heart

The heart ( Latin Cor , Greek Kardia , καρδία , or Latinized Cardia ) is a hollow muscular organ ( hollow muscle ) found in various animal groups , which pumps blood or hemolymph through the body with contractions and thus ensures the supply of all organs . More developed hearts, for example in vertebrates , work like a displacement pump in that the liquid (blood) is sucked in valve-controlled from blood vessels (in mammals, cavernous or pulmonary veins) and expelled through other blood vessels (in mammals, pulmonary trunk or aorta).
The study of structure, function and diseases of the heart is cardiology . A life without a heart is not possible for higher animals and humans - but with an artificial heart . The heart is one of the first organs created during embryonic development and can stand out as a crucial point . The New High German heart goes back to ahd. Herza .
etymology
The common Germanic word Middle High German hërz [e] , ahd. Herza , goes back to idg. Kē̌rd- , which can be traced back to the same etymological origin as the Latin and Greek forms, whereby in German the two consonants end at the beginning and at the end of the word a change due to the first and second sound shift .
Research history
Ancient thinkers such as Empedocles (5th century BC), Aristotle (4th century BC), Diocles of Karystus (4th or 3rd century BC) and most of the Stoics saw the heart as the central organ of the body and starting point for blood vessels and nerves. On the other hand, they only assumed the brain's task to cool down the heat localized in the heart.
The Greek philosopher Alkmaion recognized that it was not the heart that had the role it was assigned in antiquity as the central organ of perception and knowledge, but the brain, which he also made responsible for the movements in the body. Alkmaion's theory was followed by Plato and the author of the Corpus Hippocraticum . Even if this doctrine was confirmed by the Alexandrian physicians Herophilos of Chalcedon and Erasistratos , the older ideas of the heart as the central organ remained for a long time.
The Arab physician Ibn an-Nafis (1213-1288) was the first to describe the heart anatomically correctly. The English physician William Harvey (1578–1657) showed that the contractions of the heart drive the movement of blood through the circulation.
“The heart of living beings is the foundation of their life, the prince of all of them, the little world of the sun, on which all life depends, all of which radiates freshness and strength. Likewise, a king is the foundation of his kingdoms and the sun of his little world, the heart of the state, from which all power radiates, all grace emanates. I dared to dedicate this writing about the movement of the heart to His Majesty (as is the custom of this time) all the more because [...] almost all human deeds, as well as most deeds of a king, are carried out under the inspiration of the heart. "
Heart types and their distribution in the animal kingdom
Tubular hearts and chamber hearts
The hearts of the various animal groups can be divided into tubular and chambered types with regard to their structure.
Tubular hearts set the blood or hemolymph in motion by causing waves of contraction to run through their walls ( peristalsis ). In this way, a directed flow can be created even if there are no valves. Arthropods have tubular hearts that lie close to their backs. In insects and some crabs such as Artemia they can extend over longer body sections, in other crustaceans it is short muscular sacs. The blood or the hemolymph usually enters through openings at the side, so-called ostia . These can be quite numerous, the mantis shrimp have 13 pairs. Sometimes the inflow also happens from behind via veins . When the heart contracts, the fluid is forced forward into a valved artery . The heart is hung by ligaments or muscles that are put under tension by the contraction of the heart. When the heart relaxes, the ostia open and the heart expands so that fluid can flow in. The arthropod heart can therefore be compared to a suction pump .
Tubular heart come also with the Urochordata and embryos of vertebrates before. In the tunicate Ciona , the direction of the contraction waves changes rhythmically, so that the direction of blood flow alternates. This is also the case with insects. The visual observation of the heart of resting mosquitoes of the species Anopheles gambiae shows that it contracts at a rate of 1.37 Hz (82 beats per minute) and the direction of contraction changes, with 72% of the contractions in the anterograde direction (towards the head ) and 28% of the contractions are in a retrograde direction (towards the tip of the abdomen).
In hearts with chambers, one chamber contracts completely. Flowing in the wrong direction is prevented by flaps that only open in one direction. This type of heart occurs particularly in molluscs and vertebrates. Due to the very strong wall muscles, these hearts also act as a pressure pump that can generate high blood pressure . In many molluscs, especially snails, but also in lower vertebrates, the filling of the heart works through negative pressure in the pericardial cavity that surrounds the heart. The wall of this cavity can be very solid, so that when the heart contracts, a negative pressure is created which sucks blood into the heart after the contraction has ended. In sharks, this creates −5 mm water column . The chamber (also: ventricle) has a thick, muscular wall. Upstream of it is the atrium, which has weaker wall muscles and which fills the chamber through its contraction.
Myogenic and Neurogenic Hearts
The heart contraction is triggered by an electrical impulse. In myogenic hearts, this impulse is triggered spontaneously and rhythmically in specialized heart muscle cells, the pacemaker cells. This occurs in vertebrates, tunicates, molluscs and some annelids and arthropods (including insects). In mammals and birds , the responsible cells were located in the sinus node . The total electrical activity of a myogenic heart can be shown in an electrocardiogram (EKG). The EKG is typical for each species.
In neurogenic hearts, the impulse to contract is triggered by nerve cells (more precisely: ganglion cells ) that are attached to the heart. Such neurogenic automatism occurs in some annelids and some arthropods, such as the decapods , which include lobsters, crabs, and other groups. The responsible heart ganglion can have nine or 16 cells, depending on the species. Also, the horseshoe crab Limulus and the tarantula Erypelma californicum have a neurogenic heart. While in myogenic hearts the excitation from the pacemaker cells spreads through neighboring muscle cells in the entire heart, such a muscular excitation transmission does not take place in neurogenic hearts as far as is known. Instead, the muscle cells are innervated in many ways. In Limulus , each muscle cell is innervated by six or more nerve cells, the origin of the ganglia, which lie on the back of the heart and control the excitation.
