arthropod
arthropod | ||||||||||||
---|---|---|---|---|---|---|---|---|---|---|---|---|
![]() Examples of fossil and recent arthropod groups: trilobites , jaw-claw carriers such as sea scorpions and arachnids , crustaceans , millipedes and hexipedes |
||||||||||||
Systematics | ||||||||||||
|
||||||||||||
Scientific name | ||||||||||||
Arthropoda | ||||||||||||
von Siebold , 1848 | ||||||||||||
Sub-tribes | ||||||||||||
|
The arthropods ( Arthropoda , from ancient Greek ἄρθρον arthron , German 'limb, joint' and πούς pous , Gen. ποδός podos , German 'foot' ) are a tribe of the animal kingdom . They include insects , millipedes , crustaceans (e.g. crabs , barnacles ), arachnids (e.g. spiders, scorpions , mites ) and the extinct trilobites .
Arthropods are a very successful tribe. Around 80 percent of all known recent (non- fossil ) animal species are arthropods. They are distributed among almost a million insect species, just under 10,000 other six-pods , around 16,000 millipedes, a good 100,000 arachnids and just under 50,000 crustaceans (only orders of magnitude, exact numbers vary depending on the author and the year of publication). They were probably formed during the Cambrian Explosion , when the multicellular animal phyla suddenly appeared in the fossil record at the beginning of the Cambrian , around 540 million years ago.
The closest relatives of the arthropods are the tardigrade (Tardigrada) and columbus (Onychophora), with which they are grouped together to form the Panarthropoda . Some taxonomists call this superordinate group Arthropoda, the "actual" arthropods are then called " Euarthropoda ".
In the traditional system, this group is combined with the annelid worms to form the articulata for morphological reasons . More recent molecular data speak but for a close relationship with the arthropod nematodes (Nematoda) and their closest relatives ( Cycloneuralia ). This common grouping is called molting animals (Ecdysozoa).
anatomy

1 epicuticle with details ( B ):
1a cementum
1b wax layer
1c epicuticle externa
1d epicuticle interna
2 exocuticle, 3 endocuticle
2 + 3 Procuticula
4 epithelial 5 basement membrane
6 epithelial cell, 6a pore channels
7 gland cell
8 Cuticulagrube (Bothrion)
9 Wärmerezeptive Cells
10 nerve endings
11 trichobothrium
12 sensillum
13 glandular openings
Exoskeleton
The body shell of arthropods forms an outer skeleton that of the epithelium cells of the uppermost cell layer ( epidermis is) deposited outward; it is called the cuticle. Due to the firm outer shell, growth is only possible because this shell is periodically stripped off and replaced ( molting ). The hardened outer shell of the body and extremities is the characteristic that best characterizes the arthropods; similar formations only occur in the related tardigrade .
The body shell consists of chitin and numerous proteins. Chitin and proteins are firmly connected to one another in a network-like manner (" sclerotized ") by embedded compounds derived from dopamine . It can then remain flexible (e.g. on the abdomen of most spiders or numerous insect larvae). Typically, however, hardened, more heavily sclerotized plates ( sclerites ) are embedded, which are interrupted by less sclerotized joint membranes, but can occasionally be firmly connected to form sheaths or armor. In many crustaceans and millipedes and a few insects (larvae), the shell is reinforced by calcium deposits.
The shell is colorless, straw-colored or yellowish, often colored by melanin , pigments , and occasionally also dark or brightly colored by structural colors. In the case of insects and spiders, the outer layer has a lipid layer as protection against evaporation, which millipedes and all terrestrial crustaceans lack.
Segments
Arthropods have a body that is divided into segments . In the basic construction plan, the segments have the same structure with the same organs, attachments, etc. (with the exception of the first and the last). In all arthropods, this basic construction plan is only partially implemented through numerous modifications, but as a rule the segments are clearly demarcated from one another, and numerous organs (e.g. limbs, openings of the heart, glands, muscles) are segmented.
It is traditionally assumed that in the basic construction plan the arthropods had an indefinite number of similar segments, the number of which was only fixed later in the course of specialization. But this assumption is largely based on the analogy with the annelid worms . All arthropods with the exception of a few millipedes, the gill pods and the extinct trilobites have a fixed segment number; The Lobopods , which have been handed down to fossils from the Cambrian and are generally regarded as representatives of the core groups, also had a fixed (and manageable large) number of segments. Within research it is still disputed whether the segmentation is an original characteristic which the arthropods inherited from the common ancestor of the Bilateria , or whether it is an evolutionary novelty; in this case, the segmentation would have arisen convergently in the other animal phyla segmented in the basic building plan (especially the annelid worms and the chordates ) .
A pair of limbs ( legs , mouthparts , antennas ), which are also divided into sections , can sit on each segment, but this can also be missing on different segments. The developmental basis of body segments and leg sections is comparable, the extremities can be understood as secondary body axes.
The nervous system is also segmental and consists of a double strand running on the abdominal side with a ganglion and a cross connection (commissure) per segment (" rope ladder nervous system "). Typical is also a back-side ( dorsal ) heart and an open circulatory system.
The original structure of a segment consists of the trunk part with a ganglion and a pair of two-branched (biram) limbs ( split bones ). The (lower) inner branch is called leg or striding leg , the outer branch is called the gill branch , which also indicates the functions.
Merging of several segments (Tagmatization)
With the diversification of the arthropods in the course of evolution , groups were formed or segments were merged . Which segments are fused together to form a tagma is different for different groups. In all arthropods, some of the anterior segments have merged to form the head, which has a brain that has grown together from several ganglia and one- or two-branched limbs formed into mouthparts and antennae. The different groups of arthropods have a different number of segments fused to form the head.
In primitive arthropods like the Remipedia , the structure of the body behind the head still largely consists of almost identical segments. The trilobites had a similarly repetitive physique.
In the case of the millipedes , ancestors with relatively few segments (12 to 15 depending on the stem group) were secondary animals with numerous similar segments; In the double-footed ( Diplopoda ) two successive segments are fused together to form a double segment, so that each “segment” of the trunk has two pairs of legs; many centipedes have a wide and a narrow segment following each other.
Tagmatization is more advanced in the case of insects : several segments are fused to form the head, middle and abdomen. The three body sections that are created in this way have very different functions. The limbs of the original segments of the head are transformed into antennae and mouthparts. In the middle body ( thorax ) three segments have grown together to form a tagma, whose three pairs of limbs have lost the outer branch and serve as striding legs. Such limbs are called uniram . In the abdomen, the limbs can be completely regressed or transformed into special organs (gills, mating organs, ovules), but they never serve as legs for locomotion. In arthropods that do not have any visible gills, breathing takes place either via gills ( lungs ) that are turned into the surface of the body or via branched-out invaginations of the body wall, the trachea . The abdomen can consist of different numbers of segments without limbs. The insect wings are traditionally not interpreted as limbs, but as transformed side plates of the breast segments. However, recent genetic and developmental findings suggest that they go back to the branch of the gill of the original split bone. (In this case, the gills of aquatic insect larvae, e.g. the mayflies , are homologous formations. Fossil insect larvae exist from the Mesozoic, which can be interpreted as transitional links.)
In the other sub-tribes, the segments have been merged in other variants. Spiders consist of two sections. The front abdomen ( prosoma ) consists of six fused segments. One of the pairs of legs was transformed into mouth parts, the chelicerae , one into feelers and four into legs. The limbs of the abdomen ( opisthosoma ) have lost the leg branch, the branch of the gill lies in the form of a fan lung in invaginations of the abdomen; in some species these fan lungs have been transformed into tracheas. The spinnerets arose from the last pairs of legs . The front and rear bodies of the harvestmen and sham scorpions are fused into one compact body.
The horseshoe crabs (Xiphosura) are on the abdomen or legs get (leaf legs), their gills branches form compartments gills, which are very similar to the subjects lungs of spiders.
