Continuous wave radar
A continuous wave radar is a radar system that differs from a pulse radar in that the transmitter works continuously for the duration of the measurement process . (The term continuous bar comes from the bar symbol in Morse code ).
The various functional principles of continuous wave radars differ mainly in whether and, if so, how the transmitted frequency is modulated . An unmodulated continuous wave radar is also called CW radar (from English continuous wave radar ). With an unmodulated continuous wave radar, distances can only be poorly determined. Rather, it was developed for non-contact speed measurement using the Doppler effect and is used as a motion detector . Modulated continuous wave radar devices , also called FMCW radar (from English frequency modulated continuous wave radar ), are primarily used as distance or altimeters or as navigation radars with a short range on ships and boats.
historical development
Heinrich Hertz already described the reflection of electromagnetic waves on metallic (i.e. electrically conductive) surfaces in his practical experiments on Maxwell's calculations . This was taken up by the German engineer Christian Hülsmeyer , who developed the first radar, which was publicly demonstrated in 1904 from the Rhine bridge in Cologne and subsequently patented in several countries. The function of this radar was a continuous wave radar. It was able to locate an approaching ship at a distance of up to 3 km, even though no sensitive high-frequency components were available at the time. As a non-modulated CW radar, it could not measure distances in principle. Hülsmeyer submitted a second patent, which made it possible to measure a distance with a CW radar from an elevated point of view through the directional effect of the antenna and subsequent trigonometric calculation of the distance from the radiation angle of the antenna. Both inventions, however, did not meet with any interest in the German naval command.
Two electrical engineers, Albert H. Taylor and Leo C. Young of the Naval Research Laboratory (USA), were inspired by Marconi's success in wireless telecommunications. In the autumn of 1922, they used a continuous wave radar to locate a wooden ship on a wavelength of 5 meters for the first time using a bistatic configuration (i.e. separate transmitter and receiver positions). In this experiment, by localizing the transmitter and receiver on the left and right banks of a river, not backscattering was received, but rather the attenuation and scattering of the electromagnetic wave when crossing the ship was recorded. This principle is used in security technology as a barrier radar.
A targeted development of radar technology took place from around 1935 after the military benefit of this technology was recognized. However, because of the range required for locating ships and aircraft, the focus was not on continuous wave radar, but on pulse radar technology . The continuous wave method was partly forgotten again, since with the technical possibilities of the time with a continuous wave radar only short ranges could be achieved.
Initial considerations to enable the height of the ionosphere to be measured using a frequency-modulated continuous wave radar method (FMCW radar) were formulated as early as 1920. The function of a radar altimeter was published in 1930. However, it was not until 1938 that the Western Electric Company presented a practically functioning FMCW radar altimeter.
Civilian use of radar technology was severely hindered by the military secrecy of radar technology. As a result of the events of the Second World War, direct bans on research into radar technologies were issued, which were lifted in 1960. It was not until much later that the advantages of a CW or FMCW radar (simple structure for relatively large ranges with the lowest transmission power) over the pulse radar method were rediscovered. The first FMCW radar for atmospheric investigations was built in 1969. FMCW radars have been used as weather radar and for simultaneous distance and speed measurements since the 1970s. In 1976, FMCW radar was first used to measure the Doppler frequency of wind speeds.
In the 1990s, radar systems on FMCW base for motor vehicles as a collision warning and automatic distance control were ( adaptive cruise control , English Adaptive Cruise Control , ACC) developed. 1600 FMCW radars in the 24 GHz frequency band were installed in Greyhound Lines buses , which reduced the accident rate with these vehicles in 1993 by 21%. A far-reaching 77 GHz FMCW radar has been used for automotive safety in Daimler-Benz vehicles since 1996. At the beginning of the millennium, other vehicle manufacturers followed suit.
With the increasing availability of ever higher frequency ranges and simultaneous miniaturization, modern hybrid technology enables smaller CW and FMCW radar modules to be offered inexpensively in large numbers.
technical realization
A continuous wave radar sends a high-frequency electromagnetic wave in a direction that is predetermined by the directivity of the transmitting antenna . If the electromagnetic wave hits an obstacle, a small part of the transmission power is reflected back to the transmitter as an echo signal and registered there by the receiver. This echo signal serves as proof that there is an obstacle in the direction of propagation of the wave. The properties of the obstacle can be inferred from the properties of the echo signal (for example: strength , phase position , frequency spectrum ).
Channel
The radar modules are often able to be configured as both CW radar and FMCW radar. The transceiver of an FM-CW radar includes a high frequency generator as a voltage controlled oscillator ( English Voltage Controlled Oscillator , VCO) constructed and whose output frequency is dependent on a control voltage. If this is a direct voltage or if this control option is missing, the module works as a CW radar. A signal form to be modulated for an FMCW radar is often generated by means of direct digital synthesis or direct digital synthesis (DDS) and fed to the high-frequency generator in the transceiver as a control voltage. The instantaneous frequency of the VCO can, for example, be changed in a sawtooth shape with the period Τ : the signal S s (t) is transmitted.
For very high frequencies, the VCO often works at a lower frequency. The conversion into the desired radar frequency band is done by a downstream frequency multiplier. In the simplest case, this can be a doubling of the frequency and can be up to a factor of 25. Such a frequency multiplier is a resonant component (for example a cavity resonator ) which can be undamped by an IMPATT diode . For example, the VCO can generate a frequency between 7 and 8 GHz which, after a possible modulation, is converted into the band around 94 GHz by thirteen-fold. It should be noted that the modulation is also affected by the frequency multiplication: a transmitter bandwidth of typically 60 MHz would result in a bandwidth of 780 MHz after the multiplication. This means that the possible frequency tolerance of the resonator assembly has often already been exceeded. Therefore, the modulated bandwidth of the VCO must be reduced when the frequency is to be multiplied.
The maximum possible transmission power is limited by the unavoidable cross-coupling between transmitter and receiver. Since the transmitter works continuously, part of the transmission power reaches the receiver input at the same time as the delayed echo signal and is superimposed on it. If this overcoupled portion of the transmission power is so large that the receiver is driven to the limit , the very weak echo signals can no longer be separated from this composite signal and received. For this reason, separate transmitting and receiving antennas are usually used. These can be decoupled by an additional shield and by the greatest possible distance from one another.
Due to the extremely low transmission power of CW and FMCW radar modules, which are mostly in the range of ≤ 100 mW, no special radiation protection measures are necessary. Even with a strong directivity of the antenna, a very much lower radiation density can be expected than that which occurs in normal operation with a mobile phone.