Myogenic hearts are also often innervated, for example in molluscs and vertebrates. Both myogenic and neurogenic hearts can be controlled by the nervous system. The pacemaker cells, for example, can be stimulated or inhibited by appropriate nerve impulses, so that the heart rate is increased or decreased, depending on the physical requirements.
In arthropods, long hearts are more often neurogenic and short hearts are more myogenic. In general, both types of heart beat independently, without a signal transmitter from the central nervous system. This is known as autonomy or autorhythmy.
Sideline
Most molluscs have an open vascular system with a heart, atrium and ventricle. In cephalopods , which have a largely closed vascular system, there are two gill hearts in addition to the main heart, which press the blood through the capillaries of the gills. So they have a similar function to the right half of the heart in mammals, which drives the pulmonary circulation.
In the case of myxins , a genus of the hagfish , there are portal hearts, cardinal hearts and caudal hearts in addition to the main heart. There are also secondary hearts in the wing veins of bats . In the lymphatic system of anurans called lymph hearts occur. They are created in pairs near the coccyx and have a neurogenic automatism. However, it does not have its own pacemaker. Instead, they are controlled by the autonomic nervous system. Lymph hearts are also found in some reptiles and birds, such as ostriches , but not in most birds and mammals.
Many insects have additional hearts in their wings, legs, and antennae that help force the hemolymph through these narrow appendages. Up to a few dozen of these accessory hearts can occur.
Other organs that promote blood
In addition to hearts, other organs also contribute to the flow of blood in some species. In the legs of the terrestrial vertebrates, the contraction of the muscles leads to an improved return flow of the venous blood to the heart. The movement of the body of the arthropod sets the hemolymph in motion. In some types of blood vessels occur, which can contract, for example, when Nautilus Nautilus where they pump the blood through the gills to the heart.
Heart rate
In general, the heart rate of large species within a group of animals is lower than that of smaller species. This has been shown for example for mammals, crustaceans or arachnids . In mammals, the values for fully grown animals at rest are between 6 beats per minute for the blue whale and 1000 beats per minute for the Etruscan shrew . An exception to the rule is the giraffe , which with 170 beats per minute has a significantly higher frequency than animals of comparable size.
In animals of the same temperature , the frequency is higher than in animals of the same size as cold-blooded animals . Like the respiratory rate , the heart rate is related to the metabolic rate . In related species of the same size and activity, the lazy ones have a slower heart rate than the livelier ones. In birds, crabs and pulmonary snails, the relationship between increasing body mass ( ) and decreasing heart rate ( ) can be described using the following allometric equation :
where is a constant specific to the animal group and is −0.27 for birds, −0.12 for crabs and −0.11 for snails. Basically, such comparisons relate to adult animals.
The frequency of the heartbeat ( heart rate ) is not only decisive for the amount of blood that is pumped through the heart, but also the amplitude between the expansion and contraction of the heart muscles, i.e. the heartbeat volume . The interaction of the two results (as a product ) in the cardiac output .
Blood pressure
The blood pressure is the pressure against which the heart must eject its contents. It is therefore crucial for the work that the heart has to do. In animals with a closed blood circulation , the level of blood pressure is directly related to the output of the heart. The cardiac output is the quotient of blood pressure and peripheral resistance . This is not the case in animals with an open circulatory system . Since the hemolymph also flows through the body cavity , the blood pressure here is on the one hand comparatively low and on the other hand depends on the body's movement and posture and is therefore very variable.
In the molluscs it was shown that the pressure that can be built up by the ventricle is greater in the groups of animals with a more active lifestyle. In cephalopods , up to 600 mm water column has been measured in octopus (corresponds to 44 mm Hg ), in the snail patella 50 mm water column (3.7 mm Hg) and in mussels usually below 20 mm water column (1.5 mm Hg).
In vertebrates , blood pressure is highest in the bird's circulatory system , closely followed by the mammalian circulatory system. The other vertebrate groups, which do not have a complete separation between the pulmonary circulation and the body circulation (see below), have significantly lower blood pressures (see table). In birds and mammals, blood pressure increases with age and is slightly higher in males than in females. In mammals that hibernate , blood pressure drops sharply. Unless otherwise stated, the figures in the table are based on the textbook cited. First, the resting blood pressure at the end of the heart contraction (systolic blood pressure) is given, which corresponds to the pressure in the (left) ventricle, and followed by a slash is the pressure in the aorta at the beginning of the next contraction against which the heart has to eject the blood (diastolic Blood pressure). All values in mm Hg .
Mammals
|
Birds
|
Cold-blooded vertebrates
|
Heart of the vertebrates
All vertebrate hearts are myogenic, a pacemaker ensures that the heart generates its own stimuli. In many cases, hearts keep beating under controlled conditions after they have been dissected from an animal. This property is known as autorhythmy or autonomy.
Wall structure
The walls of the vertebrate hearts are made up of several layers. The heart is surrounded by the pericardium (pericardium). The outermost layer of the heart is the epicardium and inward follows the outer connective tissue of the heart. If there are coronary arteries (coronary arteries), that's where they are. They then extend from here into the underlying muscle layer, the myocardium . This is where the heart muscle cells , the cardiomyocytes, are located. The innermost layer is the endocardium , a layer of connective tissue that is closed off from the inside of the heart by a layer of epithelial cells.
