Monitor lizards
Monitor lizards | ||||||||||||
---|---|---|---|---|---|---|---|---|---|---|---|---|
![]() Black tie monitor ( V. salvator ), Malaysia (Borneo) |
||||||||||||
Systematics | ||||||||||||
|
||||||||||||
Scientific name of the family | ||||||||||||
Varanidae | ||||||||||||
Merrem , 1820 | ||||||||||||
Scientific name of the genus | ||||||||||||
Varanus | ||||||||||||
Merrem, 1820 |
The monitor lizards (lat. Varanus ) form a genus of over 80 species of the scalloped reptiles (Squamata) from the sub-order of the sneaky (Anguimorpha). They are found in the tropical and subtropical areas of Africa, Asia, and Australia and inhabit a wide variety of habitats. Most monitor lizards are elongated lizards with a pointed head and a long tail. Depending on the species, a length of 20 cm to 3 m is achieved. The largest lizard living today belongs to the genus of monitor lizards: the Komodo dragon . A striking feature of the monitor lizards is the long tongue, which is deeply split at the tip; it serves the olfactory perception when licking, which is probably the most important sense in monitor lizards.
All monitor lizards are active during the day and, depending on the species, spend the night in burrows they have dug themselves, tree hollows or similar shelters. The seasonal activity of many monitor lizards is shaped by the dry season in their habitats. During this time there is a lack of food and the monitor lizards survive the dry season in hiding.
There are ground-dwelling and tree-dwelling as well as partly water-dwelling (semiaquatic) species. Through special adaptations of the heart, lungs and ventilation , monitor lizards can take in far more oxygen than other scale crawfish, so they have more efficient breathing and are capable of a more active lifestyle and greater performance. Most monitor lizards search large areas for prey. Almost all monitor lizards are carnivores and feed on insects, other invertebrates or small to medium-sized vertebrates. Some species also eat carrion. Only three species in the Philippines eat fruit to a large extent in addition to animal food.
Some species of monitor lizards are of high economic importance for humans and are hunted for the leather trade and as meat suppliers, especially in Africa and Southeast Asia. While a number of species appear to be able to withstand this hunting pressure, other commercially used monitor lizard species have suffered considerable population losses. In addition, monitor lizards are often endangered by the loss of their habitat. In the Red List of Threatened Species of IUCN is a kind of a high risk classified, two other species as endangered . For most species, however, insufficient information on the endangerment status is available.
The name Waran and the generic name Varanus are etymologically derived from the Arabic wordورل / Waral from which stands for monitor lizards as a whole, and from the homonymous ancient Egyptian name for the Nile monitor ( V. niloticus ).
distribution and habitat
Monitor lizards live in the tropics , subtropics and, to a lesser extent, the temperate zones of the ancient world. The distribution area of the genus extends in Africa over almost the entire continent and extends from there over the Arabian Peninsula, Central Asia, continental Southeast Asia and the Southeast Asian island world to Australia. In addition, some species reach the westernmost archipelago of the Pacific, such as the Solomon Islands , the Marshall Islands , the Caroline Islands and the Mariana Islands . The most northerly occurrence of all monitor lizards has the desert monitor , whose distribution extends from North Africa and Central Asia to the Aral Sea and the Caspian Sea at about 46 ° north latitude. Monitor lizards are absent in Madagascar, Tasmania and New Zealand as well as in Europe, America and the Antarctic.
Monitor lizards have their greatest diversity in Australia; 30 species have been found there. In places up to eleven species occur sympatric . Another “hotspot” for monitor lizards is New Guinea, which shares five species with Australia. 16 species of monitor lizards are known in New Guinea, the Solomon Islands and the Bismarck Archipelago . Up to ten species could be found in the Moluccas , but the number is not certain. The Philippines is home to eight species, including all the fruit-eating species of the Philippinosaurus subgenus . Since 2010, three more species have been described from the Philippines. The occurrence of the rudicollis monitor ( V. rudicollis ) in the Philippines is not certain, together there would be 12 species. In the rest of the Malay archipelago there are 12 species, in continental Asia 7 species. Finally, there are 5 species in Africa.
In their large area of distribution, monitor lizards colonize a variety of habitats at various altitudes, for example rainforest, desert and mangroves . Some species also inhabit anthropogenic and urban areas. The tropical habitats of monitor lizards are characterized by seasonal fluctuations. Usually these can be divided into a nutrient-rich rainy season and a nutrient-poor dry season .
features
Appearance
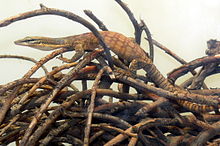
Monitor lizards have the basic shape of a lizard with four legs and a tail. The head is usually moderately high, comparatively long and tapering towards the muzzle; however, there are a number of species with a high skull or a short head and a blunt snout. Like snakes, monitor lizards have a long forked tongue that can be twice the length of the head. The eye has a round pupil . The nostrils are round or slit-shaped and can be directly at the tip of the snout, directly in front of the eye or in between. The position and shape of the nostril can be used to differentiate between individual species. The head is well set off from the rather long neck. The limbs are comparatively long and always have 5 fingers. The hind limbs are longer than the front limbs. The cross section of the tail is round in most species, but in some water-dwelling (semiaquatic) species the tail is laterally compressed as a row tail. In this way, it generates more drive in the water when it hits the side. The representatives of the V. prasinus group, on the other hand, have long, thin grasping tails that they use as a climbing aid. The tail length usually exceeds the head-trunk length . Monitor lizards look similar to the not closely related rail lizards (Teiidae).
The color of monitor lizards is highly variable and ranges from the bright green emerald monitor ( V. prasinus ) to the completely black ( melanistic ) Panay monitor ( V. mabitang ). Often there are point patterns or transverse changes, and the basic color is usually a camouflage color in the respective habitat. Vertical stripes are rare in monitor lizards. In most cases, the stomach side is lighter than the upper side of the body. In some species, young animals are significantly lighter or more colorful than old animals.
height and weight
Monitor lizards are known for their wide range in body size and weight: within no other genus of vertebrates is the difference between small and large species greater. The smallest monitor lizard is the short-tailed monitor ( V. brevicauda ), which can reach a maximum total length of 23 cm and a maximum weight of 17 g. The largest monitor lizard living today is the Komodo dragon ( V. komodoensis ), which can reach a maximum length of up to around 3 m with a weight of over 70 kg. The Komodo dragon is the largest lizard living today; Other medium-sized to large monitor lizards are also among the largest lizards living today. Within the monitor lizards, particularly large or small species developed independently of one another several times.
In most species, males become somewhat larger than females, as the growth rate of females decreases faster in the course of individual development ( ontogenesis ) than in males. Also, males sometimes have slightly different head and limb proportions, but most species cannot be sexed with certainty without further investigation.
Scaling
For the most part, monitor lizards have small, simply built scales . The head has small, polygonal scales. The snout shield ( rostral ) is only indistinctly developed. This also applies to the nasal shields ( nasals ) that are not very clearly defined ; instead, the nostrils are surrounded by many small scales. In some species, conspicuously enlarged scales are formed within the rows of the Überugenenschild ( Supraocularia ). The nuchal scales also experienced enlargement or tapering in some species; Examples are V. nuchalis and the Raunackenwaran ( V. rudicollis ). The scales of the trunk are elongated oval and arranged in fairly regular transverse rows. Many dwarf monitors of the subgenus Odatria have spiky scales on their tails . When the tails are pressed together laterally, the two uppermost rows of scales of the tail usually form keels. Some monitor lizards (e.g. Gray's monitor lizard, V. olivaceus ) also have specially keeled scales on the underside of the body in order to have additional support when climbing.
anatomy
skull

The large, relatively far back nasal windows are striking in the facial skull. The upper jaw bone (maxillary) is quite long. In the lower jaw, there is a wide suture filled with connective tissue between the lower jaw bone (dental) and some adjacent bones (coronoid, supraangular and angular) . The brain skull shows only a few special features.
The kinetic properties of their skull are important for monitor lizards, which means that they can swallow large prey or large pieces of them whole. This compensates for the lack of ability to chew. Cases have become known in which monitor lizards have devoured prey animals of 42% of their own body weight; However, such large prey is the exception. On the one hand, the lizard skull is metakinetic : the skull elements of the back of the head (occipital region) are movably connected to the rest of the skull, so the rest of the skull can be moved towards the back of the head. On the other hand, the monitor skull is also mesokinetic , the upper jaw can be moved against the brain skull at an articulated joint behind the eye windows ( orbit ). The connective tissue seam between some of the lower jaw bones also makes them movable against each other. As with all scaled reptiles, the waran skull is also characterized by streptostyly : the quadrate is articulated with its adjacent skull elements, which makes the skull more mobile.
teeth
In most species, the teeth of the monitor lizards are laterally compressed, taper to a point and are curved backwards. In some species the cutting edges are sawn. Teeth that have fallen out are renewed by forming new teeth in the back of the jaw and continuously moving up to vacant spaces ( polyphyodontics ). According to Mertens (1942), a complete waran bite has 37 to 71 teeth, depending on the species. The odd number results from the peculiarity that there is almost always an odd number of teeth on the intermaxillary bone (premaxillary). The number of teeth on the upper jaw roughly corresponds to the number on the lower jaw ; Usually there are 1–2 more in the lower jaw, but there can also be the same number or less. The teeth are attached sub pleurodont . In the case of monitor lizards, this means that a microscopic bony plate connects the teeth with the jawbone. Small monitor lizards use their teeth primarily to hold prey, while large monitor lizards use their teeth to cut out pieces from larger prey. The teeth are particularly well suited for this due to their sawing.
A number of monitor lizard species, on the other hand, develop blunt, brick-like teeth in the course of their individual development. While the juveniles of such species usually have pointed teeth for hunting insects, the adults feed mainly on mollusks (e.g. snails). This ontogenetic tooth change is known from the Nile monitor ( V. niloticus ), among others . This change of teeth is also typical for the Gray Monitor Lizard ( V. olivaceus ), which also eats fruit after the change of teeth.
Hyoid apparatus
The hyoid bone apparatus (the functional unit of the hyoid bone and attached muscles) of the monitor lizards corresponds in the basic pattern to the hyoid bone apparatus of most scale crawfish. The individual elements, however, are very mobile in relation to one another, and the muscles attached to the hyoid skeleton are unusually well developed. The hyoid bone can be moved in many different directions together with the floor of the mouth. The hyoid apparatus plays an important role in swallowing prey, breathing and threatening gestures.