In the case of the crustaceans , the body is usually also divided into head, thorax and abdomen, but the blueprint has been modified in many ways. Many cancer orders have one or two original segments of the thorax functionally fused to the head. The thorax has a very different number of leg-bearing segments. A limbless abdomen is present or may be absent, depending on the kin group. In the Malacostraca , instead of the abdomen, a limb-bearing pleon is formed. At the end of their body stands a tail rudder fused from several appendages. Many other crabs here have long appendages (usually referred to as furca). In many crustaceans, the structure of the body can be covered by a body duplication, the carapace . This can be designed in one piece or with two shells to be movable relative to one another; in some groups ( Ostracoda , Conchostraca ) it envelops the whole body, making it look like a shell. In the Malacostraca, the carapace, which was originally only attached to the head, is firmly fused with the thorax and thus forms a uniform cephalothorax that is no longer mobile . For some fixed ( barnacles , barnacles ), but especially with parasitic groups of the physique can be completely redesigned so that the animal barely recognizable as arthropods is.
Sense organs
eyes
Arthropods have different numbers (up to nine) eyes on their heads with very different structures and different functions. The eyes of all arthropods have a blueprint that can be traced back to a few basic types whose endpoints, some of which are greatly modified, can be connected to one another by rows. It can therefore be assumed that the eyes of all arthropods represent homologous formations. The eyes can be divided into two types, each characterized by innervation, receptor and transmitter molecules, these are the side eyes and the middle eyes.

The outwardly curved side eyes are compound eyes that consist of numerous individual eyes (complex eyes). The individual eyes are called ommatidia . In the insects and crustaceans, the structure of the ommatidia is very uniform: They consist of a retina that contains eight light-sensitive sensory cells, above which lies a transparent crystal cone made up of four so-called Semper cells. To the side of this are two pigment cells that screen light from the side; these also separate the outer cornea . The cornea is thickened in the shape of a lens in most groups. In addition to these two so-called main pigment cells, there are numerous other secondary pigment cells. The structure of the ommatidia can be secondary modified or simplified in other groups of arthropods.
Complex eyes exist in most insects (they are absent from the other Hexapoda) and numerous crustaceans. With the chelicerates, complex eyes are only present in one group, with the horseshoe crabs . The only millipedes that have complex eyes are the arachnids (Scutigeromorpha). The ommatidia of these groups differ in their fine structure from those of the insects and crustaceans. In the horseshoe crab Limulus , each ommatidium consists e.g. B. from more than 300 cells.
In the groups in which they are present, the complex imaging eyes are usually the most important eyes. The visual cells of all retina cells of an ommatidium work together and form an image point. The resolution of the complex eye therefore depends on the number of ommatidia, which ranges from a few to a few hundred (e.g. around 700 in the fruit fly or 1000 in the horseshoe crab) to a maximum of 30,000 in some species of dragonfly. The spatial resolution of a complex eye never comes close to that of the highly developed lens eyes of vertebrates and cephalopods, but their temporal resolution is significantly better when seeing motion.
In addition to spatial vision, numerous arthropods can distinguish colors from all lines. As with humans, their color vision goes back to the possession of different visual pigments (as generally in the animal kingdom variants of opsin ), which respond at different wavelengths. Similarly to humans, most of them have three such pigments ( trichromatic vision). In contrast to humans, however, the sensitivity is often shifted to the short-wave part of the spectrum. Many species can therefore not see red, but a part in the ultraviolet. Another sensory quality that arthropods have ahead of humans is seeing the plane of polarization of polarized light. This enables them to see the position of the sun when the sky is overcast, for example, and makes it easier to discover bodies of water.
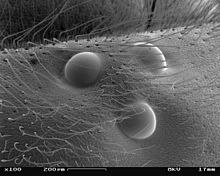
Almost all groups that have complex eyes also have other eyes that go back to the median eyes (exception: median eyes are absent in all millipedes). Other eye types also occur in the other arthropods in which the eyes are not completely regressed. In the case of insects, there are usually three point eyes ( ocelles ) on the forehead , the function of which has not been clarified in all cases, at least in some groups they are used for position information in flight. In many groups of crustaceans, three median eyes (only four in the phyllopods) form a central organ of vision, the nauplius eye. This is the most important sensory organ of the crab larvae, but is also present in numerous adults. The Malacostraca often have a pair of back (dorsal) and a pair of ventral (ventral) median eyes; in other groups there may be a different number. The real spiders, which never have complex eyes, only have a pair of median eyes and usually two or three pairs of side eyes. The side eyes are never used for visual vision, they are motion detectors in the wolf spiders , the jumping spiders and the crab spiders , in the other groups they are only used for spatial navigation according to the position of the sun, often by detecting polarized light.
In the species-rich group of arthropods, there are special adaptations in numerous groups, each of which enables special sensory performance. Jumping spiders , for example, have enlarged median eyes, which enable them to see images based on principles similar to those of the vertebrate lens eyes. Some groups (such as the copepod Labidocera or the larvae of the swimming beetle Thermonectes ) have developed eyes with a minimal field of view and high resolution, with which they scan their surroundings with a scanner movement like a reading head. Adjustments to low light conditions are particularly common. Many species that are active at twilight or that live in greater depths of the sea have redesigned their complex eyes in such a way that light can reach every receptor unit from a larger section of the environment (superposition eye). However, some groups, especially those living on the ground, have completely given up their eyes. This saves you a considerable amount of energy, because in addition to the eyes themselves, a considerable part of the brain in all arthropods with the ability to see images serves the optical resolution.
In addition to the eyes, numerous arthropods also have a skin or nerve light sense, which is completely independent of having eyes. This can e.g. They can be used, for example, to recognize enemies by casting shadows (e.g. in the crayfish Procambarus clarkii ) or to control the gonads by recognizing the length of the day, as in some butterfly species.
Mechanical senses
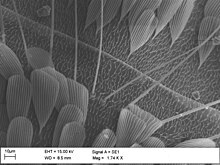
Arthropods mainly use two different ways to perceive mechanical stimuli, through sensory hair or bristles and through receptors that register the deformation of the cuticle (the exoskeleton). These organs are used to perceive the position of body parts in space (proprioceptors), for close-up orientation (sense of touch) and for distance orientation (sense of vibration, flow, hearing). Although numerous sensors can perform several of these tasks in parallel, there are sometimes highly specialized organs for special purposes.
The arthropods' sense of touch is largely based on simple sensory hair. In addition, the aerial insects and arachnids in particular have developed extremely thin sensory hairs that serve as flow and vibration sensors; these are called thread hairs in insects and trichobothria in arachnids . In contrast to normal hair, these are usually turned into a ring-shaped depression in the cuticle. In all arthropods, sensils are located directly in the cuticle , which can perceive both tactile stimuli and vibrations. The receptors ( scolopidia ) can develop special sensory organs, such as the cleft sensory organs of arachnids, which in real spiders are bundled in groups ( lyre-shaped organ ). The chordotonal organs, which are only found in insects and crustaceans, are not at all visible from the outside. Your scolopidia consist of one to four sensory neurons, an envelope cell (scolopal cell), several attachment cells and enveloping glial cells . How they work has not yet been clarified in detail, but modified cilia are definitely used for the actual stimulus absorption. So-called dome-shaped (campaniform) sensillae also occur in insects. The antennae are important organs of sense of touch, vibration and flow, and a special organ in the base of the antenna, the Johnston organ, serves for perception .
Gravity
Spiders and many insects perceive the force of gravity through the pulling forces of the body suspended by the legs, in spiders lyre-shaped organs are used for this, some insects perceive the pull of gravity on the antennae with the Johnstonian organs. Crustaceans living in water often show hardly any differences in density compared to the surrounding medium (they can float in water). They have therefore developed their own heavy sense organs, the statocysts . These consist of a capsule with numerous inward-pointing hair cells. During the molting process, a few grains of sand are inserted into this capsule, which, by pressing on the hair on one side, indicate the direction of gravity. The way it works is almost entirely the same as that of the vertebrate's sensory organs. Statocysts are mostly located in the basal antennae, but in some groups also at the end of the body in the uropods or in the telson.