The muscle layer comes in two forms, as compact or cancellous (spongy) myocardium. The respective proportion of both types is species-specific. Fish and amphibians have mainly cancellous myocardium, while mammals have almost only compact myocardium. In contrast to compact ones, cancellous myocardium often has no blood vessels; it is supplied by the blood in the heart. The cancellous myocardium can be drawn into the heart chamber in trabeculae or trabeculae.
fishes
The heart of the fish collects the blood from the body and pushes it into the gills with strong pressure . These functions can be found in the structure. Of the four chambers lying one behind the other, the two rear chambers, the sinus venosus and the atrium (atrium), collect the blood. From these two thin-walled spaces, the blood is first directed into the muscular ventricle. With the plate gills (Elasmobranchii, sharks and rays) the muscular Conus arteriosus (also Bulbus cordis ) follows . Your heart sac (pericardium) is stiff, so that a negative pressure is created by squeezing out the blood . This helps with the filling for the next cycle.
In the real bony fish (Teleostei), the Conus arteriosus has largely regressed. Instead, they developed the bulb arteriosus from the beginning of the aorta , a structure that contains many elastic fibers and smooth muscle cells . It creates a strong windkiller effect to maintain blood pressure.
The contraction wave arises myogenic in the sinus venosus and then runs forward. Flaps between the chambers prevent it from flowing back (see also blood circulation in fish ).
A modification of this blueprint is available for the lungfish (Dipnoi). The forecourt is divided here. While the right atrium, like the atrium of the other fish, absorbs the oxygen-poor blood from the body, the left atrium is supplied with oxygen-rich blood by the newly developed pulmonary circulation. A long spiral fold in the bulb cordis helps to channel the oxygen-rich blood into the body via the aorta dorsalis. Like the Elasmobranchii, the lungfish also have a stiff pericardium.
Amphibians
The heart of the amphibian is similar to that of the lung fish: It has two separate atria and a main chamber (ventricle). The sinus venosus is reduced in size in amphibians. Oxygenated blood from the lungs arrives in the left atrium, the blood from the circulatory system in the right. From there, the blood enters the chamber and then through the conus arteriosus into the pulmonary and body circulation. Trabeculae in the chamber allow oxygen-rich and oxygen-poor blood to be kept largely separate. A spiral fold in the conus arteriosus leads preferentially oxygen-poor blood to the arteria pulmocutanea, whose further branches lead to the lungs and the skin. In amphibians, skin breathing can provide an important part of the oxygen supply. The body's circulation, on the other hand, is supplied with oxygen-rich blood.
In contrast to the blood from the lungs, oxygen-rich blood from the skin arrives in the right atrium, together with the oxygen-poor blood from the body's circulation. When a frog is immersed in oxygen-rich water, less blood flows through the lungs and more through the skin. Even in this situation, the common ventricle of both circuits allows oxygen to be directed into the tissue (see also amphibian blood circulation ).
Reptiles
There are two different types of heart in reptiles. The heart type of the Crocodylia on the one hand and that of all other reptiles on the other.
The other reptiles, like the amphibians, have a heart with two separate atria and a common ventricle. The ventricle is divided into three interconnected spaces ( Cavum arteriosum , Cavum venosum and Cavum pulmonale ) by muscle ridges , so that a total of five chambers (better: heart cavities ) are spoken of. The sinus venosus, in the amphibians and fish upstream of the atrium, is further reduced and sometimes completely absent. Correspondingly, the stimulating tissue ( sinus node ) is shifted into the wall of the atrium, near the vein confluence.
While the blood from the ventricle in the amphibians still flows into a common conus arteriosus, in the reptiles this is divided into three parts, the pulmonary artery and the right and left aorta. In contrast to amphibians, skin respiration no longer plays a role, so that oxygen is only enriched in the lungs. Despite a common chamber, oxygen-rich and oxygen-poor blood usually remain separate. Oxygen-poor blood comes from the body's circulation into the right atrium, from there into the cavum venosum and further into the cavum pulmonale (blue arrows in the right figure). The oxygen-rich blood flows through the cavum arteriosum and cavum venosum into the two aortas and thus into the body's circulation.
The common ventricle enables the reptiles to bypass the pulmonary or body circulation with a shunt (German: branch, secondary connection) if necessary. The regulation of these processes is not yet fully understood, presumably it differs from species to species. A right-left shunt causes the pulmonary circulation to be bypassed and blood from the circulatory system in the heart to be returned to the circulatory system. This takes place in pauses in breathing that are common in reptiles. They also occur in reptiles that rest under water.
In the Crocodilia, the ventricle is completely divided, so that, as in mammals and birds, there is a four- chamber heart (better: a heart with four cavities , a four-cavity heart, from Latin cavum = cave, as a generic term for atrium and chamber). In contrast to these, however, the pulmonary circulation and the body circulation are not completely separate, so blood can move between the two. Oxygenated blood comes from the lungs to the left atrium and left ventricle. This is where the right aorta arises, which supplies the front body with the brain. Oxygen-depleted blood enters the right atrium and on to the right ventricle via the body vein. This is where both the left aorta, which supplies the abdomen, and the pulmonary artery arise. When breathing air and exercising, the pressure in the left ventricle is higher than that in the right. As a result, the pressure in the left aorta is higher than in the right. Through two connections between the left and right aorta, oxygen-rich blood also flows into the back of the body. The oxygen-poor blood, on the other hand, largely ends up in the lungs.
Those reptiles in which the separation of the ventricle into two halves is particularly pronounced achieve higher metabolic rates than others. In monitor lizards with their additional muscle bar (see illustration), it can amount to 20 milliliters of oxygen per minute per kilogram of body weight, while turtles only reach 10 milliliters. It is concluded that the development of completely separate chambers was important for the high metabolic rates of mammals and birds.