Postcranial skeleton

The postcranial skeleton (skeleton excluding skull) is typically built for scale reptiles. The vertebrae are concave (procoel) at the front. The centers are pressed flat and therefore not cylindrical. After examining six specimens (four species), Mertens (1942) gives the following numbers: 7 cervical vertebrae , 1–2 posterior ones with short ribs , 22–23 dorsal vertebrae, except for the last 1–3 all with ribs, 2 without ribs Sacral vertebrae and 101–148 caudal vertebrae . Monitor lizards are not capable of autotomy , so they cannot shed their tails like lizards.
The upper arm bone ( humerus ) is short and squat, the Elle ( ulna ) is longer than the radius ( radius ). There are five toes, the phalangeal formula (number of phalanx bones per front toe) of the front legs is 2–3–4–5–3. At the thighbone ( femur ) of the joint head is shin ( trochanter tibialis ) is very large, the joint head of the fibula ( trochanter fibular ) very small. The fibula is slightly longer than the tibia . There are also five toes on the hind foot, the phalangeal formula of the hind legs is 2–3–4–5–4.
Ossification of the skin
Some species of monitor lizards have worm-shaped osteoderms under their scales , especially on the legs, the base of the tail, on the head, as well as on the neck and underside of the abdomen. In old specimens, the small osteoderms can grow together locally to form continuous armor. It is assumed that these bone structures represent a certain protection against injuries, among other things, in the case of intra-species conflicts. Another ossification of the integument is the hemibaculum, an ossified structure at the end of the hemipenes and hemiclitoris. The usefulness of this structure is unknown. The hemibaculum represents an autapomorphy of the monitor lizards.
Metabolic organs and physiology
heart

![]()
Diastole : The heart relaxes and fills with low-oxygen (blue) and high-oxygen (red) blood.
|
![]()
Systole : the heart contracts and pumps blood. The sealing of the muscle bar is symbolized here by the use of a darker color than before.
|
The unusually built organs heart and lungs are of particular importance for the monitor lizards . The monitor heart is located quite far back in the body and only begins after the rear end of the breastbone (sternum). As with all scaled reptiles, the monitor heart consists of two atria and three not completely separated chambers, namely Cavum arteriosum , C. venosum and C. pulmonale . C. arteriosum and C. ulcers be by a septum ( septum incompletely separated). C. venosum and C. pulmonale, on the other hand, are almost completely separated by the muscular ridge , but a small gap remains between the top of the muscular ridge and the heart wall. The C. venosum is relatively small. The two septal atrioventricular valves extend from the septum that separates the two atria . In the monitor heart, the well-developed muscle bar and the well-developed septa and valves are particularly important.
During the filling phase ( diastole ), oxygen-poor blood flows from the body's circulation from the right atrium into the C. venosum and from there through an opening between the muscle bar and the heart wall into the C. pulmonale . At the same time, oxygen-rich blood flows from the pulmonary circulation at higher pressure from the left atrium into the C. arteriosum . The left septal atrioventricular valve is pressed against the septum between C. arteriosum and C. venosum , creating a temporary, quite efficient functional separation between the two chambers. At the beginning of the expulsion phase ( systole ), the septal atrial ventricular valves are closed again by the rise in blood pressure in the heart chambers. In this way, the heart chambers are separated from the atria, which refill with blood. At the same time, the small gap between the muscle bar and the inner wall of the chamber is closed. With increasing chamber compression, the oxygen-poor blood of the C. pulmonale is pressed into the pulmonary circulation. At the same time, the oxygen-rich blood of the C. arteriosum is pressed into the body's circulation by the C. venosum, which was previously used for the oxygen-poor blood . In practice, the C. venosum only has the role of a conduit for blood, not the function of an autonomous ventricle. While the oxygen-poor blood of the C. pulmonale is pumped to the pulmonary circulation practically without mixing, the oxygen-rich blood of the C. arteriosum mixes with the oxygen-poor blood of the C. venosum , which did not reach the C. pulmonale . The advanced separation of deoxygenated and oxygenated blood in the heart enables more effective oxygen distribution. Only about 30% of the oxygen-poor blood intended for the pulmonary circulation gets back into the body circulation, and only about 10% of the oxygen-rich blood from the lungs gets back into the pulmonary circulation.
lung
The lungs of the monitor lizards are quite large. They start a considerable distance behind the heart and extend to the anterior end of the liver. The bronchi split into two branches in the lungs. From these, in turn, small side branches emerge, which open into spacious alveolus (pulmonary alveoli-rich) chambers. This multi-chambered building is an autapomorphy of the monitor lizards; other scale reptiles have simple, sac-like lungs. Thus there is a larger area for gas exchange in the lungs of monitor lizards, which is therefore particularly efficient with monitor lizards.
ventilation
The ventilation of the lungs of monitor lizards is also different to that of other scale crawlers. Most reptiles breathe primarily with the help of the intercostal muscles ( Musculi intercostales ). These muscles can turn the ribs slightly on both sides of the trunk, thereby expanding the chest and thus filling the lungs by increasing its volume. However, lateral undulations of the trunk play an important role in the locomotion of lizards. At medium and high speeds, the intercostal muscles are tense on one side to bend the trunk. As a result, the intercostal muscles contribute to locomotion, but at the same time breathing is hindered at increased speed, even though an increased oxygen supply would be important at this point. This is known as axial constraint ("axial constraint"). Monitor lizards are able to compensate the axial restriction with the help of the hyoid apparatus through gular pumping ("throat pumps"). At medium to high speeds, a normal breath is taken first, which cannot completely fill the lungs. Then the hyoid apparatus is lowered and the throat area is expanded and air is drawn in. Then the hyoid apparatus compresses the throat space again and pumps air into the lungs. On average, each normal breath is pumped three times. This form of breathing also occurs in other scale creeps, but is particularly pronounced in the monitor lizards.
Aerobic capacity
These adaptations result in a particularly high ability to absorb oxygen. Most scale creepers have only a low aerobic metabolic capacity and thus a low anaerobic threshold . For short, intense physical exertion, scale crawfish have a well-developed ability to provide anaerobic energy . However, scale crawlers tire quickly and the pH of the blood drops due to lactic acid production (a waste product of anaerobic metabolism). Metabolic acidosis develops when the patient is overworked . Monitor lizards, on the other hand, are known for their large aerobic capacities. In addition to special adjustments to ventilation, the lungs and the heart, the heart and skeletal muscles are also equipped with a high content of myoglobin , which means that additional oxygen can be stored. The hematocrit of monitor lizards is also higher than that of other lizards. These adaptations enable particularly high physical performance and a highly active way of life for scalp reptiles. In addition to their greater endurance, monitor lizards do not consume more energy than other lizards and snakes in their resting state.
This was confirmed in experiments in which the performance metabolism of steppe monitors ( V. exanthematicus ) and green iguanas ( Iguana iguana ) was compared at 35 ° C for 20–45 minutes of stress on a treadmill. The maximum oxygen consumption of the steppe monitor is 1.26 milliliters per gram of body weight of the animal per hour. This enables him to run at a constant speed of 1.2 km / h completely aerobically over the test period. For the green iguana, on the other hand, a maximum oxygen consumption of 0.82 milliliters per gram of the animal's body weight per hour was calculated, so that it could only maintain a completely aerobical rate of 0.5 km / h on the treadmill.
Species of monitor lizards that are particularly strongly bound to the water and regularly dive show further adaptations in terms of breathing . The blood of such species has a particularly high content of blood buffers , which allows the body to gain energy anaerobically over a long period of time. In addition, the heart rate can be reduced by up to 85% when diving.
Metabolic Rate, Water, and Fat Body
The metabolic rate of wild monitor lizards has been studied several times; the values ranged from 2.3 kJ / d in a 10.4 g caudolineatus vein to 1330 kJ / d in a 16.62 kg Komodo dragon ( V. komodoensis ). In between was z. B. a 3.28 kg Argus monitor ( V. panoptes ) with 468 kJ / d. In monitor lizards in highly seasonal areas, the metabolic rate is also subject to strong fluctuations, since most species then have a dormant period. In the dry season, the metabolic rate of the examined Argus monitor was only 168 kJ / d. The situation is similar with the water balance: In the rainy season the Argus monitor excreted 135 ml / d, in the dry season 58.5 ml / d. Especially monitor lizards from arid regions excrete only a little water with the urine. Monitor lizards cover their water needs almost exclusively from the water content of their prey.
In order to survive hunger periods with little prey, monitor lizards create fat bodies. One body of fat is found at the base of the tail, two more are opposite, flap-like structures on the underside of the body between the peritoneum and the abdominal wall . Monitor lizards feed on this fat especially during the dry season with little food; White-throated monitors ( V. albigularis ) lose up to 50% of their body weight during the dry season. In many monitor lizards, the females feed on their fatty bodies to form yolks for their eggs.
Sense organs and sensory services
Tongue and Jacobson organ
The monitor lizard's long, forked tongue plays an important role in olfactory perception . The tongue is repeatedly drawn in and stretched out as part of the tongue for olfactory perception. At the tip of the tongue, odor particles from the air or surfaces are picked up. The odor particles on the tip of the tongue are then transported to the Jacobson organ on the roof of the mouth. The Jacobson organ plays a role primarily in locating prey at greater distances and in social behavior, and in monitor lizards it is powerful enough to track down buried prey. The tongue is moved by various muscles: on the one hand by the genioglossus muscle , which attaches to the underside of the tongue and the lower jaw, and by muscles that extend from the inside of the tongue. Most of these start at the hyoid skeleton. The tongue is drawn into a sheath on the floor of the mouth, into which it almost completely fits.
eye
The eyes of the monitor lizards are very well developed for reptiles. Monitor lizards have an upper eyelid , a lower eyelid, and a nictitating membrane that can be drawn over the entire eye. In its fine structure, the monitor's eye is very similar to that of other diurnal reptiles. The retina of monitor lizards contains mostly or exclusively cones , so monitor lizards are able to see color. Each cone contains two oil droplets - this unusual property is probably a peculiarity of the monitor lizards. Since monitor lizards are diurnal and often inhabit habitats with high light intensity (e.g. deserts), these oil droplets are probably a kind of sun protection against harmful short-wave radiation.
Monitor lizards perceive movements particularly well and recognize, for example, a moving person from around 200 m away. Stationary objects are only seen out of focus. The field of view is around 240 °, 25 ° of which is stereoscopic . The sense of sight plays a role in avoiding enemies, in hunting at short distances and in social behavior. Sprackland (1999) even suspects that closely related monitor lizard species (e.g. in the V. indicus group) can differ in the colors of their tongue.
