damage is minimized by the fact that glutathione renders ROS harmless. A glutathione peroxidase can reduce peroxides (see figure) by oxidizing two molecules of glutathione. NADPH acts as a protective reducing agent as it regenerates glutathione. This is catalyzed by glutathione reductase (GSR).
In people with a defect in glucose-6-phosphate dehydrogenase (G6PDH), the erythrocytes are exposed to increased oxidative stress because, unlike other tissues, they do not express hexulose-6-phosphate dehydrogenase, so that a defect has a drastic effect on the pentose phosphate pathway . Too little NADPH is formed, so that more oxidative damage occurs, for example in the membrane. G6PDH deficiency therefore leads to hemolytic anemia .
On the other hand, people with a G6PDH defect have increased protection against malaria . The pathogen Plasmodium falciparum multiplies in the erythrocytes, but is very sensitive to oxidative stress. This leads to a selection advantage: G6PDH deficiency is common in areas where malaria is common.
Erythrocytes have no nucleus and no mitochondria . This means that they can neither operate a citric acid cycle nor need ribose-5-phosphate to produce DNA and RNA. So you could be generating enough antioxidants with the oxidative branch alone. On the one hand, this deprives the energy-supplying glycolysis of the glucose; on the other hand, an osmotic problem would soon arise from the pentose. The non-oxidative branch converts two molecules of xylulose-5-phosphate and one molecule of ribose-5-phosphate into glyceraldehyde-3-phosphate and two molecules of fructose-6-phosphate. These products can use the erythrocytes in glycolysis to generate energy (see section above ).
literature
- Reginald Garrett and Charles M. Grisham: Biochemistry. (International Student Edition). Thomsom Learning Inc .; 3rd edition 2005; ISBN 0-534-41020-0 ; Pp. 725-735
- Thomas M. Devlin (Ed.): Textbook of Biochemistry with Clinical Correlations . Wiley & Sons; 6th edition 2005; ISBN 978-0-471-67808-3 ; Pp. 638-643
- H. Robert Horton, Laurence A. Moran, K. Gray Scrimgeour, Marc D. Perry, J. David Rawn and Carsten Biele (translators): Biochemistry . Pearson Studies; 4th updated edition 2008; ISBN 978-3-8273-7312-0 ; Pp. 490-496
- Werner Müller-Esterl: Biochemistry, an introduction for physicians and natural scientists. Spektrum Akademischer Verlag, 2004, ISBN 3-8274-0534-3 , pp. 522-527.
- Albert Lehninger, Michael Cox and David L. Nelson: Lehninger Principles of Biochemistry . WH Freeman & Co; 5th edition 2008; ISBN 978-0-7167-7108-1 ; Pp. 558-563
Individual evidence
- ↑ Here in the sense of "lateral entry" of products in glycolysis.
- ^ David Nelson, Michael Cox: Lehninger Biochemie . Springer, Berlin; 4th, completely revised u. exp. Edition 2009; ISBN 978-3-540-68637-8 ; P. 1076.
- ↑ a b c d H. Robert Horton, Laurence A. Moran, K. Gray Scrimgeour, Marc D. Perry, J. David Rawn and Carsten Biele (translators): Biochemie . Pearson Studies; 4th updated edition 2008; ISBN 978-3-8273-7312-0 ; Pp. 490-496.
- ^ A b c d Albert Lehninger, Michael Cox, and David L. Nelson: Lehninger Principles of Biochemistry . WH Freeman & Co; 5th edition 2008; ISBN 978-0-7167-7108-1 ; Pp. 558-563.
- ↑ a b c Werner Müller-Esterl: Biochemistry, an introduction for physicians and natural scientists . Spektrum Akademischer Verlag, 2004, ISBN 3-8274-0534-3 , pp. 522-527.
- ↑ Karlsons Biochemie and Pathobiochemie. ISBN 978-3133578158 , p. 258.
- ^ Reginald Garrett and Charles M. Grisham: Biochemistry . (International Student Edition). Thomsom Learning Inc .; 3rd edition 2005; ISBN 0-534-41020-0 ; Pp. 725-735.
- ↑ Thomas M. Devlin (Ed.): Textbook of Biochemistry with Clinical Correlations . Wiley & Sons; 6th edition 2005; ISBN 978-0-471-67808-3 ; Pp. 638-643.
- ↑ Jeremy M. Berg, Lubert Stryer and John L. Tymoczko: Biochemistry . Spectrum Academic Publishing House; 6th edition 2007; ISBN 978-3-8274-1800-5 ; P. 650.