Mammals and birds
In mammals and birds , the left and right halves of the heart are completely separated from each other. In contrast to the Crocodilia, however, the lungs and body circulation are also completely separate, so that different pressures can be built up in both. Mixing of oxygen-poor and oxygen-rich blood is also excluded. Both halves of the heart have a thin-walled atrium and a thick-walled ventricle, so that there are a total of four heart cavities. Compared to other vertebrates, these have rather smooth inner walls.
The right half of the heart pumps the blood through the pulmonary circulation ("small circulation"), after which it arrives in the left atrium, rich in oxygen. The left half of the heart transports the blood through the body's circulation (“great circulation”), at the end of which it lands again in the right atrium. Since the total vascular resistance , as well as the blood pressure in the systemic circulation are considerably larger than in the pulmonary circulation, should the left ventricle a correspondingly larger work ( cardiac work ) against this resistance do, and therefore has a much greater wall thickness than the right to. Even with different filling volumes of the four heart cavities, the stroke volumes in both heart chambers and in both atria must be the same with each heartbeat. This equality is ensured, if necessary, by different ejection fractions. Heart valves between the atria and the ventricles and at the exit of the ventricles prevent blood from flowing back (see also blood circulation in vertebrates at the same temperature ).
The heart of the mammals differs little between the different species, apart from a size adjustment. In turn, individual heart muscle cells of the various species have hardly any differences in morphology and size. The heart mass increases linearly with the body mass in mammals, it is usually 0.6% of the body mass. The stroke volume increases accordingly. The blue whale has the largest heart, with a body weight of 100 tons, a heart weight of 600 kg and a stroke volume of 350 liters. His heart rate at rest is 6 beats per minute and can drop to 2 to 3 beats per minute during a dive. The Etruscan shrew probably has the smallest mammalian heart . With a body weight of 2 g, the heart weight is 12 mg and the stroke volume is 1.2 µl. The resting heart rate of 800 to 1200 beats per minute (= 13 to 20 beats per second) can be increased to 1500 beats per minute (25 beats per second) during physical exertion.
On the other hand, the blood pressure built up by the left ventricle in the body's circulation changes only slightly; it is between 100 and 150 mmHg systolic and between 70 and 105 mmHg diastolic, regardless of body size (see table).
In mammals, too, there is an allometric relationship between heart rate and body mass. The exponent b here is −0.25, similar to that of birds, so the heart rate decreases with increasing body mass. This is explained by the metabolic rate, which also decreases allometrically with increasing body mass (b = 3/4). The time interval from the excitation of the atria to the excitation of the ventricles ( PQ interval , see below) also increases allometrically with b = 1/4.
An exception in several respects is the giraffe, which requires higher blood pressure due to the height difference of around two meters between the heart and the brain. Depending on the source, this is 300/230 or 280/180 mmHg, making it the highest of all mammals. To build up the high pressure, the heart rate is 170 beats per minute, which is also very unusual for animals of this size (see also: Giraffe # Cardiovascular System .)
Due to the similarity of the hearts of the different mammalian species, the human heart shown below can be used as a model for all mammalian hearts.
Location and structure of the human heart
Some key figures of the human heart (averages) | |
---|---|
length | 15 cm |
Weight | 300 g |
Stroke volume | 70 cm³ per stroke |
Cardiac output (CO) at rest | 4.9 liters / minute |
CO with great effort | 20-25 liters / minute |
Work ( heart work ) | 0.8 joules per beat (left chamber) 0.16 joules per beat (right chamber) 100,000 joules per day (total) |
location
The heart lies within the heart sac (pericardium) in the mediastinum : the left and right lungs border the heart laterally, separated by the parietal and visceral pleura ( pleura ) . At the bottom the heart sits on the diaphragm , which is fused with the pericardium. Above, the windpipe (trachea) divides into the two main bronchi ( bifurcatio tracheae ), of which the left is crossed by the aortic arch . Below this division is the left atrium. If this is abnormally enlarged, this can lead to a spreading of the main bronchi, which is shown in the X-ray image as an enlarged angle between the bronchi. The left atrium is also in direct posterior contact with the esophagus . In front of the heart is the breastbone (sternum), in the upper area it is in front of the outgoing large vessels. The thymus lies between the sternum and the heart .
The heart is practically directly behind the anterior body wall at the level of the second to fifth rib . The base of the heart extends to the right about two centimeters beyond the right edge of the breastbone. At the bottom, the apex of the heart comes close to an imaginary vertical line that runs through the middle of the left collarbone (left medioclavicular line ).
anatomy
The shape of the heart resembles a rounded cone , the tip of which points downwards and slightly to the left in front. The heart volume roughly corresponds to the volume of the person's closed fist. In humans, the heart is usually slightly offset to the left behind the breastbone . In rare cases it is shifted to the right (the so-called dextrocardia - "right- heartedness "), mostly with situs inversus ( i.e. with mirror-inverted organ arrangement).
A healthy heart weighs around 0.5% of body weight, between 280 and 340 grams for men and between 230 and 280 grams for women. The heart mass increases continuously over the majority of life, whereby with sustained stress it reacts more with the (low-risk) enlargement of already existing heart muscle cells: from around 500 g, the so-called critical heart weight , the risk of insufficient supply of the now enlarged heart increases with oxygen, as the coronary arteries supplying it do not grow to the same extent.
Contrary to previous assumptions, humans form new heart muscle cells in the course of their lives, but only to a limited extent. At the age of 25, the annual regeneration is around one percent, by the age of 75 it drops to below 0.5 percent. This means that less than 50% of the heart muscle cells are replaced during an average life span.
Wall layers
The heart is full of connective tissue pericardium ( fibrous pericardium, pericardium enclosed). The lower side of the pericardium is connected to the diaphragm ( diaphragm fused), so that the movements of the diaphragm during respiration on the heart are transmitted. The innermost layer of the pericardium ( pericardium serosum ) turns into the epicardium at the exit of the large blood vessels (see below) , which lies directly on the heart. Between the pericardium and the epicardium there is a capillary gap filled with 10–20 ml of fluid , which enables the heart to move with little friction in the pericardium .