Listen
Perception of sound waves is a special form of the vibration sense. Numerous arthropods can use the same hair sensors with which they perceive air movements (e.g. from an approaching predator) or vibrations. In some real spiders, such a sound perception with the trichobothria has been proven. In mosquitoes , the males recognize the females by the sound that is generated by the rapid vibration of the wings (and that humans can also hear). For this purpose, the antennae are greatly enlarged, the hearing organ is Johnston's organ. Acoustic communication through vibrations perceived with the trichobothria has also been demonstrated in a flagellated scorpion and may be widespread. In the case of insects, in addition to these more closely working sensors based on direct perception of particle movement, special organs have been developed that also react to pressure differences, ie perceive sound waves like the ears of vertebrates, these are called tympanic organs . In addition to the perception of enemies, sound perception is used to identify the sex of locusts and cicadas (and possibly water bugs), which are also capable of producing sounds. Parasitic flies have taken advantage of this by developing tympanic organs that can also hear the chants of crickets, which they can seek out to lay their eggs on. Most insects with tympanic organs, however, only use them for one purpose: to hear the ultrasonic locating sounds of bats. This ability has developed independently of one another in seven families of butterflies, fishing horrors, lacewings and two groups of beetles (tiger beetles and scarab beetles). The tympanic organs are in any case modified chordotonal organs in which the sensory cells lie on air sacs (enlarged trachea) inside the body. The position is extremely different, in the abdomen (cicadas, most butterflies, short-antennae terrors, tiger beetles), in the thorax (owl butterflies, fishing horrors), in the legs (long-antennae terrors), at the base of the wings (hawkers, lacewings), behind the head (flies , Scarab beetle), on the mouthparts (swarmers). While many groups can only hear a narrow band of frequencies in ultrasound, some have sophisticated distance and directional hearing.
It has only been proven for a few years that crabs ( Decapoda ) also have a sense of hearing. These can perceive low-frequency sound waves of around 100 to 3000 Hertz with their statocysts.
Chemical senses
Chemical sensory organs for near orientation (sense of taste) and for distant orientation (sense of smell) are present in all arthropods in the form of a large number of sensilla types. Especially in all groups without highly developed eyes, it is usually the most important organs for foraging. In addition, social communication and gendering in numerous arthropods are based on pheromones , which are perceived via chemical sensory organs. The most important sensory organs for chemical orientation are usually the antennas, often also the buttons (palps) and the outer (distal) leg links. In the absence of antennas and buttons, the leg receptor equipment can be similarly rich, e.g. B. with the flagellum spiders . In addition, many arthropods have chemical sensors scattered over their entire body surface. One to several hundred thousand chemoreceptors are regularly found on an arthropod antenna.
The sensils, especially the antennae, are divided into two different groups according to their morphology, the hair sensils and the pit sensils, which are connected by transitions (pin-shaped or conical appendages that lie in a pit). Furthermore, different types of hair are differentiated according to their morphology, namely dome-shaped (campaniformia), bottle-shaped (ampullacea), conical (basiconia) and a number of other types. Although the shape of the receptors is related to the function, it has been shown that sensory organs of the same shape have different functions in different groups of animals. It is typical for all types of chemoreceptors that their surface is covered by pores (in the micro to nanometer range). The hollow interior (lumen) of the Sensille is in contact with the outside world through these pores. Inward diffusing molecules are registered by specialized nerve cells, which represent the actual sensory cells. Very often a single sensory hair can process different sensory impressions, e.g. B. as a combined chemo and mechanoreceptor, rarely even three (trimodal). Usually five to ten different types of sensilla can be morphologically differentiated on an antenna.
Sensilles used for food acquisition typically have a relatively broad, but quite differentiated range of senses between different cells for a multitude of soluble or volatile substances such as amino acids, peptides, sugars, nucleotides or amines. The corresponding substance can then be determined from the excitation pattern of various sensors (similar to the sense of taste in humans). There are also highly specialized sensors for messenger substances (pheromones) that only perceive this single substance.
Carbon dioxide sensors
A number of arthropod species, including butterflies, mosquitoes, lobsters, millipedes and ticks, have special sensors that respond specifically to carbon dioxide. Mostly it is about sensilla on the antenna (often bottle-shaped sensilla), but also special sensory organs on the head surface have been developed for this, e.g. B. Millipedes. The biological purpose of this sensory performance is z. B. in finding a host in blood-sucking species or in avoidance reactions against asphyxiation in soil-dwelling species that live in narrow cavities.
Humidity and temperature sensors
Although strictly speaking these are not chemical senses, arthropods perceive them with similarly structured sensillae. Conical sensillae often serve to cover both properties, often even combined with one another. The specialized infrared radiation detectors of the "fire beetle" Melanophila have become famous . These animals can detect forest fires up to 12 kilometers away, which they target specifically to lay their eggs in the charred wood.
Magnetic and electrical sense
Perception of the earth's magnetic field is widespread in the animal kingdom and has also been demonstrated in many arthropods. Intracellular iron oxide particles are used for perception, as have been demonstrated, for example, in social insects and crabs. The magnetic sense is used in all cases for large-scale orientation in space.
While it was previously thought that arthropods lacked a sense of electrical fields, it has now been possible to prove such a sense in crustaceans. In terms of its sensitivity, however, it is an order of magnitude worse than the highly developed electrical sensory organs of many fish species. What the crabs use the sense for has not yet been clarified; it would be possible to participate in spatial orientation or in the perception of prey organisms.
Reproduction
Gender dimorphism
While, on the one hand, males and females can only be distinguished in many species of arthropods on the basis of their sexual organs, on the other hand, the sexes in various species also differ considerably externally.
An example of clear color differences between the sexes can be found in the great golden terrier ( Chrysochraon dispar ) from the order of the short-antennae terrors . Another example of clearly pronounced sexual dimorphism is the stag beetle ( Lucanus cervus ) from the order of the beetles . Here the males have significantly enlarged, antler-like mandibles with which they fight against each other for the less conspicuous females.
In some species there are dwarf males that are considerably smaller than their females, for example the swan ( Euproctis similis ) from the order of the butterflies or the wasp spider ( Argiope bruennichi ) from the order of the spider .
In insects there are many cases in which the males are winged in contrast to the females, for example the common cockroach ( Blatta orientalis ) from the order of the cockroaches or the sloe-brush moth ( Orgyia antiqua ), a butterfly. In the extreme case, the female is larva-like and quite large, while the male is capable of flight, full, developed and has considerably better developed sensory organs. An example of this is the little moth moth ( Heterogynis penella ), also from the order of butterflies.
Attractants, attracting strategies
With the arthropods, different ways of attracting the respective sexual partner have developed.
In many butterflies , the females have simple thread-shaped antennae, while the males have combed antennae, as they use the larger surface area to be able to perceive the sexual attractant of the female from as great a distance as possible.
Other species use visual signals: So the male waving fiddler crabs with their increased scissors, fireflies attract their mates on by a species-specific flash rhythm.
Noises are also used: wolf spiders courting with knock signals. Giant crab spiders attract females by vibrating the surface they are sitting on. Long- feeler terrors chirp ( stridulation ).
Mating and fertilization
Most arthropods have internal fertilization. Exceptions like Limulus are rare. The sperm are often combined in a spermatophore, which is taken up by the female or attached by the male in or on the female. In some species, therefore, legs have been transformed into gonopods , which are used for fertilization. The spermatophores often open long after copulation to fertilize the eggs.
In some insects, the sex organs of the males only match those of the females of the same species (lock-and-key principle), so that fertilization between different externally indistinguishable species is often not possible for this reason alone. Sometimes the females eat the males after mating, such as the great angle spider .
Special forms in the training of the sexes
Parthenogenesis is quite common in arthropods. It occurs in some mites (Acari), crabs (Crustacea) and in insects. Examples of this are the great sawfly and the summer generations of aphids , and winged sex animals appear in autumn.
Hermaphrodite is a rare exception among the Arthropoda. Cyatura carinate ( Isopoda ) and many scissors are first females and then males (protogynous hermaphrodite).
Brood care
In the area of brood care, there are all varieties of arthropods, from laying eggs in free water without any care to live- bearing animals, such as Euscorpius mesotrichus .
Insects often lay their eggs on the forage plants. Endoparasites such as wood wasps and the common oak gall wasp put them straight into the host. Female cave spiders and trembling spiders of the genus Pholcus cover their eggs with a web and carry the egg cocoon with them. The female crayfish carries the eggs stuck to the body with them until they hatch. Some species, such as the earwigs, guard their eggs in a protected cave until they hatch.