- annotation
- ↑ Frequency tolerance is a quantity that is often equated with the −10 dB bandwidth . The −10 dB bandwidth of a resonant component is the bandwidth within which the reflection factor due to mismatching on the feed line is a maximum of −10 dB.
antenna
The antenna is often an integrated patch antenna : transmitting and receiving antennas are built on a substrate and often contain 4, 16 or more patches each. The more patches used next to each other, the narrower the resulting antenna pattern of the group. Diagram widths of around 130 ° are typical for a single patch. This can be reduced to about 12 ° by arranging 8 patches next to each other. These patch antennas are a very inexpensive solution. The patches that are furthest away from the feeding point receive the lowest power through a transmission power distributed serially to the left and right. This power distribution is influenced by varying the width of the feed line ( strip line ). It is often a function according to a Taylor series , which results in a narrow main lobe with only a small size of the side lobes. Due to the reduced power at the edge of the antenna field, further added radiation elements have a steadily reduced effect until the engineering effort for enlarging the antenna field and improving the antenna diagram is no longer justified. This limits the number of patches used in the antenna. For very demanding applications, a more effective solution is to arrange the transmitting and receiving antennas in front of a large parabolic reflector, which form an antenna pattern with an opening angle of less than one degree. The antenna diagram is often included in the data sheet of the module as a real measured diagram.
Simpler modules, each with a small quarter-wave dipole as a transmitting and receiving antenna, are also commercially available, which, as motion detectors, enable radar surveillance over a wider area. Depending on the arrangement of this module, 180 ° to 360 ° radar coverage is possible.
receiver
There is at least one down mixer in the module that converts the received signal to a lower frequency. The reflected signal S e ( t ), which is delayed by the transit time, is received . The detector of the module (here the mixer for downconversion to baseband) can be used for a separation of the output signal in an in-phase (I) signal and a quadrature component (Q signal) as a so-called english Synchronous Detector for the in-phase and quadrature components to be designed. For this purpose the final downmix to baseband is done twice. So two mixer stages are fed in parallel with the echo signal divided in phase. The LO frequency (or, if applicable, the transmission frequency) used for the downward mixing is once in phase as a sine (for the I signal, from English in phase ), at the other mixer stage by 90 ° as a cosine (for the Q signal, from English quadrature ) provided. In order to use the information from these two outputs sensibly, the interface must evaluate the I&Q data at the same time . A time-division multiplex transmission to the computer without intermediate storage is not sufficient, as even the slightest time differences during the measurement lead to additional phase changes.
When considering instantaneous values of the frequencies present at the outputs, I is the real part and Q is the imaginary part of a complex signal. The calculation of an amplitude according to the Pythagorean theorem and the phase position according to an angle function is not necessary with continuous wave radar. Since with continuous wave radar no instantaneous values of a sinusoidal oscillation are analyzed, but rather all measured values are available over the entire period of the measurement, when measuring the amplitude of an alternating voltage you can wait until it has reached its maximum value and then this maximum value or the rms value can be measured . The signal at the Q output is only checked to determine whether its phase position leads or lags the I output.
Direct mix receivers and the more sensitive heterodyne receivers evaluate the frequency shift that occurs in moving objects due to the Doppler effect .
Direct mix receiver

A direct mix receiver works as a homodyne receiver . After amplification, the high-frequency received signal is directly superimposed with part of the transmitted signal in a mixer and thus transformed into the baseband . The following amplifier works in the low frequency range . A Doppler radar sensor is usually designed as a small hybrid module . The block diagram shows the basic structure; the analog / digital converter can be part of the module or part of a digital signal processor .
The advantage of the simple structure as a direct mix receiver has some disadvantages:
- For very slow radial velocities, the Doppler frequency is often extremely low and is masked by the 1 / f noise of the down mixer. Additional statistical fluctuations in the phase position of the transmitted signal, the so-called phase noise , make it difficult to measure very small Doppler frequencies.
- This 1 / f noise has a particularly strong effect in the direct mixer, since the components of the mixer were selected for very high frequencies and therefore often have more noise in the low-frequency range. With a heterodyne receiver, this noise can not pass the bandpass filters of the IF amplifier. Because of the strong signal amplification (V> 1000) that took place here, the noise of the second mixer stage is insignificant.
- The maximum installable transmission power is limited. Since the transmitter and receiver work at the same time, part of the transmission energy is always coupled directly into the reception path. If the transmission power is too high, the receiver goes into the limitation , which means that weak echo signals can no longer be recognized. This can only be remedied if the frequency response of the receiver is set up so that a Doppler frequency of zero is not processed. This means that very slow movements can often no longer be detected.
- A poor ratio of the limited maximum possible transmission power to the minimum possible reception power, which is also limited in the case of direct-mix receivers, essentially determines the maximum achievable range.
The ratio of transmission power to reception power and the effect on the radar range is generally described for all radar devices by the basic radar equation . In most applications, the largest damping factor in this equation is the two-way free space damping . It is expressed as r 4 - dependence and has a dramatic effect on the theoretically possible range of the radar (compare: r 2 - dependence of the distance law , which occurs here twice: on the way there and on the way back). In order to double the range of a radar, according to the basic radar equation, the transmission power has to be increased sixteen times.
Overlay Receiver

The sensitivity is improved if the direct mixer is replaced by a heterodyne receiver. In the first mixer of the receiver, the reception frequency band is reduced to a lower frequency band of the intermediate frequency f IF (often between 10 MHz and 100 MHz). The local oscillator frequency required for down-mixing is mixed up from the transmission frequency and a second highly stable generator at the center frequency of the intermediate frequency amplifier and is around the intermediate frequency above the transmission frequency. By converting to a lower frequency band, higher-order band filters can be implemented more easily and thus help to reduce the influence of 1 / f noise. The amplifier stages can be built on this frequency with a higher gain without the risk of self-excitation (natural oscillation due to feedback).
A second down mixer converts the echo signal to baseband. Another possibility is to digitize the received signals directly on the intermediate frequency in a fast analog-digital converter and to carry out the downward conversion digitally.
Possible phase noise as well as smaller frequency jumps of the oscillators lead to short-term measurement errors up to an interruption of the received echo signal, which lies outside the receiver bandwidth during the runtime of this frequency jump. These measurement errors are averaged over time in a CW radar, regardless of the type of receiver. Here, too, the steeper filter flanks of the heterodyne receiver offer better conditions.
Even if only one antenna and a circulator are used to separate the transmission and reception path, part of the transmission energy reaches the receiver directly, as the circulators cannot ideally isolate the outputs from one another. Isolation values of typically 18 dB to a maximum of 30 dB can be achieved. That means: at least one thousandth of the transmission energy is present at the receiver input. From a distance of about 5 m, the echo signal is usually smaller than this unwanted (“parasitic”) signal. In a high-quality continuous wave radar with an invariable transmission frequency and separate transmitting and receiving antennas, the unavoidable crosstalk can be partially compensated internally with a circuit measure : Since its amplitude is constant within certain limits and always takes place with the same phase position , a part can be built within the transmitting antenna the transmission frequency are decoupled and re-coupled into the high-frequency reception path in antiphase. If the phase position and amplitude match, the external crosstalk detected by the receiving antenna is canceled out by destructive interference .