Further sense organs
The parietal organ (crown eye ) of the monitor lizard is well developed; outwardly it appears as a light spot on an enlarged scale, the so-called interparietal. The parietal organ plays a central role in thermoregulation (through sunbathing) and the daily rhythm; If the parietal organ is experimentally covered, the monitor lizards often sleep outside of hiding places, are not very active, are sometimes also active at night and no longer show any thermoregulatory behavior.
The monitor's hearing is not very efficient; many individuals also do not respond to loud noises (e.g. gunshot).
Poison
Recent studies suggest that all monitor lizards are poisonous. Fry et al. (2006) created cDNA libraries of serous tissue in the lower jaw of 4 species of monitor lizard and also examined the oral secretions of these species using mass spectrometry . In the DNA libraries of all species, genes were found that code for toxic proteins typical of reptile poisons. Mass spectrometry showed that the saliva of monitor lizards contains a whole range of toxic proteins that are also encoded in the DNA libraries. The toxic proteins are therefore most likely produced by poison glands in the lower jaw. The presence of poison glands would be shared by the monitor lizards with all members of the taxon Toxicofera . Another study by Fry et al. (2010) demonstrated poison production in 11 other species; it is therefore very certain that all monitor lizards have poison glands. Of the basic Toxicofera proteins, Warangift contains proteins from the AVIT family, natriuretic peptides (B-type), CRISP proteins, Cobra Venom Factor , Kallikrein and Nerve Growth Factor . Crotamine, cystatin and Vespryn were lost secondarily, but phospholipase A 2 (PLA 2 ) was added.
With the help of magnetic resonance imaging (MRT), venomous glands could be detected for the first time in the Komodo dragon ( V. komodoensis ) (Fry et al. 2009); later, identically constructed poison glands were found in a total of 15 species (Fry et al. 2010). Monitor lizards have an elongated poison gland on each side of the lower jaw that extends under the rows of teeth. These glands are each enveloped by connective tissue and divided into a large posterior and five smaller anterior compartments. A separate passage leads up from each compartment and opens into the oral cavity between two teeth. Monitor lizards have no furrowed or hollow teeth for poison transmission, so the poison mixes with other secretions in the oral cavity.
The effects and benefits of waran poison have not been adequately researched. The poison of the Komodo dragon has, however, been well researched: it primarily causes a volume deficiency shock , inhibits blood coagulation and thus supports the capture of prey (see also the role of poison and bacteria in hunting behavior in the Komodo dragon ). For other species there is still some uncertainty. If the oral cavity secretions of a spotted monitor ( V. varius ) are injected into an anesthetized rat, this leads to the relaxation of the smooth muscles of the aorta , as a result of which blood vessels expand considerably. The poison contained in the mixture also reduces blood clotting ( coagulopathy ). In a non-anesthetized rat, these effects would lead to a sharp drop in blood pressure and unconsciousness due to volume deficiency shock. However, most monitor lizards are very unlikely to be of any use in catching prey, as monitor lizards usually swallow their small to medium-sized prey alive or kill it with purely mechanical force. According to Arbuckle (2009), the poison is more likely to aid digestion through ingredients such as PLA 2 . The species-specific variations in the toxicity are unknown.
The effects of the poison on humans have not been adequately researched. In some cases, symptoms of poisoning after biting monitor lizards have been reported. According to Fry et al. (2006) led bites from the Komodo dragon ( V. komodensis ) Buntwaran ( V. varius ) and V. scalaris excessive swelling of the bite site, dizziness, stinging pain and bleeding disorder. The symptoms lasted for several hours. In addition, an exceptional case of the desert monitor ( V. griseus ) has been published in which palpitations ( tachycardia ), muscle weakness and breathing difficulties also occurred.
Locomotion

Like all lizards, monitor lizards walk on four legs with their limbs spread apart. The trunk is alternately bent to the side while walking ( undulation ). The front legs carry about 40% of the body weight, the rear legs about 60%. When running, monitor lizards keep their head and torso well above the ground, and only the back half of the tail and feet touch it. When monitor lizards walk in the sand, there are typical tracks with footprints and a dragging track in the middle. The remarkably long tail and the unusually long neck of the monitor lizards also play a role in locomotion. The long neck makes it easier to catch fast, agile prey due to its mobility. The tail acts as a kind of counterweight to the rapidly moving neck. The tail can also be shifted for a quick change of direction. Information on the speed of monitor lizards is rare: Gould's monitor lizards ( V. gouldii ) run over longer distances with an average of around 1.6 km / h, the speed can range from around 1.1 km / h to 2.4 km / h. The Komodo dragon ( V. komodoensis ) moves at an average of 4.8 km / h and can reach up to 18.5 km / h with short sprints.
Many monitor lizards are good climbers. Several species are known to be able to use their tails as a grasping organ when climbing. Many species are skilled swimmers. Swimming monitor lizards lay their legs loosely on the torso and generate propulsion through the lateral wave movements of the torso and tail. The head is kept above water. Some species also dive regularly and can sometimes stay under water for up to an hour. Regular dives are significantly shorter. The vast majority of monitor lizards are capable of both climbing and swimming, but the extent of these abilities varies from species to species and depends on the respective way of life. There are specialized tree-dwellers, ground-dwellers and species that are strongly bound to water, as well as generalists.
behavior
Activity, thermoregulation and hiding places

All monitor lizards are diurnal. In the evening monitor lizards retreat to their hiding place and rest until the next morning. Most monitor lizards sunbathe extensively in the morning. Depending on the climatic conditions in their area of distribution, monitor lizards hide from excessive heat around noon or are particularly active at this time due to optimal temperatures. Like all scaled reptiles, monitor lizards are ectothermic and increase their body temperature when they sunbathe, or they cool off by retreating to a hiding place, in the shade or in the water. Monitor lizards can also " pant " and lower their body temperature by quickly raising and lowering the floor of their mouth using the hyoid apparatus . however, this behavior leads to considerable water loss and is only used when there is an imminent risk of overheating. Active monitor lizards usually have a body temperature of 22–38 ° C. Most monitor lizards try to maintain a preferred temperature of around 36 ° C through active thermoregulation. The preferred temperature, however, differs from species to species and in particular semi-aquatic species prefer lower body temperatures. Some species are poorly prudent thermoregulators and still maintain a favorable body temperature. This is apparently related to greater light absorption by their skin.
Monitor lizards use many different types of hiding place, such as tree hollows, dense vegetation, crevices and termite mounds. The majority of species go digging a burrow for themselves or taking over the construction of another animal. Monitor lizards prefer to build their own burrow under rocks or roots. In some species, burrows that you dug yourself can be several meters long.
Monitor species from the northern and southern distribution areas usually have a dormant period. Species from North and South Africa, Australia and Central Asia in particular remain largely inactive in their habitat during the dry season, as there is a lack of food at this time. At the beginning of the dry season, the monitor lizards retreat into hiding and wait until the rainy season. Species from tropical areas, on the other hand, are often active all year round.
Spaces of action, movements and social behavior


Most individual monitor lizards move in an ancestral area, which can be described as an action space . The action spaces of males are usually much larger than the action spaces of females. The size of such action spaces also depends very much on the habitat and the monitor species. The action spaces of individuals overlap in most species, and the range is usually not defended. In nutrient-rich areas, the action areas can be smaller, whereas in nutrient-poor areas, larger areas must be used.
The daily distances covered by monitor lizards are highly variable, depending on the species and environment: The maximum length of 80 cm V. glauerti covers an average of 37 m daily, while the white-throated monitor ( V. albigularis ) , which is up to 1.5 m long, covers up to 5 km. With their higher aerobic capacity, monitor lizards are far more active than other lizards and their action areas are particularly large compared to similarly sized lizards.
All monitor lizards are solitary animals and the individuals in an area rarely meet. The Komodo dragon ( V. komodoensis ) is also known to actively avoid confrontation with dominant individuals. If monitor lizards nevertheless meet, conflicts often arise, since it is not uncommon for both monitor lizards to be attracted to one and the same resource, for example carrion, hiding places or reproductive partners. These conflicts are usually resolved with a comment fight. Such fights can occur in all gender constellations and the course of action varies from one species to another. In the beginning, threatening gestures are usually exchanged: The monitor lizards begin to lick intensely, nod their head and inflate their throats with the help of the hyoid apparatus. The rivals begin to hoist loudly (hissing, hissing) and in some cases straighten up slightly on their back legs. If none of the rivals is intimidated, the clinch phase begins , which can last from a few seconds to an hour. Both opponents stand up on their hind legs, put their front legs around the rival's shoulder area and try to wrestle each other down. Finally a monitor lizard is put down and the winner mounts the inferior specimen (pseudo-population). Such a fight establishes a short-term dominance relationship and the winner can use the contested resource. The comment fights mostly follow the basic pattern shown here, but can differ from species to species. In contrast to damage fights, none of the rivals suffer permanent physical damage in the comment fight, since there is no bite in the comment fight. Damage battles can also occur with monitor lizards, but are far less common. Not all monitor lizards include a clinch phase in the fight, even if this behavior is original in monitor lizards. The clinch phase was lost in the Indo-Asia B group (see Biogeographical development of today's groups ). The same is the case with the dwarf monitor lizards of the subgenus Odatria ; they carry out a wrestling match on the ground.
intelligence
Monitor lizards are considered comparatively intelligent and are often referred to as the most intelligent lizards of all. For example monitor lizards can count. This was proven with an experiment in which white-throated monitor lizards ( V. albigularis ) were accustomed to always find a given number of snails in a certain space. If a snail was removed after several times, the monitor lizards began to search the entire room for the missing snail after consuming all the snails present. The possibility of entering another chamber with snails was also not used. Only the addition of another snail calmed the monitor lizards. The monitor lizards noticed a missing snail in up to 6 snails. In another experiment, Gould's monitor lizard ( V. gouldii ), Papua dragon ( V. salvadorii ), and spotted monitor lizard ( V. varius ) were found to be intelligent. A monitor was thrown with a mouse, which it killed immediately. Then the monitor lizard was given another mouse: it interrupted the swallowing process and killed the new mouse. This could be repeated several times and in the end the monitor lizards ate all the killed mice. Monitor lizards can also become very tame in captivity and visually distinguish their keepers from other people. There are also reports of Nile monitors ( V. niloticus ) that cooperated in the hunt. Krebs (2007) concludes from his observations and the available literature that monitor lizards can solve problems, learn from experience and have a good memory. He also attributes monitor lizards to be able to generalize, categorize and differentiate between similar individuals.
nutrition

Food spectrum
Most monitor lizards are carnivores and hunt a wide variety of prey, both invertebrates and vertebrates . Typical prey objects include a wide variety of arthropods (especially insects and spiders), mollusks (e.g. snails), crustaceans , fish, amphibians (especially frogs), reptiles, birds (especially young birds) and small mammals. In addition, the eggs of reptiles and birds are captured, and a number of monitor lizards also eat carrion.