These complicated relationships become clearer if one imagines the pericardium as a balloon that is filled with air and closed. Your own hand, closed into a fist, represents the heart. If you press the balloon in with your fist so far that it is completely enclosed by the balloon, a layer of the balloon lies directly on the fist (the "heart"). This layer, which corresponds to the epicardium, turns into an outer layer at the transition to the arm. This outer layer corresponds to the pericardium. Between the two there is an air-filled space, which is comparable to the fluid-filled gap in the pericardium.
Under the epicardium there is a layer of fat ( Tela subepicardiaca ) in which the coronary vessels run. The thick muscle layer ( myocardium ) of specialized muscle tissue that only occurs in the heart follows inward . The interior of the heart is lined by the endocardium , which also forms the heart valves .
Spaces and vessels of the heart
The right and left halves of the heart each consist of a chamber ( Latin: ventriculus cordis , (heart) ventricle, RV and LV for short) and an atrium ( atrium , RA and LA). These spaces are separated by the heart septum (septum). This is divided into the atrial septum ( septum interatriale , atrial septum ) and the ventricular septum ( septum interventriculare , ventricular septum ).
The blood can only flow in one direction between the heart chambers, since heart valves that work like non-return valves are located between the atria and the chambers and between the chambers and the adjoining vessels . All four valves of the heart are roughly in one plane, the valve plane , and are suspended together on a connective tissue plate , the cardiac skeleton . Within the chambers and atria there are muscles that protrude into the cavities - the papillary muscles and the pectinate muscles .
Arteries carry blood from the heart to the organs, veins from the organs to the heart. Arteries in the circulatory system carry oxygen-rich (arterial) blood, while arteries in the pulmonary circulation carry oxygen- poor (venous) blood. Conversely, the blood in the veins of the body's circulation is low in oxygen (venous) and that in the pulmonary veins is rich in oxygen (arterial).
The superior and inferior vena cava ( superior and inferior vena cava ) open into the right atrium . They lead the oxygen-poor blood from the great circulation (body circulation) to the heart. The tricuspid valve is located between the right atrium and the right ventricle , which prevents the blood from flowing back into the atrium when the ventricle contracts. From the right ventricle of the heart, the blood flows through a common trunk ( Truncus pulmonalis ) into the two pulmonary arteries . The backflow into the right chamber is prevented by the pocket-shaped pulmonary valve . The pulmonary arteries supply the oxygen-poor blood to the pulmonary circulation (small circulation).
The oxygen-enriched blood in the lungs flows through usually four pulmonary veins into the left atrium. From here it reaches the left ventricle via another leaflet valve , the mitral valve . The outflow occurs through the so-called left ventricular outflow tract ( LVOT ) via another pocket valve ( aortic valve ) and the main artery ( aorta ) into the body circulation.
Coronary arteries

From the initial part of the aorta , the right and left spring coronary artery ( coronary arteries ). They supply the heart muscle with blood itself. The coronary arteries are so-called "functional end arteries ". This means that a single artery is connected to other arteries ( anastomoses ), but that these connections are too weak to ensure blood flow to the tissue in another way if there is insufficient supply. So if an artery fails due to a blockage or other disruption, tissue death occurs in the area supplied by this artery.
The left coronary artery ( Arteria coronaria sinistra , left coronary artery , LCA) supplies the front of the heart. It divides into a ramus interventricularis anterior (RIVA, left anterior descending , LAD) and a ramus circumflexus (RCX).
The right coronary artery ( Arteria coronaria dextra , right coronary artery , RCA) gives off the A. marginalis dextra , which supplies the free wall of the right ventricle. At the "heart cross" ( crux cordis ) it divides into the ramus interventricularis posterior and the ramus posterolateralis dexter . The right coronary artery also supplies an important part of the excitation system ( sinus node , atrioventricular node ).
There are three large coronary veins that open into the coronary sinus of the right atrium and drain the deoxygenated blood from the heart muscle. The great cardiac vein ( V. cordis magna ) runs on the front, the middle cardiac vein ( V. cordis media ) on the back and the V. cordis parva on the right edge of the heart. A small part of the deoxygenated blood is emptied directly into the ventricles via the Thebesius veins.
development
In humans, the heart begins to form as early as the 3rd week of embryonic development . For this purpose, angioblasts (blood vessel formation cells ) are deposited in front of and to the side of the prechordal plate - the beginning of vascular development ( vasculogenesis ). At first they form several smaller cavities (sinuses), which finally merge to form the horseshoe-shaped heart tube. The appendix then moves caudoventrally (downwards and towards the abdomen). Embryonic connective tissue ( mesenchyme ) from the splanchopleura (side plates mesoderm ), which forms the heart muscles ( myocardium ) , is located around the heart tube . The epicardium - the thin covering of the heart - is made up of mesothelial cells .
The primitive heart tube consists of the following parts:
- Truncus arteriosus
- Bulbus cordis primitivus
- Ventriculus primitivus
- Atrium primitivum
- Sinus venosus
On the 23rd or 24th day, the heart begins with peristaltic contractions and thus with pumping movements.
A distinction is made between an inflow and an outflow path in the fetal heart. The yolk sac veins ( Vv. Vitellinae ), which carry the blood from the yolk sac into the embryonic circulation, flow into the sinus venosus , the umbilical vein ( V. umbilicalis ), which carries oxygen-rich blood from the chorionic villi , and the cardinal veins ( Vv. Cardinales anteriores et posteriores ). which contain the blood from the actual embryonic circulation and bring it back again. The outflow path is first connected to the branchial arch arteries , later to the aortic arch or the pulmonary trunk .