The requirement for a high stability of the generator for the intermediate frequency f IF seems to contradict the block diagram: this frequency is added once in the signal curve ... and then subtracted again. The absolute size of this frequency is theoretically irrelevant, in practice it only has to be in the structurally specified pass band of the IF amplifier. However, it must not change during the measurement. A drift in the intermediate frequency during the transit time of the echo signal within the receiver inevitably leads to a measurement error. In practice, frequency instabilities that would result from mechanical vibration of the generator are particularly relevant here.
Unmodulated continuous wave radar (CW radar)
functionality
An unmodulated continuous wave radar sends electromagnetic waves with a constant amplitude and a constant frequency. The echo signal from moving reflecting objects differs from this transmitted frequency by a superimposed Doppler frequency. The frequency difference between the transmission signal and the echo signal results in the Doppler frequency at the output of the mixer stage. Echo signals from fixed targets would result in a DC voltage at the receiver output and are often by a Transformer balanced hidden mixing stage. Here, too, there is a transit time between the transmission signal and the echo signal. Due to the periodicity of the sinusoidal oscillation, it can only be measured as a phase difference. How many full periods of the sinusoidal oscillation have passed in addition to this measurable phase difference cannot be determined. An absolute distance measurement is therefore not possible with a single CW radar (see below).
Subject of measurement
Speed measurement
The Doppler frequency f D is used as the measured variable for a speed measurement . In the case of radar devices, it should be noted that the Doppler effect always occurs twice: Once on the way from the transmitter to the reflecting object (signal source at rest, observer moving). There the wave that has already been Doppler-shifted is reflected and the Doppler effect occurs a second time on the way back to the receiver (now: observer at rest, signal source moved). The Doppler frequency for monostatic radar devices (i.e. those in which the transmitter and receiver work at one location) is therefore calculated according to the equation:
where v r is the radial velocity of the reflecting object and λ is the wavelength of the transmission energy. An object with the speed v = 5 m / s generates the Doppler frequency f D = 800 Hz at an oscillator frequency of 24 GHz , which is in the audible range and can be easily evaluated from a technical point of view. In most cases, the "approximately" sign in the formula can be replaced with an equal sign. It has its cause in the condition for the validity of the equation. On the one hand, this is the requirement that the radial speed must be much smaller than the speed of light (which is true in all known cases) and, on the other hand, that the propagation speed of the electromagnetic waves does not change during the measurement. In some applications, this can happen sporadically, for example when crossing layers of air with different densities in the atmosphere or when using it as a level meter, since the speed of propagation in air is different from that in liquids or solids (bulk material). With level gauges, for example, this has the effect that the bottom of the container appears to be further away when the container is full than when the container is empty.
If the speed is not measured directly in the direction of movement of the object, the measured radial speed differs from the real speed of the object. For example, the mobile measuring system shown in the picture is used for speed monitoring in road traffic and works in the K band on the frequency 24.125 GHz. The system can be installed in a measuring vehicle or operated on a special tripod to monitor the flow of traffic with photographic documentation of traffic violations. With this device, speeds of 20 km / h to 250 km / h can be measured. The speed of the incoming and / or outgoing traffic can be measured from the right or left edge of the road. Each device has to be calibrated individually and is set for operation at an angle θ of 20 ° to 22 ° inclined to the road. A sufficiently accurate calculation of the driving speed from the measured radial speed is only possible with this fixed radiation angle:
- here:
A slight inaccuracy in the alignment of the continuous wave radar to the roadway therefore has only a minor influence on the speed calculation in this angular range and is smaller than the specified measurement tolerance.
Detection of several objects at different speeds is possible with a continuous wave radar, but this increases the effort. Since all Doppler frequencies are superimposed in the receiver , they must be separated from one another by filters that work in parallel. In practice, this means that a separate filter with a subsequent threshold value circuit and detection logic must be set up for each expected Doppler frequency. Since the possible number of these filter channels is limited, both the measurement accuracy and the resolution of the continuous wave radar are limited. An assignment of the measured Doppler frequency to the respective target is not possible with the unmodulated CW radar. In practice, such a distinction is usually not made; instead, only the most intensive Doppler frequency at the time of measurement is evaluated. For the speed measurements by the police or the public order office, this means that if several vehicles can be seen in the image, it can usually not be proven to which vehicle the measured speed belongs.
In the case of simple downward mixing with the aim of measuring the amount of a Doppler frequency, the information about the direction in which the reflecting object has moved is lost. Some Doppler radar sensors can also provide phase information using the I&Q method . The evaluation circuit is thus able to recognize a direction of movement in addition to the speed. This is not an angle measurement: this just means that the radar can detect whether the object has moved towards or away from the antenna at the measured radial speed.
Distance checking
Under certain conditions, a continuous wave radar can measure a change in distance on the order of half the wavelength of the transmitter, i.e. a few millimeters. For this purpose, no Doppler frequency is evaluated, but a phase position between the transmitted and received wave is checked and a change in this phase position is evaluated either with a storage oscilloscope or spectrum analyzer or a computer with appropriate software to register the change.
This operation is possible if a high quality mixer stage, for example an HF ring modulator or a Gilbert cell , is used. If this is fed with two identical but phase-different very high frequencies at the inputs (i.e. the frequencies are only slightly shifted in time), a DC voltage appears at its output, which represents the size of the phase difference between the two input frequencies. To evaluate this voltage to the internal mixer terminals and the further signal processing must for direct current be coupled. Due to the periodicity of the sinusoidal oscillation of the carrier frequency in a single CW radar, this measurable phase difference cannot provide a clear determination of the distance. How many complete periods of the sinusoidal oscillation are added to this measured phase difference cannot be determined.
Since the diodes used in the ring modulator have a forward voltage typical of the design , the relationship between phase difference and DC voltage is not linear and very small phase differences do not result in any clear changes in the output voltage. Because of this (and because of the lack of clarity), this operating mode cannot be referred to as a measurement, but only as a check.
The echo signal must for evaluating a very strong amplitude have, since in spite of the directivity of the antenna part of the strong transmission power (relative to the reception power) by superposition superimposed on the echo signal at the receiving antenna. This energy, which acts directly on the receiving path from the transmitting antenna (so-called crosstalk ), may be stronger than an echo signal from a distance of about 5 m. The necessary direct current coupling significantly reduces the possible receiver sensitivity and thus the achievable range. Either a very short measuring distance or a quasi-bistatic operation (i.e. a certain distance between transmitting antenna and receiving antenna) with shielding between the two antennas is therefore assumed. The echo signal can be amplified by using corner reflectors .