The food spectrum of an individual monitor strongly depends on its size, its environment and the season. Small species and juveniles of large species feed almost exclusively on small invertebrates, and invertebrates usually make up the bulk of the prey in large species. In addition, larger monitor lizards also hunt small to medium-sized vertebrates; energetically, these are then often just as important as the invertebrates. All monitor lizards hunt prey that is smaller than themselves, even if prey animals can make up around 20% of their own body weight. Only the Komodo dragon ( V. komodoensis ) hunts large mammals such as deer or water buffalo.
An exception among the largely carnivorous monitor lizards is the subgenus Philippinosaurus with the 3 species Gray monitor lizard ( V. olivaceus ), Panay monitor lizard ( V. mabitang ) and V. bitatawa . In addition to a certain proportion of small invertebrates, these species almost exclusively eat fruits, preferably from screw trees ( pandanus ). The three species of Philippinosaurus developed a pronounced appendix . It contains symbiotic bacteria and facilitates the digestion of the plant food. In all other monitor lizards, the appendix is missing or only rudimentary .
Loot acquisition
Most species of monitor lizards flickly search large areas for food. With their high endurance due to their high oxygen capacity, they spend a relatively large part of their time looking for food. During their search, in turn, they spend a lot of time examining promising places such as roots, piles of leaves, burrows or hollow tree trunks. Prey hidden in leaves or the like is digested with the snout or the forelegs. In the vicinity, monitor lizards rely on the sense of sight to hunt: as soon as the prey moves, it is grabbed in a rapid forward movement. Small prey are swallowed alive, large prey are shaken to death or hit against hard objects. Quite a few monitor lizards also hunt in trees. In contrast, some water-dwelling species are looking for u. a. walking on the bottom of the water and licking for prey. Still other species swim freely in the water when hunting and sometimes also actively pursue fish while swimming. Likewise, semi-aquatic monitor lizards can move their tails to the side by moving their tails sharply sideways. They can also transport fish onto land or into shallow water, where they are easy to catch. With their sense of smell, monitor lizards track down buried reptile nests, which they dig up. Only a few monitor lizards seem to be ambulance hunters; However, little is known about their hunting behavior. The hunting behavior of monitor lizards correlates with their species-specific metabolic rate and aerobic capacity: while species with a high metabolic rate search large areas, the ambush hunters are characterized by their rather low aerobic capacities.
Prey is usually swallowed head first and whole. The thin tongue of the monitor lizard is not able to move a prey object in the mouth towards the esophagus . Initially, the head is stretched up using gravity in order to carry the prey into the widened gullet. If this is not enough, the prey is pushed further down the throat with the help of rocks or the surface of the earth as a resistance, or the monitor lizard uses the hyoid apparatus to further manipulate the prey. First, the floor of the mouth is lifted with the help of the hyoid bone apparatus. Then the hyoid apparatus is withdrawn and relaxed again. In this way, the prey object is drawn towards the throat region and esophagus. As soon as the prey object is even deeper in the throat, the movement pattern changes: the hyoid bone apparatus is moved in front of the prey object. Then the hyoid apparatus is raised to compress the throat region. Then the hyoid apparatus is pulled back and the prey object is pushed towards the esophagus. Larger prey is partially fixed with the forelegs and then torn individual pieces out of it with the jaws. The muscles of the hyoid bone apparently play no role when drinking.
Reproduction and development
Mating behavior
The mating usually only takes place during a short time window of the year; Species from areas particularly close to the equator, however, have quite extended mating seasons. In most species, the females apparently secrete pheromones , which attract the males. These fragrances are most likely produced in so-called preanal glands, which are stored in the skin in front of the cloaca. Of other species, it is known that the sexes often meet on carrion. During the mating season, the males are particularly active and cover unusually long distances in search of females. When they find a female, the males of most species woo the potential mate by poking, scratching, and nudging their snout. The mating proceeds as with most lizards: the male mounts the female, puts his front legs around her shoulders and leads a hemipenis from the side into the cloaca of the female. In some species, the females defend themselves against the first attempts at mating by beating or biting the males; the males of these species fixate the females particularly strongly with their front legs. It is also known that some species do not mate immediately, but only 1–2 days after they meet. If several males meet with one female, then it comes to a fight for comments about the right to mate.
Clutch, egg laying, hatching and development of the young animals
Gestation lasts for 4–6 weeks in captivity for most species. In wild animals, the clutches comprise on average 2.5–24.5 eggs, depending on the species. The African species are particularly fertile. Monitor lizard eggs have a leather-like shell and weigh on average 3.1–131.9 g, depending on the species. The size and number of eggs in a clutch increase with the size of the female and the food available. In highly pregnant females, the entire clutch can make up around 50% of the body weight. The time of oviposition varies greatly from species to species and with the climate in their area of distribution. Usually the eggs are laid in such a way that the young hatch at the height of the rainy season; so they have the highest possible food supply after birth. The females of ground-dwelling species usually lay their eggs in self-dug and then backfilled nesting holes (often dug in embankments), tree-dwelling species often in tree hollows. Quite a number of species lay their eggs in termite mounds , tree-dwelling monitor lizards also in the nests of tree-dwelling termites. To do this, a tunnel is dug into the hill and at the end a chamber is hollowed out to lay eggs. The females only partially fill the hole; the termites close up the rest. In the termite burrows, the humidity and temperature are much more stable than outside the burrows, and the eggs are largely safe from predators.
The average incubation period (incubation period) at 30 ° C is 91.7–226 days from species to species (Thompson & Pianka 2001, 33 species). In nature, incubation times of up to 300 days occur in some species, so the eggs survive the dry season and hatch during the next rainy season. In monitor lizards, the sex is determined genetically: the females are hemizygous with a WZ pair of sex chromosomes, the males are homozygous with ZZ ( ZW / ZZ system ). Young animals hatched in termite burrows dig themselves free in some species, but it is known of other species that nest in termite burrows that the females dig the hatched young animals out of the hard burrow. Apparently, the females remember their egg-laying site and return there regularly. No further details are known about this maternal care. Young animals can survive in a termite mound for 2–3 weeks by feeding on their yolk. Otherwise monitor lizards show no care for their young.
There is hardly any data available on the growth of monitor lizards in nature. In captivity, small species often reach their almost final size after around a year and then grow only very slowly. Large species, on the other hand, often grow beyond the age of 5 years. Sexual maturity occurs sooner or later, depending on the species, in the Komodo dragon ( V. komodoensis ) for example after 5 years and in the short-tailed monitor ( V. brevicauda ) sometimes after 10 months.
parthenogenesis
So far, parthenogenesis has been reported in three species of monitor lizards , so it is likely that all species have this ability. Cases of parthenogenesis are very rare and reports have only come from captive females who have not had contact with males. Parthenogenesis occurs automatically in monitor lizards with terminal fusion, which means that initially a completely normal meiosis takes place. So there are haploid germ cells. Using special polar bodies, haploid germ cells then merge with the same genetic material again to form diploid zygotes (i.e. self-fertilization). As a consequence, all chromosome pairs consist of the same chromosomes, including the sex chromosomes. In monitor lizards, the ZW system is used for sex determination; Females have the sex chromosome pair ZW, males ZZ. A young animal with two W sex chromosomes dies in the egg. Therefore, only males hatch, these have received two Z chromosomes from their mother. Since, to a certain extent, only "half" of the maternal genome is expressed, numerous genes are homozygous . The effects of otherwise recessive , deleterious mutations can be compared to 10 to 15 generations of inbreeding between siblings. Male animals often die in the egg and only in rare cases are parthenogenetically produced monitor lizards viable. The utility of parthenogenesis remains highly speculative.
Natural enemies, enemy avoidance and life expectancy
Most monitor lizards chase after snakes, other monitor species, birds of prey and predatory mammals. Aquatic species are also hunted by large predatory fish. Adult specimens of large species, on the other hand, have few enemies. In addition, monitor lizards are attacked by various ecto- and endoparasites; the most common are ticks from the genera Aponomma and Amblyomma and roundworms from the genus Abbreviata .
Mostly monitor lizards flee from enemies in a hiding place, but especially larger species can react aggressively to enemies. They show threatening behavior similar to that of other species and hiss loudly. Monitor lizards defend themselves against enemies with bites and blows with their tail. According to some reports, tails of large specimens can break the legs of dogs. Most species flee immediately from humans, and the large Komodo dragon ( V. komodoensis ) only bites when cornered. Only a few monitor lizards are known to play dead.
Data on mortality and life expectancy are rare. Young monitor lizards in particular are likely to have an extraordinarily high mortality rate in nature. Data on life expectancy are practically only known from captivity, where monitor lizards have proven to be comparatively long-lived. Age around 10 years has been documented for several species. The Komodo dragon ( V. komodoensis ) can probably live to be over 30 years old, and specimens of small dragon species have been kept in captivity for up to 20 years in exceptional cases.
Systematics
A cladogram of the closer relationship of the monitor lizards according to Pyron et al., 2013
|
External system
The genus of monitor lizards ( Varanus ) forms the family Varanidae with some extinct genera. The closest recent relative is the Borneo Taubwaran ( Lanthanotus borneensis ), which is classified in an independent, monotypical family (Lanthanotidae). Varanidae and Lanthanotidae form the sister group of the Shinisauridae with only one recent species, the crocodile tail lizard ( Shinisaurus crocodilurus ). The clade from these three families, together with the crusty lizards (Helodermatidae), the humpback lizards (Xenosauridae), sneaks (Anguidae) and ringed snakes ( Anniellidae), form the group of creeps (Anguimorpha), which together with the iguanas (Iguania) and snakes ( Serpentes) form the group of Toxicofera within the scale reptiles (Squamata).
The relationship between the monitor lizards and the Cretan aigialo and mosasaurs is unresolved , even if they were often placed near the monitor lizards in the past.