Important processes in the context of development are the formation of the cor sigmoideum (from the tube to the loop) and the separation into two separate circuits (body and lung circulation). The atrium primitivum is further divided into a right and a left atrium (through the outgrowth of endocardial pillows ) and the ventriculus primitivus into a right and a left heart chamber (through the formation of the muscular and membranous septum).
The leaflet valves (between atria and ventricles) are also formed from outgrowing endocardial cushions, the pocket valves through the formation of endothelial bulges .
How the human heart works
Excitation formation and excitation conduction system
The contraction of heart muscle fibers is triggered by electrical signals. In a myogenic heart like the human one, the impulses necessary for the heart's action are generated spontaneously and rhythmically in specialized heart muscle cells, the pacemaker cells. So that the electrical excitation can spread through the heart, the individual heart muscle cells are connected to one another via small pores in their cell membranes . Ions flow from cell to cell via these gap junctions . The excitation originates in the sinus node between the superior vena cava and the right atrial appendage , first spreads through both atria and then reaches the chambers via the atrioventricular node (AV node) in the valve level.
In the two chambers of the heart there is an excitation conduction system for faster transmission, which consists of specialized heart muscle cells. Starting from the AV node, these cells form the bundle of His that pierces the heart skeleton and divides into a right and a left tawara branch for the right and left ventricles. The left tawara thigh divides into a left anterior and a left posterior bundle. The end section of the excitation conduction system is formed by Purkinje fibers that run to the apex of the heart, where they turn around and end directly under the endocardium in the working muscles. In some cases they can also pull through the clearing of the chamber as "false tendon threads" (Chordae tendineae spuriae) or within the moderator ligaments (Trabeculae septomarginales). This system enables the chambers to contract in a coordinated manner despite their size.
If, for whatever reason, the atrial excitation does not reach the AV node, it is itself a slower ventricular excitation (approx. 40 / min). The AV node also forms a frequency filter that blocks atrial excitation that is too fast (e.g. atrial flutter or fibrillation ) (→ AV block ).
Mechanics of the action of the heart
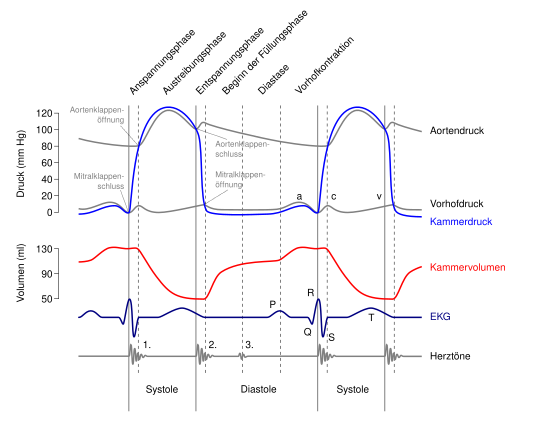
At rest, the human heart pumps around the entire volume of blood in the body once a minute through the circulation, which is around five liters per minute in adults. During physical exertion, the pumping power can be increased by about five times, with the oxygen demand increasing accordingly. This increase is achieved by doubling the stroke volume and increasing the heart rate by a factor of 2.5.
With each pumping action, each chamber and each atrium conveys a little more than half of the maximum filling volume, i.e. around 50-100 ml of blood. This is the ejection fraction , i.e. the percentage of the end-diastolic filling volume that is thrown out of the heart cavity. The heart rate (beats / minute) is 50–80 / min at rest (over 120–160 in newborns) and can rise to over 200 / min under exertion. If the heartbeat is too slow (less than 60 / min at rest), it is called bradycardia . If the heart beats too fast (in adults over 100 / min at rest), one speaks of a tachycardia .
During a cardiac cycle, the atria initially fill while the ventricles expel blood into the arteries. When the ventricular muscles relax, the leaflet valves open and the blood flows from the atria into the ventricles, sucked by the pressure drop in the ventricles. This is supported by a contraction of the atria (atrial systole). The systole chamber follows . The ventricular muscles contract, the pressure rises, the leaflet valves close and the blood can only flow into the arteries through the now open pocket valves. A backflow of blood from the arteries during the relaxation phase ( diastole ) is prevented by closing the pocket flaps. The direction of flow is determined solely by the flaps.

In addition to the muscles, which are by far the largest part of the tissue in the heart, the heart has what is known as a cardiac skeleton . It is a connective tissue structure, which mainly consists of the “bezels” of the valves. The heart skeleton has three important functions: It serves as an attachment point for the muscles, as an attachment point for the heart valves (hence also known as the valve level) and for the electrical separation of the atrial and ventricular muscles in order to prevent simultaneous contraction.
The heart skeleton is decisive for the mechanics of the heart's action: Due to the recoil during the expulsion of blood, the apex of the heart is relatively fixed during the entire cardiac cycle and hardly moves. Consequently, when the ventricular muscles contract (systole), the valve plane is pulled downwards in the direction of the apex of the heart. In the relaxation phase of the ventricular muscles (diastole), the valve level moves again towards the heart base.
When the valve level is lowered, on the one hand the blood is ejected from the chamber into the circulation and, on the other hand, the associated atrium is enlarged. A negative pressure occurs, which causes blood to flow from the large veins into the atria. When the ventricular musculature slackens, the valve level rises, whereby the ventricles are passively expanded over the blood columns of the atria and fill up to about 70-80%. The subsequent contraction of the atria pumps the remaining blood into the chambers and thus initiates a new cardiac cycle. The atrial contraction is therefore not absolutely necessary for the functioning of the heart, which is also shown by the fact that (in contrast to ventricular fibrillation ) patients with atrial fibrillation are quite viable.