Distance measurement
Distance measurement is also possible with unmodulated continuous wave radar with certain restrictions. For this purpose, two identical unmodulated continuous wave radar modules, with slightly different transmission frequencies f 1 and f 2 , must be operated in parallel independently of one another, and their output signals are subject to a common signal processing. In the propagation of electromagnetic waves created a beat both vibrations. The target distance can be determined by means of the phase difference Δ Φ between the two echo signals, the possibilities of speed measurement being retained in both channels. So both output signals of the continuous wave radar modules are given to the inputs of a phase measuring circuit. The phase difference is proportional to the frequency difference of the transmitters and the distance R ( t ) to the target:
The frequency difference (f 1 - f 2 ) must be chosen so that the phase difference for the energetically maximum possible distance does not exceed the value 2π. It should therefore be less than or equal to the reciprocal of the maximum expected running time :
It should also be larger than the respective receiver bandwidths so that mutual interference is excluded. If two pairs of harmonic oscillations with two different phase differences are present on the phase meter, which corresponds to a measurement of two targets at different distances, only the phase difference of the sum of these oscillations can be displayed. That is, this method can only measure the distance to a target; there can be no resolving power after removal.
If inexpensive continuous wave radar modules are used as transceivers in the K-band, they must be selected so that they do not interfere with one another, i.e. do not output any output signals directly to one another during test operation. Then the difference frequency (caused by the spread of the tolerances of various frequency-determining components) is greater than the receiver bandwidth. With a frequency difference of 100 kHz, for example, the maximum unambiguous measurement distance is 3 km. If a third transmission frequency is used, the maximum unambiguous measuring distance can be extended accordingly, since a given combination of a phase difference between three different frequencies can only be repeated much later.
This procedure is called Multi-Frequency Ranging (MFR) (in German: distance measurement with several frequencies). In practice, however, no two (or more) unmodulated CW radars are used for this, but a frequency-modulated CW radar which works on the various frequencies in time division multiplex and is thus assigned to the FMCW radar units.
Applications of unmodulated continuous wave radar
The use of continuous wave radar devices for speed measurement is diverse and is always carried out where contactless measurement is necessary.
- It is widely used in sport: for measuring ball speeds, for example when shooting a goal in football or handball; or the ball speeds in tennis, table tennis or golf; as well as in winter sports for measuring the speed on bobsleigh or toboggan runs, as a jump speed for ski jumping or for measuring speeds on special sections of downhill runs.
- CW radars are used to measure the speed in road traffic for mobile use. The speeds permitted by the radar devices due to the necessary calibration for measurement are often limited to 20 km / h. At lower speeds, the phase noise of the high-frequency generator would have too great an influence on the measured (then very small) Doppler frequency, so that these measurements would subsequently be legally unusable.
- With a motion detector , a speed determination is dispensed with. Instead, it is only checked whether the Doppler frequency exceeds a specified value, because then an object moves with the selected minimum radial speed in the radiation area. The switching signal is evaluated by a higher-level controller, for example an alarm system or a controller for a video or infrared camera (in a so-called “slew-to-cue” configuration). ( There is no meaningful German translation for this “slew-to-cue” . It means something like: “Camera panning caused by a hint”.)
- In addition to infrared sensors, these motion detectors are also used to control door openers. You can set off a false alarm when driving by vehicles that are equipped with a radar warning system (which is illegal in some countries) .
- The principle found a military application in the anti-aircraft missile system HAWK and in the Sea Sparrow in the form of bistatic radar. The target was illuminated with a strong continuous wave radar (High-Power Illuminator Radar, HPIR). There were two directional antennas in the semi-active missile: one in the bow aimed at the target and one in the stern aimed at the transmitter. From the comparison of the signal strengths of the stern and bow antennas, it was possible to infer an imminent approach to the target.
- The principle of the distance check can be used as a special case of application for a contactless measurement of breathing and cardiac activity of an intensive care patient. However, this method has not yet been clinically established.
Modulated continuous wave radar
Unmodulated continuous wave radar devices (also called Doppler radar ) can only measure changes in the distance to objects (radial velocities), but not absolute distances, because the time reference for measuring the absolute transit time of the transmitted signal is missing. In contrast, if the signal is changed ( modulated ) over time, the transit time of the change in the transmission signal can be measured. In principle, any type of modulation (frequency modulation, phase modulation and amplitude modulation) is suitable for providing the transmitted wave with marks that allow conclusions to be drawn about the transit time after reception. One type of amplitude modulation leads to pulse radar . Because different object sizes and reflectivity strongly influence the amplitude curve of the echo, frequency modulation is almost exclusively used in continuous wave radar devices and the devices using this method are referred to as frequency-modulated continuous wave radar or FMCW radar. In their function, these can also be used as imaging radar.
Frequency modulated continuous wave radar (FMCW radar)


functionality
FMCW radars often work with periodic frequency modulation, which has to be as linear in time as possible in order to meet high demands on the accuracy of the measurement. The often existing non-linearity of the change in the output frequency as a function of the control voltage is compensated by a predistortion of the digitally generated tuning voltage, which deviates from the linear course in the opposite direction as the high-frequency generator. Historical FMCW radars also used sinusoidal modulation.
The modulation is usually carried out cyclically. Such a passage from the lowest to the highest frequency is called a signal sequence (usually English burst ). The frequency swing of such a burst can be a few gigahertz. The steepness of the frequency change has an influence on the resolving power of the FMCW radar. Since the bandwidth of the receiver then limits the maximum possible frequency change at the same time, a compromise has to be found between resolution and range.
Subject of measurement
Distance measurement only
During the time Δ t = 2 r / c that the emitted signal needs to travel the distance r to the reflecting object twice (there and back) at the speed of light c , the frequency of the transmitter has already changed. The faster the frequency is changed, i.e. the greater the steepness of the frequency change d f / d t , the greater the effect. If no Doppler effect has to be taken into account, Δ f = Δ t d f / d t and the distance can be calculated as follows:
When the reflecting object moves relative to the radar, the echo shifts up or down by the Doppler frequency f D. In the case of a sawtooth-shaped modulation, this frequency change cannot be clearly assigned to a distance or a speed and therefore generates a measurement error . This becomes smaller, the greater the slope of the frequency change is chosen. If, for example, an FMCW radar in the K-band uses a frequency change of 100 MHz per microsecond and uses a total bandwidth of 250 MHz, the radar only works in the range of a few 100 meters with a clear measuring distance. For this, a possible Doppler frequency in the kilohertz range, which corresponds to a speed of around 10 m / s, can be neglected.
The steeper the frequency change, the greater the frequency difference for a given time difference and the range resolution of the FMCW radar improves. At the same time, the limitation due to the installed maximum transmitter bandwidth takes effect much earlier and the maximum clearly determinable measuring distance is reduced. The reverse applies: if the frequency change is flatter (read: slower), this clearly determinable measuring distance increases. The choice of this parameter is therefore a compromise between range, accuracy (because of the measurement errors due to the Doppler frequency) and the distance resolution.