Internal system
The German herpetologist Robert Mertens (1894–1975) is considered to be the founder of the monitor system . In 1942, three pioneering works by Mertens on monitor lizards were published in the treatises of the Senckenberg natural research society . Mertens' system is now considered outdated, but his work paved the way for modern systematists.
Currently about 80 recent species are recognized as valid within the monitor lizards . At the moment these are divided into 9 sub-genera . This division was charged with investigations of hemipenis morphology by the German herpetologist Wolfgang Böhme and Thomas Ziegler in 1997 established previously some work published by Boehme. They established the subgenus Soterosaurus (Ziegler & Böhme, 1997) and used old subgenus or redefined them, namely Empagusia (Gray, 1838), Euprepiosaurus (Fitzinger, 1843), Odatria (Gray, 1838), Papusaurus (Mertens, 1958), Philippinosaurus (Mertens, 1959), Polydaedalus (Wagler, 1830), Psammosaurus (Mertens, 1942) and Varanus (Merrem, 1820). Within the Empagusia a V. bengalensis group is separated, in Euprepiosaurus a distinction is made between V. prasinus and V indicus groups , Odatria divided into V. acanthurus group and V. timorensis group, Polydaedalus is made up of a V. niloticus Group and a V. exanthematicus group together and in Varanus a V. gouldii group is secreted. With the exception of a few points, this classification agrees with later molecular biological investigations. Nevertheless, the subdivision based on the hemipenis structure has established itself, and the generic names are taken up again in molecular biological studies. Since not all monitor lizards have been genetically examined, no purely molecular-biological based system is in use.
A complete list of all species is available under Systematics of monitor lizards. The list can be sorted according to sub-genera, and the monitor species can also be arranged according to results from molecular biology.
Phylogeny
|
Biogeographical development of today's groups
While the fossil record of monitor lizards is very sparse, molecular biological studies (e.g. Ast 2001, Fitch et al. 2006, Ziegler et al. 2007, Amer & Kumazawa 2008) and some important fossils (Hocknull et al. 2009) the development history of monitor lizards can be reconstructed. The spread of the monitor lizards was probably facilitated by their good locomotion skills. The rising and falling sea levels during the Pleistocene certainly had a major influence on the spread of monitor lizards . During the ice ages, glaciers bound large quantities of seawater, and as a result, sea levels fell by up to 100 m. Due to the rather flat sea in the Indo-Australian island world, the spread in the archipelagos was favored.
Most likely, the monitor lizards originated in Central Asia or Africa. This is supported on the one hand by the mtDNA analyzes by Ast (2001), according to which all 6 African species form the sister group to the other monitor lizards, on the other hand also the fossil record. Primitive crawlers were found predominantly in Central Asia, and the oldest known fossils of monitor lizards are 37 million year old eddies from Egypt. According to the molecular clock by Amer & Kumazawa (2008), the genus of monitor lizards split off about 60 million years ago.
So basal Varanus migrated either from Central Asia to South Asia and Africa or first to Africa and from there to Asia. According to Ast (2001), the non-African monitor lizards are divided into 2 large clades: the Indo-Asia cladus and the Indo-Australian cladus. The Indo-Asia cladus is divided into 2 large groups: Indo-Asia A and Indo-Asia B. These two lines of development separated about 51 million years ago. Indo-Asia A on the one hand contains the already postulated by Ziegler & Böhme (1997) subgenus Empagusia which up to the Raunackenwaran ( V.rudicollis is) monophyletic. Instead, this is the sister taxon to the so-called V. salvator complex (with Ziegler & Böhme Soterosaurus ), which together with V. rudicollis is the sister taxon to Empagusia excl. V. rudicollis is. On the one hand, Empagusia developed in Southeast Asia and the Sunda Archipelago , on the other hand a rudicollis -like species. The extent of genetic variability within different V. salvator -species and Ast's (2001) analyzes suggest that a rudicollis -like species was found during a period of globally low sea level immigrated to the Philippines during the Pleistocene. This is where today's V. rudicollis and V. nuchalis emerged with also rough neck scales, while a V. cumingi -like shape was the origin for radiation to the south and west. Numerous species of the V. salvator complex emerged from this radiation (e.g. V. salvator , V. togianus ).
In Indo-Asia B the first split took place about 34 million years ago. This resulted in the Filipino subgenus Philippinosaurus and the subgenus Euprepiosaurus . Euprepiosaurus in all probability developed in the Moluccas; due to the poor fossil record, however, this is only one of several possibilities. The sub-genus Euprepiosaurus is divided into the gracefully built, tree-dwelling V. prasinus group and the V. indicus group, which is often bound to the water , which is more powerfully built and has tails compressed at the sides. This highly diverse group migrated from the Moluccas to New Guinea and the Solomon Islands, and some species even as far as Australia. The exact speciation processes remain unexplained, only the monophyly of the two groups has been confirmed. Allopatric speciation probably played a decisive role on the numerous small islands and established the diversity and the high degree of endemism .
The Indo-Australian clad reached Australia about 15 million years ago; the Lesser Sunda Islands are a possible expansion corridor . Initially, three groups formed in very rapid speciation: On the one hand the dwarf monitor lizards of the subgenus Odatria , on the other hand the two sister groups V. gouldii and V. varius group. The split between small and enormous body size is basal within the Australian monitor lizards. The speciation rate has decreased to this day, but happened very quickly after colonization. Most species of monitor lizards still live in Australia today. Odatria is split into the tree-dwelling V. tristis group and the ground-dwelling V. acanthurus group. The V. gouldii group contains all typical large Australian monitor lizards except for the spotted monitor ( V. varius ). This is closer to the Varanus Salvadorii ( V. salvadorii ) on New Guinea and the Komodo dragon ( V. komodoensis related). This very large group (colored monitor 2 m, Papua monitor and Komodo dragon> 2.5 m) developed in Australia. During the low sea levels during the Pleistocene, some representatives of this group emigrated again from Australia. The gradual spread to the northwest via Timor and the Lesser Sunda Islands to Java can be documented on the fossil record. Only recently have representatives of the V. varius group died out in large parts of their distribution, so that today only V. varius in Australia and V. salvadorii and V. komodoensis outside Australia remain. Some monitor lizards of the V. gouldii group later reached New Guinea again; this includes B. a subspecies of the Argus monitor ( V. panoptes horni ).
Monitor lizards and the Wallace line

The evolution of the monitor lizards was significantly influenced by the Wallace line . There are no predators (Carnivora) east of this biogeographical boundary . To the west of the Wallace Line there are mainly large species of monitor lizard and very few small species. East of the Wallace line, however, the number of small monitor lizard species (e.g. V. prasinus group and Odatria ) increases enormously, while the number of small predatory mammals (e.g. martens) decreases sharply. Large species of monitor lizards can exist west of the Wallace Line because they have many young and these grow quickly. Thus, these species are resistant to hunting pressure from small to medium-sized predatory mammals. Small species are exposed to this pressure at any age. In Wallacea, however, they could and still exist today. At the same time, they occupy the ecological niche of small predatory mammals.
Fossil record

The fossils of monitor lizards are usually vertebrae, and overall the fossil record is very sparse. In Africa only one named fossil monitor lizard is known with V. rusingensis , which is at the same time the oldest monitor lizard ever described with a binomial name . The Kenyan fossil dates from the early Miocene . Monitor lizards also lived in Europe, with the pliocene V. marathonensis being particularly widespread . V. hofmanni was found in Germany and France's Miocene . In Europe, the monitor lizards probably died out in the course of a cooling climate during the Ice Ages. In Asia and Australia, paleontologists also found some fossils of extinct species that are still alive today, especially some very large species. The best known fossil monitor lizard is V. priscus , which lived in the Pleistocene of Australia and reached a length of about 6 m. On the basis of its skull structure, V. priscus can be assigned to the V. varius group.
Importance to humans

Monitor lizards are important for the international leather trade; In addition to the often attractive pattern, monitor leather is also durable and is processed into watch straps, shoes, purses, handbags and other goods. In addition, the meat of monitor lizards is an important food for the local population: the liver and tail as well as the eggs of the animals are preferred. In Asia in particular are Asian Water Monitor ( V. salvator ), Yellow Monitor ( V. flavescens ) and Bengal monitor ( V. bengalensis ) hunted in Africa especially the Nile monitor ( V. niloticus ), the savannah monitor ( V. Exanthematicus ) and the white-throated monitor ( V . albigularis ). Important exporters in Asia are Indonesia, the Philippines, Thailand, Bangladesh, India and Pakistan, in Africa Nigeria, Sudan, Mali and Cameroon. Monitor lizards are not subject to human persecution in Australia. The number of specimens killed each year for some species is likely in the millions. Several thousand specimens of some species are caught annually for the pet trade, in particular the steppe monitor ( V. exanthematicus ) and the Nile monitor ( V. niloticus ) are important in this regard. Commercial breeding of monitor lizards would be very unprofitable compared to hunting wild specimens, so all monitor skins come from wild-caught specimens. Occasionally, the practices of the leather industry are also criticized; in Southeast Asia, white monitor monitors are often skinned alive due to insufficient methods of killing (hammer blows on the head). Before that, the animals are stored for days with their legs tied together. In addition, a number of ethnic groups make traditional medicine from monitor lizards. Monitor lizards make themselves useful as eaters of pests such as rodents and insects. On the other hand, they are often unpopular for attacking small pets like chickens.
In addition, there are cultural references to monitor lizards in numerous ethnic groups, both positive and negative. 10,000 year old drawings of monitor lizards are known from caves near Bhopal (India). The Bugis and Macassars on Sulawesi have a particularly unusual relationship with monitor lizards : They see monitor lizards as twins of their children and ascribe them a human soul. The lizards are involved in everyday family life.
Some types of monitor lizards are popular in terraristics . However, this is also associated with massive live imports of wild-caught animals and sometimes irresponsible or inappropriate keeping, for example due to poor nutrition or insufficient accommodation.
Hazard and protection
The IUCN Red List of IUCN has entries for 20 species of monitor lizards. Of these 20 species, the IUCN describes 13 as least concern , 1 as near threatened and 3 as not sufficiently known ( data deficient ). As endangered ( vulnerable ) the be Komodo dragon ( V. komodoensis ) and the Gray's monitor ( V. olivaceus ) out, and the Panay Monitor ( V. mabitang ) is classified as endangered strong. The Washington Convention on the Protection of Species (CITES) sets trade restrictions on all monitor lizard species and products made from them, but these are sometimes ignored. 5 species are listed in Appendix I, all remaining species in Appendix II.