Similar to the marsupials, the woodlice have a breast pocket in which they carry their young around, as described for the basement wood louse and water wood louse . The spotty brood bug not only guards and protects its eggs, it later also leads the young to the food like a duck its young in single file. Some species like the common earwig and the crested-web spiders as well as most colony-forming insects go even further with brood care and feed their young.
development
In arthropod eggs, embryonic development begins with a total furrow in the case of egg cells with few yolks and superficial furrows at various points on the surface of egg cells with a large amount of yolk. With superficial furrowing, a spherical, single-celled layer arises around the yolk, often with numerous cell nuclei, but without cell boundaries ( syncytium ). Next, the cells migrate to one side of the yolk and form a germinal disc there, from which the embryo emerges.
Only in primarily aquatic groups does a primary larva hatch from the egg; in addition to most crustaceans, these are the woodlice spiders belonging to the Chelicerata . The crustaceans hatch with segments carrying three pairs of limbs (those of the first and second antennae and the mandibles) as nauplius larva , woodlice spiders with three segments (enclosures of the cheliforas, palps and brood legs) as protonymphone larva. In the following moults, further segments are added in a rear growth zone. The limbs of these segments are often created only as undivided buds and only gradually acquire a functional shape in the course of further moulting. The limbs of the existing front extremities are also remodeled during the later moulting, in some cases they are melted down (ie they are present in the larvae but not in the adult animals). In the case of crustaceans, in the course of a long development with numerous moults, the animal can pass through several anatomically and functionally completely different larval forms with different ways of life. This development, which was originally developed for the arthropods, in which the number of segments of the adult animal is gradually reached, is called anamorphosis (or anamerism ). In the original case, exactly one segment is added with each molt. In many arthropods with anamorphic development, however, several new segments can arise in one step.
In numerous groups, this original development is modified in that the early stages of development are already passed through in the egg, so that the first free-living larval stage is already more advanced in its development (even free-living nauplius larvae of many crustaceans do not eat). That these are modified forms can be shown, for example, by the fact that the embryo separates a cuticle at the appropriate stage. Finally, in numerous groups, independently of one another, it came about that all larval stages in the embryo were passed through, or the development was completely modified so that no trace of these stages remained. In these cases, a young animal hatches from the egg, the shape of which largely corresponds to that of the adult. This case is the rule with terrestrial arthropods, since stages corresponding to the nauplius or protonymphone would not be viable here. B. also for the lobsters belonging to the crustaceans. If the first free-living larva already has all the segments of the adult animal, one speaks of telomorphosis (or telomerism). Development without a (primary) larval stage is called direct development. Larvae, which largely correspond to the adult animal in its organization, also show, for example, the primarily marine horseshoe crabs , which belong to the Chelicerata. Direct development is typical e.g. B. for all real spiders and the more primitive hemimetabolic insects. In the case of the millipedes and the leg taster ( Protura ) belonging to the hexipedes , the hatched animal largely corresponds in shape and way of life to the adult, but further segments are formed with later moults.
Holometabolic insects are characterized by a completely modified development. With them there was secondary formation of a larva again, which has a completely different shape and way of life than the imago. The origin of these larval stages is not fully understood; it may go back to a stage known as the prolarve, which in many hemimetabolic insects is inserted between the embryo and the actual first larval stage. The tissue of the holometabolic larva is largely dissolved in a resting stage, the pupa, and the imago is completely rebuilt from bud-like structures (the imaginal discs).
Hatch with just a few segments (anamorphosis)
- the swordtails (Xiphosura) and woodlice spiders (Pycnogonida) among the jawbones (Chelicerata)
- most millipedes (Myriapoda) (except epimorphic Chilopoda)
- the protura among the six pods (Hexapoda)
and reach their final number of segments only after hatching.
With the full number of segments (telomorphosis) leave the egg
- the vast majority of jaw-claw carriers (Chelicerata)
- Insects (Insecta), double tails (Diplura) and springtails (Collembola) among the hexipedes
- the giant runners (Scolopendromorpha) and ground walkers (Geophilomorpha) among the centipedes (Chilopoda)
In insects there are all transitions between forms that already create all or almost all segments in the embryo at the same time ("long germ") and those in which the anamorphosis has been retained during embryonic development in the egg ("short germ").
height
Most arthropods are small animals. Although they overlap with the vertebrates in terms of size , they are by far predominantly significantly smaller than these. What is the upper limit of the body size of the otherwise so diverse and successful arthropods is an old controversial question. One theory suggests that the main limitation comes from the exoskeleton. A physique based on the exoskeleton is in principle capable of almost any size, especially since deep depressions (called apodemes) as muscle attachment points are shifted inside. But the Achilles heel of the exoskeleton is the moulting phase. Land-living giant arthropods, in particular, would literally melt under their own weight during the molt. Another theory sees the open blood circulation and the less efficient respiratory system compared to vertebrates as the main limitation. One argument for this view is that giant insects with bodies significantly larger than those of recent forms appeared in periods of the Paleozoic when the oxygen partial pressure of the atmosphere was significantly higher than today. Land-living arthropods breathe through air-filled tubes (trachea), while blood is not insignificant for oxygen transport, but is much less important than in vertebrates. Contrary to some opinion, the air transport in the trachea is not purely passive, but the animals can specifically press them together and thus significantly accelerate their breathing. Nevertheless, a disproportionate increase in the diameter of the trachea is probably necessary with increasing body size, the effect is particularly evident in the legs. As a result, at some point there is no longer enough space for other vital organs. At the same time, the open blood circulation does not allow a significant increase in blood pressure, so that the oxygen transport via the blood can only be optimized with difficulty. A third theory assumes that because of the bare nerve fibers of the arthropods that are not covered by Schwann cells , the reaction speed is too slow when the body is taller. However, myelin sheaths have also been detected in crustaceans. Arthropods also have giant fibers that can achieve the same high transmission speeds as vertebrate nerves for vital movements (e.g. flight reflexes). It is possible that the presence of vertebrates means that enlargement no longer offers any ecological advantages because the niche space available is already filled. Enlarging the body does not only offer advantages. Significant disadvantages are z. B. Extension of development time (and thus longer generation time), higher nutritional requirements due to increased metabolism and thus increased resting metabolism, increased risk of falling victim to predators. Whether there is such a thing as an optimal body size for the respective building plan and where this would be for the arthropod, is an open question within science. It is noticeable, however, that there are more medium-sized arthropods with body sizes in the centimeter range than very large and very small ones.
The largest known living arthropod is a marine crab, the Japanese giant crab . The palm thief ( Birgus latro ) is the largest land-living representative of the arthropods with a body length of up to 40 cm and a mass of up to 4 kg. There are particularly small arthropods (around 0.1 millimeters) within the mites, springtails and copepods. The smallest insects (parasitic hymenoptera) are hardly larger at 0.15 mm. Many organ systems are reduced in these dwarf forms. The segmentation of the body and legs is also usually indistinct.
Ways of life
Arthropods inhabit almost all known habitats and occupy a wide variety of ecological niches . Arthropods can therefore be considered the most successful animal order on earth.
Water and land
The original arthropods lived like trilobites in the sea. Of the (recent) groups living today, the crustaceans have most of the marine representatives. Among the chelicerates, woodlice spiders and horseshoe crabs are all marine, almost no species from the other groups (exception: a few marine mites). Neither millipedes nor hexipedes have produced true marine representatives, a few insect species such as the Clunio mosquito have only penetrated into the tidal flats.
Numerous crustaceans also live in freshwater, although there are groups that are much more species-rich in freshwater than in the sea, such as B. the gill pods . Under the jaw-claw carriers, the mites with the freshwater mites penetrated secondarily into the freshwater, ie their ancestors lived on land. The numerous insect species that live in freshwater can also be traced back to land-living ancestors. This is shown e.g. B. because they have a tracheal system for breathing , while the primarily aquatic crustaceans breathe with gills. Many aquatic insect larvae such as the larvae of the mayflies or the dragonflies have developed secondary gills again, but these are traversed by (air-filled) tracheas. Many insect larvae only absorb oxygen through their skin; some have simple tube gills filled with hemolymph to increase the surface area .
Many freshwater arthropods actually breathe air. They then have a tracheal system with open stigmas. Some can "fill up on air" with snorkel-like breathing tubes on the surface of the water like the larva of the dung bee . Others live in an air bubble like the water spider or hold an air envelope on the body surface like the yellow beetle .