Simultaneous measurement of distances and speeds

Triangular modulation
The use of a triangular form of modulation offers the possibility of determining the Doppler frequency as a measure for a radial speed as an independent measured variable in addition to the distance measurement. During the approach, the receiving frequency increases and thus reduces the amount of the difference frequency during the rising edge. Due to the superposition of the Doppler frequency, the frequency difference when measuring an approach is smaller in the rising edge of the triangular modulation, in the falling edge it is higher by the same amount than with a static reflector. With the same steepness of the frequency change in the rising and falling edges of the triangular signal, the mean value of the difference frequencies from the two measurement periods is a measure of distance, regardless of the speed. The sum of the difference frequencies is a measure of half the radial speed of the object. Particularly high demands are now placed on the linearity of the frequency change.
In order to convert the primary measurement results into an image, the measured frequencies must be recognized by means of a filter and then the frequencies of the rising edge must be correlated with the frequencies of the falling edge of the triangular modulation. Only then can an average value for the distance and half the difference for the radial speed be calculated.
In a scenario with multiple reflective targets, there may be ambiguities when using triangular modulation. The measured Doppler frequencies and the frequency changes due to the transit time can no longer be clearly assigned to a specific target due to the signal processing. So-called ghost targets can arise by assigning the measured Doppler frequency of one target to the distance-determining travel time-related frequency deviation of a second target. These ambiguities can be excluded by using alternating measuring cycles with different frequency deviations and only those targets are displayed which occur in the same position in both measuring cycles.
Due to the relatively high computational effort required to calculate the speed and to correct the distance, this form of modulation is rarely used and is being replaced more and more by the following frequency shift keying method (a form of modulation with frequency shift keying ), which offers considerably simpler signal processing .
Frequency shift keying
With the modulation known as Frequency Shift Keying (FSK), the frequency-modulated continuous wave radar can adopt properties that are similar to pulse radar. No sawtooth or triangular modulation voltage is used, but a rectangular one. This is why the FMCW radar works like a CW radar, but jumps back and forth periodically between two transmission frequencies. These are relatively close together so that the echo signals of both frequencies fit into the bandwidth of the receiver. The transit time is measured from the frequency jump of the transmitter until this frequency jump is registered in the echo signal. As with impulse radar, the distance to the reflecting object is determined from this.
The Doppler frequency is then determined and the speed is calculated from this. Technically, after the frequency jump in the transmitter, a relatively high differential frequency will first occur after the mixer stage in the receiver. Their size is the installed difference frequency of the two transmission frequencies plus / minus a possible Doppler frequency. The radial speed of an object can easily be derived from this signal. The detection of several Doppler frequencies is theoretically possible with one filter bank. With this modulation method, however, it is not possible to then assign this Doppler frequency to a specific target. When this transmitted frequency jump reaches the receiver after the transit time (to the object and back), this state with the relatively high difference frequency is ended. The envelope of this difference frequency is a square pulse, the pulse length of which is a measure of the distance between the radar and the reflecting object. If several of these distances are superimposed, the falling edge of this envelope curve has the shape of a staircase voltage. Each falling partial edge of this staircase voltage stands for a single echo signal and is easy to detect with a high pass : the later this edge occurs, the further away the reflecting object is. A possible Doppler frequency has no influence on this edge; this source for a systematic measurement error is therefore omitted with the FSK-FMCW radar.
Some practically tested evaluation circuits can achieve extreme accuracy in determining the distance down to the wavelength of the transmission frequency by measuring the phase difference between the two transmission frequencies used. The measuring principle corresponds roughly to the measurement of a distance with two unmodulated continuous wave radar devices . A combination of this evaluation with the run-time measurement of the frequency jump described here can overcome the ambiguities in the distance measurement in the case of pure phase measurement, which can occur due to phase differences greater than π . The disadvantage remains that only a single target can be measured with this method, since only a single phase can be measured at the output of the mixer stage. The advantage, on the other hand, is that if two phase positions overlap and cannot be evaluated, then at least the less precise measurement distance from the time measurement is retained.
Stepped-Frequency Continuous Wave (SFCW)
The function of the SFCW radar is an extension of the function of an FSK-FMCW radar. Stepped frequency here means something like "stepwise change in frequency". The frequency change can follow a pattern similar to a staircase voltage, or for example according to the Costas code . A clear distance measurement is possible through the transit time measurement as with the FSK method. This can then be made more precise by measuring the phase position (similar to the distance measurement using two unmodulated CW radar devices ). Since many different frequencies can be compared with one another within one run of the modulation pattern (the so-called scan ), very precise measurements are possible.
However, the problem mentioned under the point of distance measurement with CW radar also applies here , that because of the impossibility of separating two different overlapping phase shifts, only a single target can be measured. In practice, this is always the destination with the greatest reception power. In most cases, according to the r 4 dependence of the amplitude of the echo signal, this is also the target with the shortest distance to the radar.
Electronic panning of the antenna pattern
In the case of a sawtooth or triangular modulation, a simultaneous electronic swiveling of the antenna diagram is possible for area scanning by an FMCW radar. Since the same distance is measured at every point in time on the edge of the sawtooth using the same frequency difference, the distance measurement is redundant over the entire frequency range. On the one hand, this can be used to carry out signal integration with a lower transmission power in order to improve the interference immunity and increase the range. However, if the radar has relatively large power reserves, such signal integration is not necessary for target recognition. Some FMCW radar sets take advantage of this to use this change in transmission frequency at the same time for electronic beam swiveling to scan the room.
Technically, this is implemented like a phased array antenna with frequency-dependent beam swiveling . Several adjacent antennas are fed in series , for example from a waveguide with many slot antennas . Detour lines of fixed length are located between the individual radiators. For the mean transmission frequency, this detour corresponds exactly to the wavelength. At this transmission frequency, the resulting antenna diagram points in the direction of the central axis of the antenna group. If the frequency increases, the wavelength decreases and the mechanically fixed detour between the individual radiators is now a little too long. A phase shift occurs from emitter to emitter, which in total causes an electronic beam swivel. The last antenna in the serial feed receives the oscillation by the sum of these phase shifts later than the first. The resulting wave front of this antenna group is thus swiveled from the central axis in the direction of the last individual antenna. Thus, a sector of up to 90 ° is scanned with a stationary antenna group and replaces a mechanical antenna pivoting or rotation.