A number of monitor lizard species are threatened by habitat destruction, habitat fragmentation and hunting, but some particularly heavily hunted species seem to withstand the pressure of persecution through high reproduction rates and are therefore not considered endangered (e.g. Nile monitor , V. niloticus & Bindenwaran , V. salvator ) . Likewise, many species are very adaptable and therefore only marginally threatened by habitat destruction, while others suffer severe population losses. Small monitor lizard species, such as representatives of the subgenus Odatria in Australia, can be decimated by predatory neozoa such as domestic cats. In Australia, larger amphibian-hunting monitor lizards suffered severe declines due to the naturalization of the cane toad ( Bufo marinus ). The lizards do not know this poisonous toad and die from its skin toxin. It is sufficient to take the toad in its mouth when catching prey. Populations of large monitor lizard species often decrease by around 90% when aga toads migrate into their habitat.
Web links
- Varanus in The Reptile Database
- Varanus on the IUCN Red List of Threatened Species2010.
- varanidae.org - Website of the International Varanid Interest Group (IVIG).
- monitor-lizards.net - website that deals with keeping monitor lizards .
- VaranID - identification aid for monitor lizards .
- ncbi.nlm.nih.gov - GenBank entry for monitor lizards .
literature
- JC Ast (2001): Mitochondrial DNA Evidence and Evolution in Varanoidea (Squamata) . Cladistics 17: 211-226.
- W. Auffenberg (1981): The Behavioral Ecology of the Komodo Monitor . University Presses of Florida, Gainesville.
- W. Auffenberg (1988): Gray's Monitor Lizard . University Presses of Florida, Gainesville.
- W. Auffenberg (1994): The Bengal Monitor . University of Florida Press.
- D. Bennett (1996): Monitor lizards of the world. World of monitor lizards . Edition Chimaira, Frankfurt am Main. ISBN 3-930612-05-4 .
- W. Böhme (2003): Checklist of the living monitor lizards of the world (family Varanidae) . Zoological Negotiations 341: 3-43.
- W. Böhme & T. Ziegler (2008): A review of iguanian and anguimorph lizard genitalia (Squamata: Chamaeleonidae; Varanoidea, Shinisauridae, Xenosauridae, Anguidae) and their phylogenetic significance: comparisons with molecular data sets . Journal of Zoological Systematics and Evolutionary Research 47 (2): 189-202.
- AJ Fitch, AE Goodman & SC Donnellan (2006): A molecular phylogeny of the Australian monitor lizards (Squamata: Varanidae) inferred from mitochondrial DNA sequences . Australian Journal of Zoology 54: 253-269.
- H.-G. Horn & W. Böhme (1991, eds.): Advances in Monitor Research . Mertensiella 2.
- H.-G. Horn & W. Böhme (1999, eds.): Advances in Monitor Research II . Mertensiella 11.
- H.-G. Horn, W. Böhme & U. Krebs (2007, eds.): Advances in Monitor Research III . Mertensiella 16. ISBN 978-3-9806577-9-2 .
- DR King & B. Green (1999): Monitors: The Biology of Varanid Lizards . Krieger Publishing Company, Malabar. ISBN 1-57524-112-9 .
- A. Koch, M. Auliya & T. Ziegler (2010): Updated checklist of the living monitor lizards of the world (Squamata: Varanidae) . Bonn Zoological Bulletin 57 (2): 127-136
- R. Mertens (1942): The family of monitor lizards (Varanidae). First part: general . Treatises of the Senckenberg Natural Research Society 462: 1–116.
- R. Mertens (1942): The family of monitor lizards (Varanidae). Part two: the skull . Treatises of the Senckenberg Natural Research Society 465: 117–234.
- ER Pianka & DR King (2004, eds.): Varanoid Lizards of the World . Indiana University Press, Bloomington & Indianapolis. ISBN 0-253-34366-6 .
- T. Ziegler & W. Böhme (1997): Genital structures and mating biology in squamatic reptiles, especially the Platynota, with comments on the system . Mertensiella 8: 3-210.
- T. Ziegler, A. Schmitz, A. Koch & W. Böhme (2007): A review of the subgenus Euprepiosaurus of Varanus (Squamata: Varanidae): Morphological and molecular phylogeny, distribution and zoogeography, with an identification key for the members of the V. indicus and the V. prasinus species groups . Zootaxa 1472: 1-28.
supporting documents
- ↑ a b c d Varanus in The Reptile Database ; accessed on May 13, 2020.
- ↑ a b c d e Varanus in the IUCN Red List of Threatened Species 2010. Accessed March 1, 2011.
- ^ A b c E. R. Pianka & DR King (2004): Introduction . In: ER Pianka & DR King (eds.): Varanoid Lizards of the World : 3–9. Indiana University Press, Bloomington & Indianapolis. ISBN 0-253-34366-6 .
- ↑ a b c d e f Div. Art chapter in ER Pianka & DR King (2004, eds.): Varanoid Lizards of the World . Indiana University Press, Bloomington & Indianapolis. ISBN 0-253-34366-6 .
- ↑ a b c T. Ziegler, A. Schmitz, A. Koch & W. Böhme (2007): A review of the subgenus Euprepiosaurus of Varanus (Squamata: Varanidae): Morphological and molecular phylogeny, distribution and zoogeography, with an identification key for the members of the V. indicus and the V. prasinus species groups . Zootaxa 1472: 1-28.
- ^ G. Dryden & T. Ziegler (2004): Varanus indicus . In: ER Pianka & DR King (Eds.): Varanoid Lizards of the World : 184-188. Indiana University Press, Bloomington & Indianapolis. ISBN 0-253-34366-6 .
- ↑ M. Stanner (2004): Varanus griseus . In: ER Pianka & DR King (Eds.): Varanoid Lizards of the World : 184-188. Indiana University Press, Bloomington & Indianapolis. ISBN 0-253-34366-6 .
- ↑ a b c d e f g h i j k l m n o p R. Mertens (1942): The family of monitor lizards (Varanidae). First part: general . Treatises of the Senckenberg Natural Research Society 462: 1–116.
- ↑ a b c d S. S. Sweet & ER Pianka (2007): Monitors, Mammals and Wallace's Line . Mertensiella 16 ( Advances in Monitor Research III ): 79-99.
- ↑ VS-A. Weijola (2010): Geographical Distribution and Habitat Use of Monitor Lizards of the North Moluccas . Biawak 4 (1): 7-23.
- ↑ VS-A. Weijola & SS Sweet (2010): A new melanistic species of monitor lizard (Reptilia: Squamata: Varanidae) from Sanana Island, Indonesia . Zootaxa 2434: 17-32.
- ↑ a b A. Koch, M. Gaulke & W. Böhme (2010): Unraveling the underestimated diversity of Philippine water monitor lizards (Squamata: Varanus salvator complex), with the description of two new species and a new subspecies . Zootaxa 2446: 1-54.
- ↑ a b c L. J. Welton, CD Siler, D. Bennett, A. Diesmos, MR Duya, R. Dugay, EL Rico, M. Van Weerd, RM Brown: A spectacular new Philippine monitor lizard reveals a hidden biogeographic boundary and a novel flagship species for conservation. In: Biology letters. Volume 6, number 5, October 2010, pp. 654-658, doi : 10.1098 / rsbl.2010.0119 , PMID 20375042 , PMC 2936141 (free full text).
- ↑ MK Bayless (2002): Monitor lizards: a pan-African check-list of their zoogeography (Sauria: Varanidae: Polydaedalus) . Journal of Biogeography 29 (12): 1643-1701.
- ↑ a b c E. R. Pianka (1995): Evolution of body size: varanid lizards as a model system . The American Naturalist 146 (3): 398-414.
- ^ A b E. R. Pianka (2004): Varanus brevicauda . In: ER Pianka & DR King (Eds.): Varanoid Lizards of the World : 312-317. Indiana University Press, Bloomington & Indianapolis. ISBN 0-253-34366-6 .
- ↑ a b C. Ciofi (2004): Varanus komodoensis . In: ER Pianka & DR King (Eds.): Varanoid Lizards of the World : 197-204. Indiana University Press, Bloomington & Indianapolis. ISBN 0-253-34366-6 .
- ↑ a b c d J. C. Ast (2001): Mitochondrial DNA Evidence and Evolution in Varanoidea (Squamata) . Cladistics 17: 211-226.
- ↑ a b c A. J. Fitch, AE Goodman & SC Donnellan (2006): A molecular phylogeny of the Australian monitor lizards (Squamata: Varanidae) inferred from mitochondrial DNA sequences . Australian Journal of Zoology 54: 253-269.
- ↑ D. Frynta, P. Frýdlová, J. Hnízdo, O. Šimková, V. Cikánová & P. Velenský (2010): Ontogeny of Sexual Size Dimorphism in Monitor Lizards: Males Grow for a Longer Period, but not at a Faster Rate . Zoological Science 27 (12): 917-923.
- ^ JG Smith, BW Brook, AD Griffiths & GG Thompson (2007): Can Morphometrics Predict Sex in Varanids? Journal of Herpetology 41 (1): 133-140.
- ↑ a b c d E. R. Pianka (2004): Varanus olivaceus . In: ER Pianka & DR King (Eds.): Varanoid Lizards of the World : 220-224. Indiana University Press, Bloomington & Indianapolis. ISBN 0-253-34366-6 .
- ↑ a b B. Weavers (2004): Varanus varius . In: ER Pianka & DR King (Eds.): Varanoid Lizards of the World : 488-502. Indiana University Press, Bloomington & Indianapolis. ISBN 0-253-34366-6 .
- ^ W. Maier, L. Olsson & A. Goldschmid (2004): Kopf . In: R. Rieger & W. Westheide (Ed.): Special Zoology Part 2: Vertebrate or skull animals . Spectrum Academic Publishing House, Heidelberg & Berlin. ISBN 3-8274-0307-3 .
- ↑ R. Mertens (1942): The family of monitor lizards (Varanidae). Part two: the skull . Treatises of the Senckenberg Natural Research Society 465: 117–234.
- ↑ DC D'Amore & RJ Blumenschine (2009): Komodo monitor (Varanus komodoensis) feeding behavior and dental function reflected through tooth marks on bone surfaces, and the application to ziphodont paleobiology . Paleobiology 35 (4): 525-552.