A reduced pressure in the right atrium measured with a pulmonary catheter, for example, can indicate insufficient blood volume or sepsis , while an increased pressure indicates a cardiogenic shock , a right ventricular myocardial infarction , a pulmonary embolism or a pericardial tamponade .
regulation
During physical exertion, the cardiac output is increased by the action of sympathetic nerve fibers, which release the transmitter norepinephrine on the cells of the work muscles and the excitation conduction system. In addition, noradrenaline, together with adrenaline, reaches the heart as a hormone via the bloodstream. The effects of noradrenaline and adrenaline are mainly mediated via β 1 -adrenoceptors . This is G-protein-coupled and activates an adenylate cyclase (AC), which catalyzes the synthesis of cAMP from ATP . A cAMP-dependent protein kinase (PKA) then phosphorylates calcium channels and thereby increases the slow influx of calcium into the muscle cell. This leads to the release of more calcium from the sarcoplasmic reticulum (SR) and thus to increased muscle contraction of the heart during systole (positive inotropic effect). In addition, the PKA phosphorylates the phospholamban of the SR, which increases calcium uptake in the SR and shortens the relaxation time of the heart in diastole (positive lusitroper effect). In addition, the heart rate ( positive chronotropic ) and the conduction speed in the AV node ( positive dromotropic ) increase.
The antagonist of the sympathetic nervous system is also the parasympathetic nervous system at the heart , which acts via the vagus nerve (Xth cranial nerve ), which with the acetylcholine transmitter reduces the heart rate , the contraction force of the heart, the conduction speed of the AV node and the excitability of the heart ( negative chronotropic, negative inotropic, negative dromotropic and negative bathmotropic ), whereby the effect of the parasympathetic on ino- and bathmotropy is rather small.
At the same time, the force of contraction ( heart force ) automatically adapts to the requirements: If the heart muscle is stretched more by additional blood volume, the function of the contractile elements in the muscle cells improves ( Frank-Starling mechanism ). This mechanism makes a significant contribution to the fact that the simultaneous stroke volumes of the right and left ventricles do not differ: If for any reason the stroke volume of one half of the heart increases for a short period of time, this leads to an increase in the filling volume of the other half of the heart during the subsequent heart action. This will stretch the wall more and the chamber can also eject a larger volume of blood with improved contraction force. With every cardiac action, the product of the end-diastolic filling volume and the associated net ejection fraction is necessarily constant in all four heart cavities . If the four stroke volumes were not identical, blood would congest immediately.
In its atria (especially in the right atrium), the heart produces a diuretic hormone, the atrial natriuretic peptide (ANP), depending on the stretch , in order to influence the circulating blood volume.
Diseases
In medicine , cardiology as a specialty of internal medicine deals with the heart and the conservative treatment of heart disease in adults; Heart surgeries are performed by cardiac surgeons . Heart diseases in children, as far as they can be treated conservatively, are the subject of pediatric cardiology, which has developed as a branch of pediatrics since around 1975. Surgical therapy for children is, at least in Germany, taken over by the pediatric cardiac surgery established as a specialization . Since around 1995 more and more children with complex congenital heart defects have reached adulthood, the question of medical care for this group of patients arises today, who are dependent on cardiological check- ups for life and who may also have to be re-operated. Only a few adult cardiologists have received intensive training in the field of congenital heart defects. Pediatric cardiologists are very competent in the field of various clinical pictures, but as pediatricians they are not trained in adult cardiology. Therefore, interdisciplinary consultation hours are increasingly offered in various heart centers.
See also
literature
- Ole Martin Høystad: Cultural History of the Heart. From antiquity to the present. Translated from the Norwegian by Frank Zuber. Böhlau, Cologne / Weimar / Vienna 2006, ISBN 3-412-28705-9
- Charles Reginald Schiller Harris: The heart and the vascular system in ancient Greek medicine from Alcmaeon to Galen. Oxford 1973.
- Friedrich Wilhelm Hehrlein : heart and large vessels. In: Franz X. Sailer, FW Gierhake (Hrsg.): Surgery seen historically: beginning - development - differentiation. Dustri-Verlag, Deisenhofen near Munich 1973, ISBN 3-87185-021-7 , pp. 164-185.
- Das Herz (memorial for Ernst Boehringer), edited by the Dr. Karl Thomae GmbH, 3 volumes, Biberach an der Riss 1965–1969.
- Susanne Hahn: Heart . In: Werner E. Gerabek , Bernhard D. Haage, Gundolf Keil , Wolfgang Wegner (eds.): Enzyklopädie Medizingeschichte. De Gruyter , Berlin / New York 2005, ISBN 3-11-015714-4 , p. 581 ff.
- Herbert Reindell , Helmut Klepzig: diseases of the heart and blood vessels. In: Ludwig Heilmeyer (ed.): Textbook of internal medicine. Springer-Verlag, Berlin / Göttingen / Heidelberg 1955; 2nd edition, ibid. 1961, pp. 450-598.
Web links
- Introduction to Cardiothoracic Imaging
- HM Sommer: Heart ( Memento from June 11, 2007 in the Internet Archive ; PDF; 2.7 MB)
Animations
- 3-dim. Animation of the beating heart with lots of information
- 2-dim. Animation of the beating heart with diagrams (English)
Institutions
- dgk-herzfuehrer.de - German Society for Cardiology
- German Society for Thoracic, Cardiac and Vascular Surgery
Individual evidence
- ^ The dictionary of origin (= Der Duden in twelve volumes . Volume 7 ). Reprint of the 2nd edition. Dudenverlag, Mannheim 1997 ( p. 283 ). See also heart. In: Digital dictionary of the German language . Retrieved on August 30, 2019 and Friedrich Kluge : Etymological Dictionary of the German Language . 7th edition. Trübner, Strasbourg 1910 ( p. 206 ).