Applications of modulated continuous wave radar

- below: assembled assembly
- left: pot circle TK with disc
triode ST - right: cover with sector element S driven by motor M for a capacitively controlled frequency modulation
- This type of distance determination is used in industrial metrology, for example, to measure the level of bulk goods or liquids in containers and silos. If different frequencies are used, the thickness of foam layers on liquids can also be measured. If the filling material is semitransparent for electromagnetic waves, the bottom surface of the container appears as an additional measured value. When calculating the distance, the different propagation speeds of the electromagnetic waves in the air (which may be under pressure), in any foam that may be present and in the filling medium must be taken into account. The speed of propagation in the filling medium is always lower than in air, which is why the bottom of the container appears to be further away when the level is high than when the container is empty. In addition to the useful signal reflected on the surface of the liquid or on the foam, interfering signals arise, for example due to reflections on attachments within the tank or due to an agitator.
- A microprocessor is often used to evaluate the measurement signal, which can calculate these interfering signals because their positions are known after a long period of operation. Another computer-aided process is called multi-echo tracking . These radar devices are usually only approved for operation inside closed, shielded containers, as they use a transmission frequency that the Federal Network Agency does not allow to be emitted into free space .
- FMCW radars in the three-digit gigahertz range, including antennas integrated on a chip, can a. can be used as a direct speedometer for vehicles or for precise distance measurement (<1 mm at a distance of several meters). These are also used for non-contact material testing and enable, for example, the inspection of already packaged components. In the case of materials that are transparent to the high frequency (e.g. molded ceramic parts, textiles), a look through the material can reveal faults in the material. In the case of reflective materials, these devices enable the surface and the position of additional layers to be inspected.
- Borehole probes, among other things, are used to investigate underground geological structures, in which the electrical properties in the vicinity of the borehole are determined by radar measurements in the frequency range from approx. 10 MHz to 100 MHz. Also, from the surface, this method can be used as so-called ground-penetrating radar ( English Ground Penetrating Radar be used GPR). In both applications, the FMCW radar method achieves a very high range resolution despite the low circuit complexity.
- With a FMCW radar comparatively very low power low carrier frequencies can be attributed to (compared to typical radar frequencies) in the short-wave range between 5 and 50 MHz with the bump one over-the-horizon radar ( Over The Horizon - Surface Wave OTH-SW) realize that for observing Coastal sections and is used as a weather radar.
- In aircraft, FMCW radars serve as radar altimeters (radio altimeters) during a landing approach, whereby the limitation to a range of a few 100 meters does not interfere. However, it cannot be used as a ground follower radar, as a radar with a view ahead is required due to the high airspeed.
- In the adjacent picture of the historical altimeter a sinusoidal modulation was used. This was caused by the rotation of the capacitive tuning element in the resonance chamber. However, only a small part of this modulated sinusoid was used (around ± 30 ... 45 ° around the 0 ° and the 180 °). This part of the sine curve is relatively linear, even if no great demands could be made on the accuracy. With this modulation method, easy-to-use and robust devices could be built.
- Far-reaching FMCW radar devices on 77 GHz are used as sensors for brake assist and adaptive cruise control in luxury vehicles. These devices can provide digital chart shaping in the receiving antenna for a quick change in the width and main direction of the antenna pattern. For this purpose, the HF signal is distributed to different antenna groups with different radiation angles by means of fast PIN diode switches .
- A navigation radar for ships and boats is commercially available under the name Broadband Radar ™ . In terms of the measurement method, this is an imaging modulated continuous wave radar, although it inserts a pause of 5 ms after every 1 ms long period of frequency change. The resolving power is much better than that of pulse radars equipped with magnetrons . In addition, the transmission power (compared to the 4 kilowatts of pulse power otherwise required for this range) can be reduced to just 100 milliwatts. Because this radar works with a frequency deviation of 65 MHz per millisecond, the measurement error due to Doppler frequencies is relatively large, which is of little importance in maritime use with the usual low speeds, but excludes the use of this radar on an airfield. At take-off and landing speeds in the range of 200 to 300 km / h, the measurement error would amount to several kilometers: the aircraft is not seen where it is, but its echo signal may be outside the screen scale.
- In security technology, FMCW radars are used to protect sensitive military or civilian objects from conventional, asymmetric or terrorist threats.
- FMCW radar devices are used in meteorology to observe boundary layers in the atmosphere , which document the flight of insects and the height of boundary layers between air of different densities. At the transmission frequency of 2.9 GHz, the radar can distinguish between the echo signals of the insects (caused by Rayleigh scattering ) and the boundaries between different layers of air with different refractive indices (recognizable by turbulence and eddies at the boundary layer according to the Bragg condition ). The heights of the boundary layers can be determined up to a height of 5 km to within 5 m. The insects serve as indicators for air movements (wind) at higher altitudes.
- Even powerful military pulse radars such as the SMART-L by Thales are "silent mode" capable of in a so-called ( English silent mode ) switch and a low probability of intercept radar called (difficult aufzuklärende radars). In this mode they can be configured with an extremely low energy emission and are very difficult to detect by a possible opponent. You then use the intrapulse modulation in order to be able to determine the distance within the transmission pulse. If the duration of the transmission pulse is longer than the reception time, then the conditions are met for this radar to also be designated as FMCW radar. At the same time, in this case, instead of the usual pulse compression method as a function of time (time domain) in pulse radar, the much simpler and more precise calculations as a function of frequency (frequency domain) can be used to determine the distance.
- Because of the extraordinary accuracy in determining the range, FMCW radar devices (especially stepped frequency FMCW) are used to track artillery shells in real time .
- FMCW radar for cable testing see also time domain reflectometry .
Phase-coded continuous wave radar

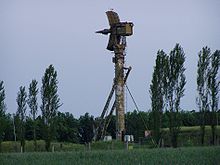
The oscillator of a phase-coded continuous wave radar generates a fixed frequency, the course of which is divided into short sections of equal length. The phase position is constant within each section, the length of the sections is determined by the required distance resolution. A phase jump is inserted at irregular intervals between the sections, which often only last a few nanoseconds. The phase is switched by fast PIN diode switches , which switch a short detour line into the transmission path, so that the phase at the antenna is rotated by 180 °. This PIN diode switch is controlled with a digital voltage that is linked to a binary code , which is why the sequence of these phase changes corresponds to a bit pattern. Another possibility for phase modulation is to use an I&Q vector modulator . This divides the RF signal in a −3dB directional coupler into two parts that are 90 ° out of phase with one another. One amplifier stage each perform a further phase rotation of 180 ° for the partial signals. Afterwards, voltage-controlled attenuation with different levels takes place in each partial path, before both partial signals are combined again with a −3dB directional coupler. As a result, the output signal has a phase shift that is dependent on the magnitude of the control voltage.
The phase position of the transmission signal is now dependent on a sequence of pulses, the bit pattern of which can correspond to a Barker code, for example . This Barker code can also be nested multiple times. This pattern must not be repeated within a long time course, which is specified by the maximum expected transit time of the electromagnetic wave. It would be ideal to generate this bit pattern using a random generator, but pseudo-random bit sequences are also practically possible and allow a simple evaluation circuit, since the original transmission signal no longer has to be delayed step by step, but is created again with a delayed start time where it is needed can be.