- ↑ a b M. Stanner (2010): Mammal-like Feeding Behavior of Varanus salvator and its Conservational Implications . Biawak 4 (4): 128-131.
- ^ S. Lenz (2004): Varanus niloticus . In: ER Pianka & DR King (Eds.): Varanoid Lizards of the World : 133-138. Indiana University Press, Bloomington & Indianapolis. ISBN 0-253-34366-6 .
- ^ A b c d e K. K. Smith (1986): Morphology and Function of the Tongue and Hyoid Apparatus in Varanus (Varanidae, Lacertilia) . Journal of Morphology 187 (3): 261-287.
- ↑ a b c T. Owerkowicz, CG Farmer, JW Hicks, EL Brainerd (1999): Contribution of Gular Pumping to Lung Ventilation in Monitor Lizards . Science 284: 1661-1663.
- ↑ a b V. L. Bels, J.-P. Gasc, V. Goosse, S. Renous & R. Vernet (1995): Functional analysis of the throat display in the sand goanna Varanus griseus (Reptilia: Squamata: Varanidae) . Journal of Zoology 236 (1): 95-116.
- ^ A b c d e f W. Auffenberg (1981): The behavioral ecology of the Komodo monitor . University Press of Florida, Gainesville.
- ↑ a b G. M. Erickson, de A. de Ricoles, V. Buffrenil, RE Molnar & MK Bayless (2003): Vermiform bones and the evolution of gigantism in Megalania-how a reptilian fox wurde a lion . Journal of Vertebrate Paleontology 23 (4): 966-970.
- ↑ H. Fuchs (1977): Histology and microscopic anatomy of the skin of the conjunctival monitor . Stuttgart Contributions to Natural History Series A (Biology) 299: 1–16.
- ^ A b W. Böhme (2004): Squamata, Schuppenkriechtiere . In: W. Westheide & R. Rieger (Eds.): Special Zoology Part 2: Vertebrate or skull animals : 358–380. Spectrum Academic Publishing House, Heidelberg & Berlin. ISBN 3-8274-0900-4 .
- ↑ a b c d G. Webb, H. Heatwole & J. De Bavay (1971): Comparative cardiac anatomy of the reptilia. I. The chambers and septa of the varanid ventricle . Journal of Morphology 134 (3): 335-350.
- ↑ N. Heisler, P. Neumann & GM O Maloiy (1983): The mechanism of intracardiac shunting in the lizard Varanus exanthematicus . Journal of Experimental Biology 105: 15-31.
- ↑ a b c d e f g h i j k l m n o p D. R. King & B. Green (1999): Monitors: The Biology of Varanid Lizards . Krieger Publishing Company, Malabar, 2nd ed. ISBN 1-57524-112-9 .
- ^ HO Becker, W. Böhme & SF Perry (1989): The lung morphology of the monitor lizards (Reptilia: Varanidae) and their systematic, phylogenetic significance . Bonn Zoological Contributions 40: 27–56. ( online ; PDF; 3.5 MB)
- ↑ T. Owerkowicz, EL Brainerd & DR Carrier (2001): electromyographic pattern of the gular pump in monitor lizards . Bull. Mus. Comp. Zool. 156: 237-248.
- ↑ MSAD al-Ghamdi, JFX Jones & EW Taylor (2001): Evidence of a functional role in lung inflation for the buccal pump in the agamid lizard, Uromastyx aegyptius microlepis . Journal of Experimental Biology 204: 521-531.
- ↑ X-ray of gular pumping ( MOV ; 3.0 MB)
- ^ AF Bennett (1973): Blood physiology and oxygen transport during activity in two lizards, Varanus gouldii and Sauromalus hispidus . Comparative Biochemistry and Physiology A 46: 673-690.
- ^ AF Bennett (1973): Ventilation in two species of lizards during rest and activity . Comparative Biochemistry and Physiology A 46: 673-690.
- ↑ a b T. T. Gleeson, GS Mitchell & AF Bennett (1980): Cardiovascular responses to graded activity in the lizards Varanus and Iguana . In: American Journal of Physiology-Regulatory, Integrative and Comparative Physiology 239: R174-R179.
- ↑ a b c d G. G. Thompson (1999): Goanna Metabolism: Different to Other Lizards, and if so, What are the Ecologocial Consequences? Mertensiella 11 ( Advances in Monitor Research II ): 79-90.
- ↑ SC Wood & K. Johansen (1974): Respiratory adaptations to diving in the nile monitor lizard, Varanus niloticus . Journal of Comparative Physiology A 89 (2): 145-158.
- ↑ B. Green & K. Christian (2007): Field Metabolic Rates (FMRs) and Water Fluxes in Free-living Varanid Lizards; A review . Mertensiella 16 ( Advances in Monitor Research III ): 240-246.
- ↑ a b c d e f g h i j k l m n o p q r s t u v w x y z D. Bennett (1996): Warane der Welt. World of monitor lizards . Edition Chimaira, Frankfurt am Main. ISBN 3-930612-05-4 .
- ↑ a b J. A. Phillips (1995): Movement patterns and density of Varanus albigularis . Journal of Herpetology 29: 407-416.
- ↑ B. Röll & H.-G. Horn (1999): The structure of the eye of the monitor lizard Varanus griseus caspius (Reptilia, Varanidae) . Mertensiella 11 ( Advances in Monitor Research II ): 291-306.
- ^ RG Sprackland (1999): Character Displacement among Sympatric Varanid Lizard Species: A Study of Natural Selection and Systematics in Action (Reptilia: Squamata: Varanidae) . Mertensiella 11 ( Advances in Monitor Research II ): 113-120.
- ↑ C. Traeholt (1997): Effect of masking the parietal eye on the diurnal activity and body temperature of two sympatric species of monitor lizards, Varanus s. salvator and V. b. nebulosus . Journal of Comparative Physiology B 167: 177-184.
- ↑ a b c BG Fry, N. Vidal, JA Norman, FJ Vonk, H. Scheib, SFR Ramjan, S. Kuruppu, K. Fung, SB Hedges, MK Richardson, WC Hodgson, V. Ignjatovic, R. Summerhayes & E Kochva (2006): Early evolution of the venom system in lizards and snakes . Nature 439: 584-588.
- ↑ a b BG Fry, K. Winter, JA Norman, K. Roelants, RJA Nabuurs, MJP van Osch, WM Teeuwisse, L. van der Weerd, JE McNaughtan, HF Kwok, H. Scheib, L. Greisman, E. Kochva , LJ Miller, F. Gao, J. Karas, D. Scanlon, F. Lin, S. Kuruppu, C. Shaw, L. Wong & WC Hodgson (2010): Functional and Structural Diversification of the Anguimorpha Lizard Venom System . Molecular & Cellular Proteomics 9: 2369-2390.
- ^ BG Fry & H. Scheib (2007): Venom Breathing Dragons: The Molecular Diversity of Protein Toxines in Varanid Venoms . Mertensiella 16 ( Advances in Monitor Research III ): 10-24.
- ↑ a b BG Fry, S. Wroe, W. Teeuwisse, MJP van Osch, K. Moreno, J. Ingle, C. McHenry, T. Ferrara, P. Clausen, H. Scheib, KL Winter, L. Greisman, Kim Roelants, L. van der Weerd, CJ Clemente, E. Giannakis, WC Hodgson, S. Luz, P. Martelli, K. Krishnasamy, E. Kochva, HF Kwok, D. Scanlon, J. Karas, DM Citron, EJC Goldstein , JE Mcnaughtan & Janette A. Norman (2009): A central role for venom in predation by Varanus komodoensis (Komodo Dragon) and the extinct giant Varanus (Megalania) priscus . PNAS 106 (22): 8969-8974.
- ↑ a b K. Arbuckle (2009): Ecological Function of Venom in Varanus, with a Compilation of Dietary Records from the Literature . Biawak 3 (2): 46-56.
- ^ A b H. Preuschoft, U. Witzel, B. Hohn, D. Schulte & C. Distler (2007): Biomechanics of Locomotion and Body Structure in Varanids with Special Emphasis on the Forelimbs . Mertensiella 11 ( Advances in Monitor Research III ): 59-78.
- ^ A. Christian (2007): Scaling Effects in Monitor Lizards and Consequences for the Evolution of Large Size in Terrestrial Vertebrates . Mertensiella 16 ( Advances in Monitor Research III ): 1-9.
- ^ JO Farlow & ER Pianka (2000): Body Form and Trackway Pattern in Australian Desert Monitors (Squamata: Varanidae): Comparing Zoological and Ichnological Diversity . PALAIOS 15 (3): 235-247.
- ^ GG Thompson (1995): Foraging patterns and behaviors, body postures and movement speed for goannas, Varanus gouldii (Reptilia: Varanidae), in a semi-urban environment . Journal of the Royal Society of Western Australia 78: 107-114.
- ↑ a b K. Christian (2004): Varanus panoptes . In: ER Pianka & DR King (Eds.): Varanoid Lizards of the World : 423-429. Indiana University Press, Bloomington & Indianapolis. ISBN 0-253-34366-6 .
- ↑ H.-G. Horn (2007): Transmission and Reflection Spectra of Shed Skin of Various Varanids in the UV, VIS and IR and their Intra- and Interspecific Meaning . Mertensiella 16 ( Advances in Monitor Research III ): 291-303.
- ^ A b c E. R. Pianka (1994): Comparative Ecology of Varanus in the Great Victoria Desert . Australian Journal of Ecology 19: 395-408.
- ↑ M. Gaulke & H.-G. Horn (2004): Varanus salvator (nominate form) . In: ER Pianka & DR King (Eds.): Varanoid Lizards of the World , pp. 244-257. Indiana University Press, Bloomington & Indianapolis. ISBN 0-253-34366-6 .
- ^ SS Sweet (2004): Varanus glauerti . In: ER Pianka & DR King (Eds.): Varanoid Lizards of the World : 366–372. Indiana University Press, Bloomington & Indianapolis. ISBN 0-253-34366-6 .
- ↑ a b c d R. L. Earley, O. Attum & P. Eason (2002): Varanid combat: perspectives from game theory . Amphibia-Reptilia 23: 469-485.
- ^ GW Schuett, RS Reiserer & RL Earley (2009): The evolution of bipedal postures in varanoid lizards . Biological Journal of the Linnean Society 97 (3): 652-663.
- ^ A b U. Krebs (2007): On Intelligence in Man and Monitor: Observations, Concepts, Proposals . Mertensiella 16 ( Advances in Monitor Research III ): 44-58.