Most insects live on the land, almost all arachnids and all millipedes. Some lines of development from the crabs have independently of one another passed over to rural life. Are best adapted to life on land while the terrestrial isopods , while terrestrial decapods (such as the hermit crabs and land crabs ) and amphipods at least have to return to breed in the water.
Locomotion
Apart from vertebrates, arthropods are the only group in the animal kingdom that has produced organisms that walk on legs (that is, not just push themselves forward). The functioning of the arthropod leg is exactly the same for aquatic and terrestrial forms. B. not possible to decide on the basis of the legs whether a fossil-preserved species was aquatic or land-living. Only a few groups gave up their legs secondarily, mostly parasitic forms. In many crustaceans, the extremities have two functions at the same time: They serve both for locomotion and for food acquisition. In anatomically more strongly derived, more modern forms, these functions are usually separate, ie different extremities are specialized in one or the other function. Most aquatic lines of evolution have produced swimming forms. Swimming organs are usually reshaped and specialized extremities. Some lineages of crustaceans have developed convergent leaf bones that are no longer suitable for walking. Forms belonging to the zooplankton (besides crustaceans some mites and insect larvae) are permanent swimmers. The development of wings was of extremely high importance for the radiation of insects . Acquiring the ability to fly was probably the key feature that made the flying insects by far the most species-rich group of organisms on the entire planet. In contrast to permanent swimmers, there are no permanent fliers. However, z. B. many dragonfly species do not walk with their legs, they only serve to hold on and to acquire food. It is often not taken into account that the spiders have developed a certain ability to fly. The flight of young spiders in particular on a thread of spider silk (" ballooning ") that they have given up allows them to spread rapidly into all suitable habitats. Some lines of development have developed special modes of locomotion. Many species such as jumping spiders , fleas, and springtails can jump very well. Shapes that crawl like snails, such as the larvae of the snail moth, are rare .
Forms of nutrition
robber
Many crabs, most spiders and various insects are predatory.
Parasitism in animals
Many species suck blood, such as the deer louse fly in deer , the red poultry mite in birds , fish lice in fish or the varroa mite in the western honey bee . The larva of the red velvet mite attaches itself to insects similar to the wood tick ( Ixodes ricinus ) in humans.
Mites infestation of the ear leads to ear mange . Hair follicle mites live in the hair follicle of mammals and feather mites on the feathers of birds. Many of these species are harmless to the host, others cause the mange .
Botflies live in the stomach, nose and throat and / or lungs of mammals.
Ichneumon wasps are internal parasites that lead to the death of the victim, therefore they are called parasitoids .
Plant nutrition
Various species such as spider mites and aphids suckle on plants.
Many butterflies , honey bees and various other animals live on the nectar of flowering plants.
Larvae of butterflies and beetles eat the leaves of plants. Gall wasps lay their eggs inside the plants.
Intruders in insect states
State-forming insects have complex olfactory communication, which some species use to be able to enter the states of other insects safely.
The skull-and- neck hawk feeds on honey directly from the honeycomb - the bees presumably do not attack it because of a smell mask.
The ichneumon Ichneumon Eumerus is a hyperparasite : You parasitized the phengaris rebeli ( Maculinea rebeli ), the parasite itself in ants.
Mushroom cultivation and keeping of pets for ants
Some ants breed mushrooms like the leaf cutter ants or keep aphids as pets like the red wood ant ( Formica rufa ) or the black garden ant ( Lasius niger ). Ants have very different and interesting relationships with the bluebirds , which can range from symbiosis to parasitism.
Systematics
External system
The system of arthropods is traditionally based primarily on the external body structure with the sequence of segments and limbs. Since the end of the 1990s, features of the nervous system, molecular pedigrees and developmental genetic features have been increasingly included in the analysis, as well as other features such as e.g. B. the fine structure of the sperm. Numerous groups of the traditional system that were believed to be very secure had to be modified or abandoned. The most important result of the "new" system is the division of the protostomia into two large groups Ecdysozoa and Lophotrochozoa . If this model, which has now been well established in various ways, is correct, the annelid worms can not be the sister group of the (pan) arthropods. This grouping ( articulated animals or articulata) was previously considered to be well protected. More recent results indicate that both the formation of the head segments and the development of the body appendages (legs or parapodia) are controlled very similarly in both groups. Since a convergent formation of this complicated sequence does not seem very likely, it would be possible that the common ancestor of the Ecdysozoa and Lophotrochozoa ("Ur-Protostomier") or even its common ancestor with the Deuterostomia ("Ur-Bilaterier") was segmented. The problem with this assumption, however, is that in the numerous non-segmented animal phyla, the segmentation would then have to have been secondary abandoned many times independently of one another. Current research findings are not sufficient to answer this question.
Within the Ecdysozoa, the sister group of Panarthropoda is a taxon of worm-like animals with a solid, periodically skinned body shell and a "brain" that surrounds the digestive tract in a ring, named after this characteristic Cycloneuralia. Another common feature is a portion of the mouth that can be turned out in almost all groups. The relationship between the three groups that together make up the Panarthropoda is unclear: tardigrade, columbus, arthropod. Although most taxonomists now consider a sister group relationship between the arthropod and the columbus to be more likely, the grouping of the arthropod with the tardigrade continues to have numerous followers. The (traditional) grouping of the tardigrade and columbus as a common sister group is mentioned less often, but it cannot be ruled out either.
Internal system
According to recent studies, the articulated animals are divided into the following monophyletic groups:
- Chelicerata ( jaw-claw bearer ). The position of the marine woodlouse spiders (Pycnogonida or Pantopoda), which in some studies had been compared as a basal group to all other arthropods, is somewhat problematic . However, the arguments put forward for this have now largely been refuted.
- Myriapoda ( millipede ).
- Tetraconata (also called Pancrustacea ). These include the crustaceans and the hexapods ( insects and pittans ).
The grouping of the hexipedes with the crustaceans is now quite well secured by numerous independent molecular pedigrees and is also supported by the anatomy of the nervous system and the eye. Although a number of studies support crustacean monophyly, it now seems most likely that these are paraphyletic with respect to the hexipedes, that is, the hexipedes are more closely related to some cancer orders than they are to each other. The hexipedes would then be something like a line of crustaceans that had passed over to land life. It is not yet clear which crustaceans could be the sister group of the hexapods (i.e., most closely related to them among the crustaceans). Possible candidates are the "higher" crabs ( Malacostraca ), the gill-pods (Branchiopoda) or the primeval Remipedia and Cephalocarida . The combination of the hexipedes with the millipedes ("Atelocerata" or " Tracheata "), which was previously favored , now hardly appears plausible. The most important similarities between the two groups are probably the convergences that resulted from the two common rural lifestyles. The important anatomical argument, the similar structure of the head in both groups with biting mandibles and regression of the second antennae, is only a weak explanation. In the meantime, suspicions that the Collembola ( springtails ) could descend from crustaceans independently of the other Hexapoda, whereby the Hexapoda would also be paraphyletic compared to the Crustacea, have not been confirmed.
The position of the millipedes is still controversial. Based on morphological findings, a grouping with the Tetraconata into a group called Mandibulata was considered to be well established. Quite surprisingly, some molecular trees were more closely related to the Chelicerata; the resulting group, for which there was initially no morphological argument at all, was therefore called "Paradoxopoda" (also: Myriochelata). Newer molecular pedigrees based on improved data again mainly support the mandibular hypothesis, but the findings are by no means unambiguous. In addition, a morphological argument in favor of the Paradoxopoda has also been put forward (due to the embryonic development of the nervous system), while other neuroanatomical studies support the mandibules. Although the situation can by no means be considered as clarified, more recent data predominantly support the mandibulata. In the meantime it has been assumed that the genealogical trees established on the basis of ribosomal RNA - which formed the essential basis for the Paradoxopoda hypothesis - are not suitable for solving the problem.
Further hypotheses are only of historical interest, e.g. B. the summary of all groups with one-branched legs (ie without split legs ) as "Uniramia" or the association (especially popular with paleontologists) of the Crustacea with the Chelicerata ("Schizoramia").