If the downward mixing takes place in the receiver with the unmodulated frequency, the bit pattern appears at the mixer output as a pulse train. In the past, the transmitted bit pattern was gradually delayed with a long delay chain for comparison with the received signal. In the meantime, the computer-aided sliding window method is used to compare the received bit pattern with the transmitted signal . The number of bits shifted until the transmitted and received signals match is the measure of the distance, which enables the distance and radial velocity to be measured simultaneously, since the Doppler frequency that may be measured has no influence on the determination of the distance.
This procedure has the following advantages over pulse radar:
- Although high pulse power is not used, equivalent results can be achieved with much less continuous wave power;
- The low power makes it difficult to detect this radar;
- The system can hardly be disturbed by external signals, since the bit pattern used must be known for an imitation.
Noise-modulated continuous wave radar

- top: transmission signal of a noise-modulated radar (there is no pause in transmission between the pulses)
- middle: received delayed noise (the red lines in the picture are only visual aid, they have no function in the radar)
- below: correlated delayed output pulse
A noise-modulated amplitude or phase modulation can be used to measure a distance with a continuous wave radar. For broadband radar, high-frequency white noise can be directly power- amplified and transmitted. The pulses modulated by the noise are only a few nanoseconds long with a transmitter bandwidth of several gigahertz. Here, too, the transmitted noise signal has to be delayed step by step in the receiver and is compared with the received signal in a correlator . These radar devices mostly work on frequencies above 80 GHz and enable a range resolution of a few centimeters. It is not possible to clarify or interfere with these radar devices, as additional noise cannot be detected within the natural ambient noise and the exact transmission signal must be known for effective interference or deception.
For reception, the reflected high-frequency noise signals are mixed down by means of a highly stable local oscillator that oscillates at the center frequency of the transmission frequency band. The output signal of the mixer stage is amplified, compared with the delayed transmission signal and compressed in a tuned receiver, the correlator, to a short output pulse, which is similar in function to the pulse compression filter in the pulse compression method . In the received signal, the natural ambient noise also occurs, which is also superimposed on the transmitted signal as inherent noise. This additional noise is statistically correlated out by comparison with the transmission signal . The pulse duration of the output pulse is ideally as long as the individual noise pulses of the transmitter, depending on the transmitter bandwidth usually only a few nanoseconds long. The resolution of this radar is therefore 15 cm for noise pulses of 1 ns length - the transmitter bandwidth must be at least 1 GHz in this case. The accuracy of the measurement of the transit time is independent of the resolution and depends on how many steps delay in the correlator are provided or technically feasible for a single transmitted noise pulse.
The further development of this process, especially by sending out only isolated noise pulses, leads to noise radar (also called UWB radar because of the large bandwidth ). However, since their transmitters do not work continuously during the measurement process, these devices are assigned to pulse radar devices .
Frequency releases
There are two fundamentally different continuous wave radar applications with regard to frequency release. One possibility is to operate the radar inside shielded containers (e.g. for level measurements), which have the properties of a Faraday cage and do not allow any radiation to the outside. Only type approvals in which the operating conditions are specified are required for these devices .
All other radar devices that radiate into free space require a frequency approval from the Federal Network Agency . This specifies in administrative regulations which frequencies or which frequency bands may be used by whom and how.
In the so-called ISM type B bands , any applications are possible without special frequency approval. In such special frequency bands, continuous wave radar applications can also be put into operation without authorization. Many inexpensive transceivers are available for the ISM band from 24 ... 24.25 GHz and can be used to set up motion detectors and distance measuring devices. Radar transceivers that operate in other frequency bands are also often offered. These are mostly designed for other continents (e.g. the USA) and may not be put into operation in Germany or only within the above-mentioned shielded containers.
literature
- Shahan A. Hovanessian: Radar System Design and Analysis. Artech House, Dedham MA 1984, ISBN 0-89006-147-5 .
- William K. Saunders: CW and FM RADAR. In: Merril I. Skolnik (Ed.): Radar Handbook. 2nd Edition. Mcgraw-Hill Professional, New York a. a. 1990, ISBN 0-07-057913-X , Chapter 14; www.helitavia.com (PDF; 1.31 MB).
- Яков Д. Ширман: Теоретические основы радиолокации. Издательство "Советское Радио", Moscow 1970.
- German translation: JD Schirman u. a .: Theoretical basics of radio location. Military publishing house of the GDR, Berlin 1977 (a technically good translation of the original carried out by lecturers and specialist teachers from the former officers' college of the LSK / LV ; disadvantage of this translation: many technical terms are compulsively Germanized and no longer correspond to today's linguistic usage).
- Edgar Voges : high frequency technology. Volume 2: Power tubes, antennas and radio transmission, radio and radar technology. Hüthig, Heidelberg 1987, ISBN 3-7785-1270-6 (Chapter 22.6 “CW and FMCW radar”).
Web links
- Federation of American Scientists "Continuous Wave Radar"
- Radar tutorial : "Continuous wave radar devices (CW radar)"
- Radar tutorial : "Modulated continuous wave radar devices (FM-CW radar)"
Individual evidence
- ↑ David K. Barton, Sergey A. Leonov (Eds.): Radar Technology Encyclopedia. Artech House, Boston MA et al. a. 1997, ISBN 0-89006-893-3 , p. 340.
- ^ Edgar Voges: high frequency technology. Volume 2. 1987, p. 348.
- ↑ Patent DE165546 : Method to report removed metallic objects to an observer. Inventor: Christian Hülsmeyer ( dpma.de (PDF; 293 kB)).
- ↑ Patent DE169154 : Method for determining the distance from metallic objects (ships or the like), the presence of which is determined by the method according to patent 165546. ( radartutorial.eu (PDF; 230 kB)).
- ^ Merrill I. Skolnik: Introduction of Radar Systems. McGraw-Hill Inc., New York NY et al. a. 1962, p. 9, ( preview ( memento of December 3, 2012 in the Internet Archive )).
- ^ Edward V. Appleton , Miles AF Barnett: On Some Direct Evidence for Downward Atmospheric Reflection of Electric Rays. In: Proceedings of the Royal Society of London . Series A: Mathematical, Physical and Engineering Sciences. Vol. 109, No. 752, 1925, pp. 621-641, doi: 10.1098 / rspa.1925.0149 .
- ^ Lloyd Espenschied , Russell C. Newhouse: A Terrain Clearance Indicator. In: The Bell System Technical Journal. Vol. 18, No. 1, 1939, pp. 222-234, doi: 10.1002 / j.1538-7305.1939.tb00813.x .
- ^ Peter C. Sandretto: The Long Quest. In: Transactions of the IRE Professional Group on Aeronautical and Navigational Electronics. Vol. ANE-1, No. 2, June 1954, p. 2, doi: 10.1109 / TPGAE.1954.5062382 .