- ↑ a b c H.-G. Horn (1999): Evolutionary Efficiency and Succes in Monitors: A Survey on Behavior and Behavioral Strategies and some Comments . Mertensiella 11 ( Advances in Monitor Research II ): 167-180.
- ↑ a b c d e f J. B. Losos & HW Greene (1988): Ecological and evolutionary implications of diet in monitor lizards . Biological Journal of the Linnean Society 35 (4): 379-407.
- ^ W. Auffenberg (1988): Gray's Monitor Lizard . University Presses of Florida, Gainesville.
- ^ A b M. Gaulke, AV Altenbach, A. Demegillo & U. Struck (2007): On the Diet of Varanus mabitang . Mertensiella 16 ( Advances in Monitor Research III ): 228-239.
- ^ PJ Mayes, GG Thompson & PC Whiters (2005): Diet and foraging behavior of Varanus mertensi (Reptilia: Varanidae) . Wildlife Research 32: 67-74.
- ^ R. Shannon & RW Mendyk (2009): Aquatic Foraging Behavior and Freshwater Mussel (Velesunio sp.) Predation by Varanus panoptes panoptes in Central-Western Queensland . Biawak 3 (3): 85-87.
- ↑ H. de Lisle (2007): Observations on Varanus s. Salvator in North Sulawesi . Biawak 1 (2): 59-66.
- ↑ LJ Mendis Wickramasinghe, LDC Bhathiya Kekulandala, PI Kumara Peabotuwage & DMS Suranjan Karunarathna (2010): A Remarkable Feeding Behavior and a New Distribution Record of Varanus salvator salvator (Laurenti, 1768) in Eastern Sri Lanka . Biawak, 4 (3): 93-98.
- ^ KH Andres, M. von Düring & H.-G. Horn (1999): Fine Structure of Scale Glands and Preanal Glands of Monitors Varanidae . Mertensiella 11 ( Advances in Monitor Research II ): 277-290.
- ^ A b G. G. Thompson & ER Pianka (2001): Allometry of Clutch and Neonate Sizes in Monitor Lizards (Varanidae: Varanus) . Copeia 2001 (2): 443-458.
- ↑ P. Rismiller, MW & S. McKelvey Steinlechner (2007): Temperature and humidity in Egg Incubation Mounds of Varanus rosenbergi . Mertensiella 16 ( Advances in Monitor Research III ): 353-363.
- ↑ a b c R. Wiechmann (2011): Own observations on parthenogenesis in monitor lizards . elaphe 19 (1): 55-61.
- ^ B. Green, M. McKelvey & P. Rismiller (1999): The Behavior and Energetics of Hatchling Varanus rosenbergi . Mertensiella 11 ( Advances in Monitor Research II ): 105-112.
- ↑ PW Lenk, B. Eidenmüller, H. Stauder, R. Wicker & M. Wink (2005): A parthenogenetic Varanus . Amphibia-Reptilia 26: 507-514.
- ↑ PC Watts, KR Buley, S. Sanderson, W. Boardman, C. Ciofi & R. Gibson (2006): Parthenogenesis in Komodo Dragons . Nature 444: 1021-1022.
- ↑ J. Hennessy (2010): Parthenogenesis in an Ornate Nile Monitor, Varanus ornatus . Biawak 4 (1): 26-30.
- ↑ MK Bayless (2007): A Revised List of Ixodid Ticks Known to the Yemen Monitor (Varanus yemenensis); with a Review of the Ixodid Tick (Ixodoidea) Species Known to African and Arabian Monitor Lizards [Varanidae: Polydaedalus] . Biawak 1 (2): 67-72.
- ↑ a b Robert Alexander Pyron, Frank T. Burbrink & John J. Wiens: A phylogeny and revised classification of Squamata, including 4161 species of lizards and snakes. BMC Evolutionary Biology 2013, 13:93 doi: 10.1186 / 1471-2148-13-93
- ↑ Lanthanotus borneensis in The Reptile Database
-
↑ Some works on the systematics of the creeping species:
- JC Ast (2001): Mitochondrial DNA Evidence and Evolution in Varanoidea (Squamata) . Cladistics 17: 211-226.
- JL Conrad (2008): Phylogeny And Systematics Of Squamata (Reptilia) Based On Morphology . Bulletin of the American Museum of Natural History 310: 1–182.
- JL Conrad, O. Rieppel & L. Grande (2008): Re-assessment of varanid evolution based on new data from Saniwa ensidens Leidy, 1870 (Squamata, Reptilia) . American Museum Novitates 3630: 1–15.
- JL Conrad, JC Ast, S. Montanari & MA Norell (2010): A combined evidence phylogenetic analysis of Anguimorpha (Reptilia: Squamata) . Cladistics 26: 1-48.
- BG Fry, N. Vidal, JA Norman, FJ Vonk, H. Scheib, SFR Ramjan, S. Kuruppu, K. Fung, SB Hedges, MK Richardson, WC Hodgson, V. Ignjatovic, R. Summerhayes & E. Kochva (2006 ): Early evolution of the venom system in lizards and snakes . Nature 439: 584-588.
- MSY Lee (2005): Molecular evidence and marine snake origins . Biology Letters 1: 27-30
- O. Rieppel & H. Zaher (2000): The braincases of mosasaurs and Varanus, and the relationships of snakes . Zoological Journal of the Linnean Society 129: 489-514.
- N. Vidal & SB Hedges (2004): Molecular evidence for a terrestrial origin of snakes . Proceedings of the Royal Society of London B 271: 226-S229.
- ↑ a b A. Koch, M. Auliya & T. Ziegler (2010): Updated checklist of the living monitor lizards of the world (Squamata: Varanidae) . Bonn Zoological Bulletin 57 (2): 127-136.
- ^ A b T. Ziegler & W. Böhme (1997): Genital structures and mating biology in squamous reptiles, especially the Platynota, with comments on the systematics . Mertensiella 8: 3-210.
- ^ W. Böhme (2003): Checklist of the living monitor lizards of the world (family Varanidae) . Zoological Negotiations 341: 3-43.
- ↑ W. Böhme & T. Ziegler (2008): A review of iguanian and anguimorph lizard genitalia (Squamata: Chamaeleonidae; Varanoidea, Shinisauridae, Xenosauridae, Anguidae) and their phylogenetic significance: comparisons with molecular data sets . Journal of Zoological Systematics and Evolutionary Research 47 (2): 189-202.
- ^ A b c d R. E. Molnar (2004): The Long and Honorable History of Monitors and Their Kin . In: ER Pianka & DR King (Eds.): Varanoid Lizards of the World : 10-67. Indiana University Press, Bloomington & Indianapolis. ISBN 0-253-34366-6 .
- ↑ a b c d e f E. Arida & W. Böhme (2010): The Origin of Varanus: When Fossils, Morphology, and Molecules Alone Are Never Enough . Biawak 4 (4): 117-124.
- ↑ African Varanid Species . In: ER Pianka & DR King (Eds.): Varanoid Lizards of the World , p. 90. Indiana University Press, Bloomington & Indianapolis. ISBN 0-253-34366-6 .
- ↑ RB Holmes, AM Murray, YS Attia, EL Simons & P. Chatrath (2010): Oldest known Varanus (Squamata: Varanidae) from the Upper Eocene and Lower Oligocene of Egypt: support for an African origin of the genus . Palaeontology 53 (5): 1099-1110.
- ↑ a b c d e S. AM Amer & Y. Kumazawa (2008): Timing of a mtDNA gene rearrangement and intercontinental dispersal of varanid lizards . Genes, Genetics and Systematics 83: 275-280.
- ↑ T. Ziegler, M. Gaulke & W. Böhme (2005): Genital Morphology and Systematics of Varanus mabitang Gaulke & Curio, 2001 (Squamata: Varanidae) . Current Herpetology 24 (1): 13-17.
- ^ SA Hocknull, PJ Piper, GD van den Bergh, RA Due, MJ Morwood & I. Kurniawan (2009): Dragon's Paradise Lost: Palaeobiogeography, Evolution and Extinction of the Largest-Ever Terrestrial Lizards (Varanidae). PLoS ONE 4 (9): e7241.
- Jump up ↑ JJ Head, PM Barrett & EJ Rayfield (2009): Neurocranial osteology and systematic relationships of Varanus (Megalania) prisca Owen, 1859 (Squamata: Varanidae) . Zoological Journal of the Linnean Society 155 (2): 445-457.
- ↑ a b V. de Buffrénil & G. Hémery: Harvest of the Nile monitor, Varanus niloticus, in Sahelian Africa. Part I: Impact of Intensive Harvest on Local Stocks . Mertensiella 16 ( Advances in Monitor Research III ): 181-194.
- ↑ AP Pernetta (2009): Monitoring the Trade: Using the CITES Database to Examine the Global Trade in Live Monitor Lizards (Varanus spp.) . Biawak 3 (2): 37-45.
- ↑ A. Koch (2010): Bestial treatment of large Indonesian reptiles for Western luxury products . Reptilia 15 (6): 3, 6.
- ^ I. Das (1989): Indian monitor lizards: A review of human utilization patterns . Hamadryad 14 (1): 16-19.
- ↑ J.-B. Fauvel & A. Koch (2009): Zoo-Ethnological Observations in Southwest Sulawesi, Indonesia: a case study of Kembar Buaya ("Monitor Twins") . Biawak, 3 (3): 77-80.
- ↑ H.-G. Horn (2004): Keeping Monitors in Captivity: A Biological, Technical, and Legislative Problem . In: ER Pianka & DR King (Eds.): Varanoid Lizards of the World : 556-571. Indiana University Press, Bloomington & Indianapolis. ISBN 0-253-34366-6 .
- ↑ a b Appendices I, II and III. Cites , accessed March 28, 2011 .
- ↑ A. Koch (2010): Underestimated and exploited: systematics, diversity and endemism of Southeast Asian black monitors . Koenigiana 4 (1): 27-41.
- ↑ JS Doody, B. Green, R. Sims & D. Rhind (2007): A Preliminary Assessment of the Impacts of Invasive Cane toads (Bufo marinus) on Three Species of Varanid Lizards in Australia . Mertensiella 16 ( Advances in Monitor Research III ): 218-227.
- ↑ B. Ujvari & T. Madsen (2009): Increased mortality of naive varanid lizards after the invasion of non-native cane toads (Bufo marinus) . Herpetological Conservation and Biology 4 (2): 248-251.