This results in the following hypothesis about the relationships (without fossil groups):
Arthropod (arthropoda) |
|
||||||||||||||||||
|
Evolution of the arthropod
Fossils of trunk group representatives of the arthropods have been found since the earliest Cambrian. It is therefore very likely that they actually originated in the late Precambrian (Ediacarian), even if there are no convincing fossils from this period. Since the earliest traces of fossils also come from the oldest Cambrian, a much earlier origin of the trunk is unlikely. In the early Cambrian Maotianshan slate from China or in the Middle Cambrian Burgess slate from Canada, arthropods are already rich in shape and differentiated with numerous species and individuals.
The main group of panarthropods is very likely to be the lobopods (or tardypolypods), a group of fossil "legged worms" that died out in the Ordovician and whose level of organization (not necessarily their actual origin) is reminiscent of the recent Onychophora. Primitive shapes like Aysheaia show an articulated body with repetitive (uniformly repeated) structures like the curled legs, but with no discernible real segments. Two longer appendages with stiff bristles are attached to the side of an undivided head, presumably serving to procure food; these are the only differentiated body appendages. Sclerotized plates did not occur on the trunk or legs. In the meantime, a whole “zoo” of partially bizarrely modified lobopods is known that lived around the same time. Some of them show sclerotized plates or thorns and a primitively segmented head with a pair or two of appendages (like Onychodictyion or the famous Hallucigenia ). One species, the "cactus" Diania cactiformis , had extremities with articulated sclerites similar to the leg of arthropods.
Fossils that are interpreted as the main group representatives of the real arthropods such as B. Fuxianhuia , Canadaspis or Shankouia have a number of new characteristics compared to the lobopods. The position of the mouth shifts slightly towards the abdomen (ventral) from the front end of the body. At the front end there is a head, protected by a dorsal sclerite (tergite), made up of two segments, the first of which has a pair of stalked eyes, while the second has sclerotized, articulated appendages that can be antenna-like or leg-like. The rest of the body is quite uniformly divided into segments, each protected by a dorsal, heavily sclerotized plate (tergite). On the underside sit stubby or leaf-shaped legs, which were mostly not or only weakly sclerotized and which could transport the animal over the ground in a kind of swimming-running movement. Leaf- or lobe-shaped appendages on the outside of the legs probably served as gills. More advanced forms have a head shield which, in addition to the eye and first extremity segments, covers three further segments without their extremities deviating particularly from the other trunk limbs. This five-segment head is considered by many researchers to be the basic blueprint for (eu-) arthropods. Whether appropriately constructed fossils are to be regarded as main group representatives of the arthropods or as more advanced representatives of a certain arthropod lineage such as the Crustacea or the Chelicerata depends critically on the interpretation of the front extremities and their segments. Most researchers see in the "large appendages" of numerous fossils such as Leanchoilia or Yohoia (leg-like, strongly bristled mouth appendages, which were presumably used to grab prey) homologous formations of the chelicerae or the (first) antennae of living arthropods. If one follows this interpretation, most of the forms can be understood as main group representatives of the Chelicerata. However, some researchers homologate these appendages with the antennae of the Onychophora, which are located on the foremost head segment (the segment that produces the foremost part of the brain, the protocerebrum), so they do not directly correspond to the antennae or chelicerae of the arthropods; accordingly, in their opinion, the same fossils belong to the parent group of all arthropods.
The ancestral group of crustaceans is already fossilized from the lower Cambrian (i.e. parallel to the lobopods). Physically preserved, phosphated fossils (so-called "Orsten") are particularly informative, although they are only micrometers to millimeters in size, but reveal the finest details of the body structure. Core group representatives and representatives of the modern crayfish (the "crown group") are morphologically very similar, but differ primarily in the structure of the head, which can consist of a different number of segments. The antenna pairs of species such as Oelandocaris , like the “large appendages”, were used primarily for feeding.
In addition to the forms that lead to the modern arthropods, there were numerous other arthropod lines from the Cambrian onwards that became extinct without modern descendants. The most famous of these lines are the trilobites . Before the rich Cambrian fossil sites with preserved soft tissues were discovered, the trilobites were the only fossil source for early arthropod development, which is why they greatly influenced earlier ideas about the arthropod tribe. Today the trilobites are already strongly derived and morphologically advanced in many respects special forms. Their traditional combination with the chelicerates in a taxon Arachnomorpha has recently been questioned with good arguments. Another famous sideline are the anomalocaridids . Anomalocaris is one of the most famous fossils of the Burgess slate, its relatives were the largest predators in the Cambrian Sea with heights of up to two meters. Another sideline, the Thylacocephala , died out at the end of the Cretaceous period. B. found in the slab limestone of Solnhofen. Cambrian precursors like Isoxys were among the most common free-swimming arthropods in the sea at that time. Another group, the Euthycarcinoidea , has remained a mystery despite well-preserved fossils and has been associated with pretty much every kin group of the arthropods without their actual relationship being clarified. If the interpretation of Cambrian trace fossils found in America is correct, they would have been the first land-living arthropods.
Fossils of the primarily land-living Hexapoda and Myriapoda are available in large numbers and in some cases in excellent preservation, but they shed little light on the origin of these classes. The oldest fossils of both groups come from the same deposit, the Devonian Deposits of Rhynie , Scotland. The animals found here were already living on the land and by and large had a fairly modern physique. Fossils from core group representatives (e.g. marine forms) are unknown, all fossils interpreted in this way are either questionable or their classification has even been refuted.
Importance to humans
Economical meaning
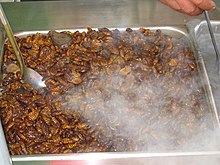
The primary role of arthropods for humans is probably in agriculture , as they play an outstanding role in the pollination of plants and thus a large part of our food . Some species are eaten themselves, such as the lobster and the North Sea shrimp , but in many cultures also insects ( entomophagy ). The honey bee provides us with honey . Various arthropods serve as food for food fish. On the other hand, arthropods include agricultural pests such as aphids and storage pests such as mealworms that feed on our food.
The caterpillars of the silk moth produce silk during pupation, which is used to make fabrics . In contrast, the caterpillars of the clothes moth eat fabrics made of wool .
In forestry , arthropods appear as important wood pests , but also as their enemies and therefore as beneficials . Wood pests also affect house and furniture construction.
health
Poison bites
While arthropods usually are not large enough to man injuries inflict that are dangerous only because of their size, but some of their stings and bites are very unpleasant because the animals leave the same poison in the wound, as for example in wasps or water spider of Case is. However, these are usually not seriously dangerous. Species in which a single sting or bite can be fatal are rarer, such as the black widow ( Latrodectrus tredecimguttatus , mortality without treatment 5%) or the scorpion Leiurus quinquestriatus . Some insects and spiders have stinging hairs to ward off predators, which can also be unpleasant for humans.
Parasites and vectors of disease
Arthropods also play a major role as human parasites and disease vectors.
There are various arthropods that suck blood in humans , such as the head louse , the pubic louse , the bed bug and the common wood tick, also called tick ( Ixodes ricinus ). Some of these are dreaded disease carriers, the transfers rat flea the plague , the tsetse fly spreads the sleeping sickness and the Anopheles mosquito , the malaria . Ticks transmit diseases such as borreliosis and early summer meningoencephalitis (TBE). The scabies mite eats burrows in the uppermost skin layer of mammals and humans and thereby causes scabies .
literature
- Heiko Bellmann : The new cosmos insect guide. Kosmos, Stuttgart 1999, ISBN 3-440-07682-2 .
- Heiko Bellmann: The new Kosmos butterfly guide. Butterflies, caterpillars and forage plants. Franckh-Kosmos, Stuttgart 2003, ISBN 3-440-09330-1 .
- Heiko Bellmann: Arachnids of Europe. Kosmos, Stuttgart 2001, ISBN 3-440-09071-X .
- Klaus Dumpert: The social life of the ants (= Parey's study texts , volume; 18), Parey, Berlin / Hamburg 1994, ISBN 3-489-63636-8 .
- RC Brusca, GJ Brusca: Invertebrates. 2nd Edition. Sinauer Associates, 2003, chap. 19, ISBN 0-87893-097-3 , p. 475.
- EE Ruppert, RS Fox, RP Barnes: Invertebrate Zoology - A functional evolutionary approach. Brooks / Cole 2004, chap. 16, ISBN 0-03-025982-7 , p. 517.