- ↑ Jutta Kollesch , Diethard Nickel : Ancient healing art. Selected texts from the medical writings of the Greeks and Romans. Philipp Reclam jun., Leipzig 1979 (= Reclams Universal Library. Volume 771); 6th edition ibid 1989, ISBN 3-379-00411-1 , p. 23.
- ↑ Jutta Kollesch, Diethard Nickel: Ancient healing art. [...]. 1989, p. 23.
- ^ Christopher D. Moyes, Patricia M. Schulte: Tierphysiologie . Pearson Studium, Munich 2008, ISBN 978-3-8273-7270-3 , pp. 6–7 ( limited preview in Google Book Search - English: Principles of Animal Physiology . Translated by Monika Niehaus , Sebastian Vogel).
- ↑ a b c d e f g h Heinz Penzlin : Textbook of animal physiology . 7th edition. Spektrum Akademischer Verlag, Heidelberg 2009, ISBN 978-3-8274-2114-2 , p. 339-351 .
- ↑ a b c d e f g h i j Adolf Remane, Volker Storch, Ulrich Welsch: Short textbook of zoology . 5th edition. Gustav Fischer Verlag, Stuttgart 1985, ISBN 3-437-20337-1 , p. 188 f .
- ↑ a b c d e f g h i j k l m n o p q Christopher D. Moyes, Patricia M. Schulte: Tierphysiologie . Pearson Studium, Munich 2008, ISBN 978-3-8273-7270-3 , pp. 379–394 ( limited preview in the Google book search - English: Principles of Animal Physiology . Translated by Monika Niehaus , Sebastian Vogel).
- ^ JD Glenn, JG King, JF Hillyer: Structural mechanics of the mosquito heart and its function in bidirectional hemolymph transport. In: Journal of Experimental Biology. 213, 2010, p. 541, doi : 10.1242 / jeb.035014 .
- ↑ a b c d e f g h Heinz Penzlin : Textbook of animal physiology . 7th edition. Spektrum Akademischer Verlag, Heidelberg 2009, ISBN 978-3-8274-2114-2 , p. 314-326 .
- ↑ a b G. P. Dobson: On being the right size: heart design, mitochondrial efficiency and lifespan potential. In: Clinical and experimental pharmacology & physiology. Volume 30, Number 8, August 2003, pp. 590-597, ISSN 0305-1870 . PMID 12890185 .
- ↑ a b c Heinz Penzlin : Textbook of animal physiology . 7th edition. Spektrum Akademischer Verlag, Heidelberg 2009, ISBN 978-3-8274-2114-2 , p. 331 .
- ↑ a b Maike Prütz et al .: The anatomy of the heart of the tortoise (Testudinidae). In: Small Animal Practice. Volume 61, Issue 4, 2016, pp. 185–194.
- ↑ FL Meijler, TD Meijler: Archetype, adaptation and the mammalian heart. In: Netherlands heart journal: monthly journal of the Netherlands Society of Cardiology and the Netherlands Heart Foundation. Volume 19, Number 3, March 2011, pp. 142-148, ISSN 1876-6250 . doi: 10.1007 / s12471-011-0086-4 . PMID 21475406 . PMC 3047700 (free full text).
- ^ GB West, JH Brown: The origin of allometric scaling laws in biology from genomes to ecosystems: towards a quantitative unifying theory of biological structure and organization. In: The Journal of experimental biology. Volume 208, Pt 9 May 2005, pp. 1575-1592, ISSN 0022-0949 . doi: 10.1242 / jeb.01589 . PMID 15855389 . (Review).
- ↑ SF Noujaim, E. Lucca, V. Muñoz, D. Persaud, O. Berenfeld, FL Meijler, J. Jalife: From mouse to whale: a universal scaling relation for the PR Interval of the electrocardiogram of mammals. In: Circulation. Volume 110, Number 18, November 2004, pp. 2802-2808, ISSN 1524-4539 . doi: 10.1161 / 01.CIR.0000146785.15995.67 . PMID 15505092 .
- ^ Theodor Heinrich Schiebler, Walter Schmidt: Textbook of the entire human anatomy. Cytology, histology, history of development, macroscopic and microscopic anatomy. 3rd, corrected edition. Springer, Berlin a. a., ISBN 3-540-12400-4 , p. 440.
- ^ E. Strauzenberg: Short outline of the problem of competition at altitude above 2000 m. In: The Journal of sports medicine and physical fitness. Volume 16, Number 4, December 1976, pp. 346-347, ISSN 0022-4707 . PMID 1011819 .
- ^ O. Bergmann, RD Bhardwaj, S. Bernard, S. Zdunek, F. Barnabé-Heider, S. Walsh, J. Zupicich, K. Alkass, BA Buchholz, H. Druid, S. Jovinge, J. Frisén: Evidence for cardiomyocyte renewal in humans. In: Science. Volume 324, Number 5923, April 2009, pp. 98-102, ISSN 1095-9203 . doi: 10.1126 / science.1164680 . PMID 19342590 . PMC 2991140 (free full text).
- ↑ Amitava Majumder, Anne Paschen: Medical working techniques. In: Jörg Braun, Roland Preuss (Ed.): Clinic Guide Intensive Care Medicine. 9th edition. Elsevier, Munich 2016, ISBN 978-3-437-23763-8 , pp. 29-93, here: pp. 39 f. ( Pulmonary catheter ).
- ↑ Erland Erdmann : Clinical Cardiology . 7th edition. Springer publishing house