- ↑ FJ Dallemand, J. Lichtenegger, RK Raney, R. Schumann et al .: Radar Imagery. Theory and Interpretation. Lecture Notes (= RSC Series. No. 67, ZDB -ID 19599-6 ). Food and Agriculture organization of the United Nations - Publications Division, Rome 1993, p. 8.
- ↑ Data sheet of a transceiver IC from the company "Silicon Radar", radartutorial.eu (PDF).
- ^ Igor V. Komarov, Sergey M. Smolskiy, Fundamentals of Short-Range FM Radar. Artech House, Boston et al. a. MA 2003, ISBN 1-58053-110-5 ( limited preview in Google book search).
- ^ Juergen H. Richter: High Resolution Tropospheric Radar Sounding. In: Radio Science. Volume 4, No. 12, 1969, pp. 1261-1268, doi: 10.1029 / RS004i012p01261 .
- ^ Donald E. Barrick: FM / CW radar signals and digital processing (= NOAA Technical Report ERL 283 -WPL 26, ZDB -ID 60512-8 ). Environmental Research Laboratories, Boulder CO 1973, codar.com (PDF; 6.17 MB).
- ^ RB Chadwick, KP Moran, RG Strauch, GE Morrison, WC Campbell: A New Radar for Measuring Winds. In: Bulletin of the American Meteorological Society . Volume 57, No. 9, 1976, pp. 1120-1125, doi : 10.1175 / 1520-0477 (1976) 057 <1120: ANRFMW> 2.0.CO; 2 .
- ^ Richard George Strauch: Theory and Applications of the FM-CW Doppler Radar. 1976, (University of Colorado Ph. D. thesis, 1976).
- ^ Martin Schneider: Automotive Radar - Status and Trends. In: Wolfgang Menzel (Ed.): German Microwave Conference - GeMiC 2005 - Conference proceedings. University of Ulm, April 5–7, 2005. Ulm University, Ulm 2005, ISBN 3-00-015423-X , pp. 144–147, uni-duisburg-essen.de (PDF; 355 kB).
- ↑ Product catalog 2010 from ELVA-1 Millimeter Wave Division (DOK Ltd.), St. Petersburg, 193318 Russia, p. 8, elva1.com (PDF; 3.9 MB).
- ^ Edgar Voges: high frequency technology. Volume 2. 1987, p. 351.
- ↑ Example of a computer interface for CW radar.
- ↑ Shahan A. Hovanessian: Radar System Design and Analysis. 1984, p. 84.
- ↑ typical values of the isolation see data sheet ( Memento of March 4, 2016 in the Internet Archive ) (PDF; 1.5 MB) for circulators in the X-band.
- ↑ Phillip E. Pace: Detecting and classifying low probability of intercept radar. 2nd edition. Artech House, Boston MA et al. a. 2009, ISBN 978-1-59693-234-0 , Section: 4.4.3 Single Antenna Isolation Using a Reflected Power Cancerler. , P. 97, ( limited preview in Google Book search).
- ↑ Derivation of the formula on the radar tutorial .
- ↑ Tobias Wust: Speed cameras and speed measurement. tredition GmbH, Hamburg 2009, ISBN 978-3-86850-021-9 ( limited preview in the Google book search).
- ^ William K. Saunders: CW and FM RADAR. In: Merril I. Skolnik (Ed.): Radar Handbook. 2nd Edition. 1990, pp. 14-18, Chapter 14 (PDF; 1.31 MB).
- ↑ on using a storage oscilloscope see this software solution ( Memento from November 19, 2012 in the Internet Archive ), for spectrum analyzer see device structure in the Institute for High Frequency Technology and Electronics ( Memento from March 18, 2014 in the Internet Archive )
- ↑ JD Schirman et al. a .: Theoretical basics of radio location. Military Publishing House of the GDR, Berlin 1977, p. 399.
- ↑ a b Brochure ( Memento of November 10, 2013 in the Internet Archive ) (PDF) about the CW Radar from BAE Systems (PDF 2.44 MByte)
- ↑ Vmax 100 , Technical data and functions of a Doppler radar. ( Memento from February 25, 2014 in the Internet Archive ) (PDF) EUROtronic Technology GmbH for speed measurements in sports.
- ↑ 60 years of radar trap, article from SWR.de.
- ↑ Jens Muehlsteff, R. Pinter, G. Morren (Philips Res. Lab. Europe): Comparison of Respiration Rate Monitoring with a Low-cost Doppler-Radar Sensor and Inductive Thorax-Plethysmography. In: World Congress on Medical Physics and Biomedical Engineering. IFMBE Proceedings, Vol. 25/7, 2009, ISBN 978-3-642-03884-6 , pp. 768-771 ( limited preview in Google book search).
- ^ David J. Daniels: EM Detection of Concealed Targets. Wiley & Sons, Hoboken, New Jersey 2010, ISBN 978-0-470-12169-6 , pp. 229-231 ( limited preview in Google Book Search).
- ↑ Shahan A. Hovanessian: Radar System Design and Analysis. 1984, pp. 329-331.
- ↑ Development board ST200, user manual. ( Memento from May 1, 2015 in the Internet Archive ) (PDF; 3.5 MB) p. 19
- ↑ Electronic beam swiveling with FMCW radar implemented in the Plextec Ltd. blighter
- ↑ Michael J. Heim and Andreas Mayr: Selection criteria for radar systems for level measurement in the chemical industry . February 2001, p. 3, endress.com (PDF)
- ↑ Norbert Thomann: Redefining reliability , level measurement with guided radar in the condensate container. (PDF) In: Verfahrenstechnik , 4/2011; Retrieved July 8, 2017
- ↑ Radar chip presented by the Karlsruhe Institute of Technology
- ↑ High frequency systems - new ways in quality control . (PDF) Fraunhofer Institute for High Frequency Physics and Radar Technology; Retrieved July 3, 2017
- ↑ WERA - Over the Horizon Radar. Retrieved July 20, 2012.
- ^ Robert F. Contreras, Stephen J. Frasier: High-Resolution Observations of Insects in the Atmospheric Boundary Layer. In: Journal of Atmospheric and Oceanic Technology. Volume 25, Issue 12, December 2008, pp. 2176–2187, manuscript from May 15, 2008 (PDF).
- ↑ А. М. Педак: Справочник по основам радиолокационной техники. 1967, p. 25 (Russian, German "Handbook on the Basics of Radar Devices").
- ↑ А. М. Педак: Справочник по основам радиолокационной техники. 1967, p. 27 (Russian, German "Handbook on the Basics of Radar Devices").
- ↑ Markus Becker: Radar with an invisibility cap. In: Spiegel Online from August 6, 2006.
- ↑ Administrative regulation for frequency assignments for radars and navigation systems (VVRadNav), bundesnetzagentur.de (PDF)