- DT Anderson: Invertebrate Zoology. 2nd Edition. Oxford Univ. Press, 2001, chap. 10, ISBN 0-19-551368-1 , p. 225.
- J. Moore: An Introduction to the Invertebrates. Cambridge Univ. Press, 2001, chap. 12, ISBN 0-521-77914-6 , p. 174.
- Jens-Wilhelm Janzen: Arthropods in Baltic Amber. Ampyx, Halle (Saale) 2002, ISBN 3-932795-14-8 .
- H. Paulus: Euarthropda, Arthropod ie S. In: Rieger Westheide (Ed.): Special Zoology. Part 1: Protozoa and invertebrates. Springer Spectrum, Berlin / Heidelberg 2013, ISBN 978-3-642-34695-8 .
- Horst Aspöck: Sick through arthropods - arthropods as pathogens and carriers of diseases in humans (= Denisia , Volume 30), Biology Center - Upper Austrian State Museums, Linz 2010, OCLC 750655600 DNB 1022693654 .
Scientific literature
- M. Akam: Arthropods: Developmental diversity within a (super) phylum. In: Proceedings of the National Academy of Sciences (USA). 97 (2000), p. 4438.
- P. Ax: The system of Metazoa II. A textbook of phylogenetic systematics. Gustav Fischer Verlag, 1999.
- RC Brusca: Unraveling the history of arthropod diversification. In: Annals of the Missouri Botanical Garden. 87 (2000), p. 13.
- CE Cook, ML Smith, MJ Telford, A. Bastianello, M. Akam: Hox genes and the phylogeny of the arthropods. In: Current Biology. 11 (2001), p. 759.
- GD Edgecombe, GDF Wilson, DJ Colgan, MR Gray, G. Cassis: Arthropod cladistics: Combined analysis of histone H3 and U2 snRNA sequences and morphology. In: Cladistics. 16 (2000), p. 155.
- G. Giribet, GD Edgecombe, WC Wheeler: Arthropod phylogeny based on eight molecular loci and morphology. In: Nature. 413 (2001), p. 157.
- UWM Hwang, M. Friedrich, D. Tautz, CJ Park, W. Kim: Mitochondrial protein phylogeny joins myriapods with chelicerates. In: Nature. 413 (2001), p. 154.
- Jan Zrzavý , Pavel Štys : The basic body plan of arthropods: Insights from evolutionary morphology and developmental biology. In: Journal of Evolutionary Biology. 10 (1997), p. 353.
- Hans Ekkehard Gruner (Ed.), M. Moritz, W. Dunger: Textbook of special zoology. Volume I: Invertebrates. 4th part: Arthropoda (without insects). 1993.
- Volker Storch, Ulrich Welsch: Kükenthal zoological internship. 26th edition. Spectrum, Akademischer Verlag, Heidelberg 2009, ISBN 978-3-8274-1998-9 .
Web links
- arthropods.de
- Taz.de , January 30, 2016, Jörg Zittlau: The big crawling under the bed
Individual evidence
- ↑ Andreas Hassl : Medical Entomology & Hirudineology & Vertebratology . 2nd German edition v6.3. Script for the lecture WS 2011/12. Self-published, Vienna, A. 25 pp.
- ^ Arthur D. Chapman: Numbers of Living Species in Australia and the World. 2nd Edition. Report for the Australian Biological Resources Study, Canberra, Australia September 2009, ISBN 978-0-642-56861-8 . (on-line)
- ↑ Michael F. Land, Dan-E. Nilsson: General purpose and special purpose visual systems. In: Invertebrate Vision. Cambridge University Press, Cambridge, UK 2006, ISBN 0-521-83088-5 , pp. 167-210. doi : 10.2277 / 0521830885 Download
- ^ Dan-Eric Nilsson: The evolution of eyes and visually guided behavior. In: Philosophical Transactions of the Royal Society. Series B 364. (2010), pp. 2833-2847. doi : 10.1098 / rstb.2009.0083
- ^ T. Ryan Gregory: The evolution of complex organs. In: evolution. Education and Outreach. 1 (2008), pp. 358-389. doi : 10.1007 / s12052-008-0076-1
- ↑ Carsten HG Müller, Andy Sombke, Jörg Rosenberg: The fine structure of the eyes of some bristly millipedes (Penicillata, Diplopoda): additional support for the homology of mandibulate ommatidia. In: Arthropod structure & development. 36 (4) 2007, pp. 463-476. doi : 10.1016 / j.asd.2007.09.002
- ↑ Steffen Harzsch, Kathia Vilpoux, David C. Blackburn, David Platchetzki, Nadean L. Brown, Roland Melzer, Karen E. Kempler, Barbara A. Battelle: Evolution of Arthropod Visual Systems: Development of the Eyes and Central Visual Pathways in the Horseshoe Crab Limulus polyphemus Linnaeus, 1758 (Chelicerata, Xiphosura). In: Developmental Dynamics . 235 (2006), pp. 2641-2655. doi : 10.1002 / dvdy.20866
- ↑ Thomas W. Cronin, Megan L. Porter: Exceptional Variation on a Common Theme: The Evolution of Crustacean Compound Eyes. In: Evolution: Education and Outreach. 1: pp. 463-475. doi : 10.1007 / s12052-008-0085-0
- ^ Elke K. Buschbeck, Markus Friedrich: Evolution of Insect Eyes: Tales of Ancient Heritage, Deconstruction, Reconstruction, Remodeling, and Recycling. In: Evolution: Education and Outreach. 1: pp. 448-462. doi : 10.1007 / s12052-008-0086-z
- ↑ Overview in: Rolf Elofsson: The frontal eyes of crustaceans. In: Arthropod Structure & Development. 35 (2006), pp. 275-291. doi : 10.1016 / j.asd.2006.08.004
- ^ Kaspar P. Mueller, Thomas Labhart: Polarizing optics in a spider eye. In: Journal of Comparative Physiology. 196 (5) 2010, pp. 335-348. doi : 10.1007 / s00359-010-0516-6
- ↑ Overview in: Carlo Musio, Silvia Santillo: Non-visual photoreception in invertebrates. 2009 Download
- ↑ Jerome Casas, Olivier Dangles: Physical Ecology of Fluid Flow Sensing in Arthropods. In: Annual Revue of Entomology. 55. (2010), pp. 505-520. doi : 10.1146 / annurev-ento-112408-085342
- ↑ Sanjay P. Sane1 Matthew J. McHenry: The biomechanics of sensory organs. Integrative and Comparative Biology. (2009), pp. I8-i23. doi : 10.1093 / icb / icp112
- ^ Friedrich G. Barth : Spider mechanoreceptors Current Opinion. In: Neurobiology. 14 (2004), pp. 415-422. doi : 10.1016 / j.conb.2004.07.005
- ↑ Jayne E. Yack: The Structure and Function of Auditory Chordotonal Organs in Insects. In: Microscopy Research and Technique . 63 (2004), pp. 315-337. doi : 10.1002 / jemt.20051
- ↑ Martin Göpfert, Hans Briegel, Daniel Robert: Mosquito hearing: sound-induced antennal vibrations in male and female Aedes aegypti. In: The Journal of Experimental Biology 202. (1999), pp. 2727-2738.
- ^ Roger D. Santer & Eileen A. Hebets (2008): Agonistic signals received by an arthropod filiform hair allude to the prevalence of near-field sound communication. Proceedings of the Royal Society Series B 275: 363-368. doi : 10.1098 / rspb.2007.1466
- ^ Daniel Robert & Martin C Göpfert: Novel schemes for hearing and orientation in insects. In: Current Opinion in Neurobiology. 12 (2002), pp. 715-720.
- ↑ A. Stumpner & D. of Helversen: Evolution and function of auditory system in insects. In: Natural Sciences. 88 (2001), pp. 159-170 doi : 10.1007 / s001140100223
- ↑ JM Lovell, MM Findlay, RM Moate, HY Yan: The hearing abilities of the prawn Palaemon serratus. In: Comparative Biochemistry and Physiology. Part A 140 (2004), pp. 89-100. doi : 10.1016 / j.cbpb.2004.11.003
- ↑ K. Dumpert: Construction and distribution of the sensillae on the antenna flagella of Lasius fuliginosus (LATR.) (Hymenoptera, Formicidae). In: Journal for Morphology of Animals. 73: 95-116 (1972).
- ↑ J. Esslen, KE Kaissling: Number and distribution of antennal sensillae in honeybees (Apis mellifera L. In:) . Zoom morphology. 83, pp. 227-251 (1976).
- ^ Alfred Ernst, Jörg Rosenberg, Gero Hilken: Structure and distribution of antennal sensilla in the centipede Cryptops hortensis (Donovan, 1810) (Chilopoda, Scolopendromorpha). In: Soil Organisms. 81 (3) 2009, pp. 399-411.
- ↑ Rainer Foelix, Eileen Hebets: Sensory biology of whip spiders. (Arachnida, Amblypygi). In: Andrias. 15 (2001), pp. 129-140.
- ^ Charles D. Derby, Pascal Steullet, Amy J. Horner, Holly S. Cate: The sensory basis of feeding behavior in the Caribbean spiny lobster, Panulirus argus. In: Marine and Freshwater Research. 52 (2001), pp. 1339-1350.
- ^ Gert Stange & Sally Stowe: Carbon-dioxide sensing structures in terrestrial arthropods. In: Microscopy research & technique 47. (1999), pp. 416-427.
- ^ Hugh M. Robertson, Lauren B. Kent: Evolution of the gene lineage encoding the carbon dioxide receptor in insects . In: Journal of Insect Science . tape 9 , no. 1 , January 1, 2009, ISSN 1536-2442 , p. 19 , doi : 10.1673 / 031.009.1901 ( oxfordjournals.org ).
- ↑ Eliane Wajnberg, Daniel Acosta-Avalos, Odivaldo Cambraia Alves, Jandira Ferreira de Oliveira, Robert B. Srygley, Darci MS Esquivel: Magnetoreception in eusocial insects: an update. In: Journal of the Royal Society Interface. 7 (2010), pp. S207-S225. doi : 10.1098 / rsif.2009.0526.focus
- ↑ Kenneth J. Lohmann: Magnetic remanence in the Western Atlantic Spiny Lobster, Panulirus argus. In: Journal of Experimental Biology. 113: 29-41 (1984).
- ^ BW Pattullo, DL Macmillan: Making sense of electrical sense in crayfish. In: Journal of Experimental Biology. 213 (2010), pp. 651-657. doi : 10.1242 / jeb.039073
- ↑ Jon F. Harrison, Alexander Kaiser, John M. Vanden Brooks: Atmospheric oxygen level and the evolution of insect body size. In: Proceedings of the Royal Society. Series B 277 (2010), pp. 1937-1946. doi : 10.1098 / rspb.2010.0001
- ↑ Karel Slama: A new look at insect respiration. In: Biological Bulletin. 175: 289-300 (1988).
- ↑ John J. Socha, Wah-Keat Lee, Jon F. Harrison, James S. Waters, Kamel Fezza, Mark W. Westneat: Correlated patterns of tracheal compression and convective gas exchange in a carabid beetle. In: The Journal of Experimental Biology. 211 (2008), pp. 3409-3420. doi : 10.1242 / jeb.019877
- ↑ Alexander Kaiser, C. Jaco Klok, John J. Socha, Wah-Keat Lee, Michael C. Quinlan, Jon F. Harrison: Increase in tracheal investment with beetle size supports hypothesis of oxygen limitation on insect gigantism. In: Proceedings of the National Academy of Sciences USA. 104 (32) 2007, pp. 13198-13203. doi : 10.1073 / pnas.0611544104
- ↑ PH Lenz, DK Hartline, AD Davis: The need for speed. In: I. Fast reactions and myelinated axons in copepods. Journal of Comparative Physiology A 186. (2000), pp. 337-345.
- ↑ cf. in addition z. E.g. Joel G. Kingsolver, Raymond B. Huey: Size, temperature, and fitness: three rules. In: Evolutionary Ecology Research. 10 (2008), pp. 251-268.
- ↑ Ilse Bartsch (2004): Geographical and ecological distribution of marine halacarid genera and species (Acari: Halacaridae) Experimental and Applied Acarology 34: 37-58. doi : 10.1023 / B: APPA.0000044438.32992.35
- ↑ Patrick RH Steinmetz, Roman P. Kostyuchenko, Antje Fischer, Detlev Arendt: The segmental pattern of otx, gbx, and Hox genes in the annelid Platynereis dumerilii. In: Evolution & Development. 13 (1) 2011, pp. 72-79 doi : 10.1111 / j.1525-142X.2010.00457.x
- ↑ z. B. Lahcen I. Campbell, Omar Rota-Stabelli, Gregory D. Edgecombe, Trevor Marchioro, Stuart J. Longhorn, Maximilian J. Telford, Hervé Philippe, Lorena Rebecchi, Kevin J. Peterson, Davide Pisani: MicroRNAs and phylogenomics resolve the relationships of Tardigrada and suggest that velvet worms are the sister group of Arthropoda. In: Proceedings of the National Academy of Sciences USA. 2011. doi : 10.1073 / pnas.1105499108
- ↑ Also based on the blood pigment hemocyanin : Beyhan Ertas, Björn M. von Reumont, Johann-Wolfgang Wägele, Bernhard Misof, Thorsten Burmester: Hemocyanin Suggests a Close Relationship of Remipedia and Hexapoda. In: Molecular Biology and Evolution. 26 (12) 2009, pp. 2711-2718. doi : 10.1093 / molbev / msp186
- ↑ z. BJ Mallatt, G. Giribet: Further use of nearly complete 28S and 18S rRNA genes to classify Ecdysozoa: 37 more arthropods and a kinorhynch. In: Molecular Phylogenetics and Evolution. 40 (3) 2006, pp. 772-794. doi : 10.1016 / j.ympev.2006.04.021
- ^ Georg Mayer, Paul M. Whitington: Velvet worm development links myriapods with chelicerates. In: Proceedings of the Royal Society London. Series B 276 (2009), pp. 3571-3579. doi : 10.1098 / rspb.2009.0950
- ^ Nicholas J. Strausfeld, David R. Andrew: A new view of insect-crustacean relationships I. Inferences from neural cladistics and comparative neuroanatomy. In: Arthropod Structure & Development. 40 (3) 2011, pp. 276-288. doi : 10.1016 / j.asd.2011.02.002
- ↑ Björn M. von Reumont, Karen Meusemann, Nikolaus U. Szucsich, Emiliano Dell'Ampio, Vivek Gowri-Shankar, Daniela Bartel, Sabrina Simon, Harald O. Letsch, Roman R. Stocsits, Yun-xia Luan, Johann Wolfgang Wägele, Günther Pass, Heike Hadrys, Bernhard Misof: Can comprehensive background knowledge be incorporated into substitution models to improve phylogenetic analyzes? A case study on major arthropod relationships. In: BMC Evolutionary Biology. 9 (2009), p. 119. doi : 10.1186 / 1471-2148-9-119 (open access)
- Jump up ↑ Maximilian J. Telford, Sarah J. Bourlat, Andrew Economou, Daniel Papillon, Omar Rota-Stabelli: The evolution of the Ecdysozoa. In: Philosophical Transactions of the Royal Society. Series B 363 (2008), pp. 1529-1537. doi : 10.1098 / rstb.2007.2243
- ↑ Omar Rota-Stabelli, Lahcen Campbell, Henner Brinkmann, Gregory D. Edgecombe, Stuart J. Longhorn, Kevin J. Peterson, Davide Pisani, Hervé Philippe, Maximilian J. Telford: A congruent solution to arthropod phylogeny: phylogenomics, microRNAs and morphology support monophyletic mandibulata. In: Proceedings of the Royal Society London. Volume 278, number 1703, January 2011, pp. 298-306, doi : 10.1098 / rspb.2010.0590 , PMID 20702459 , PMC 3013382 (free full text).
- ^ Gregory D. Edgecombe: Arthropod phylogeny: An overview from the perspectives of morphology, molecular data and the fossil record. In: Arthropod Structure & Development. 39 (2010), pp. 74-87. doi : 10.1016 / j.asd.2009.10.002
- ↑ Karen Meusemann, Björn M. von Reumont, Sabrina Simon, Falko Roeding, Sascha Strauss, Patrick Kück, Ingo Ebersberger, Manfred Walzl, Günther Pass, Sebastian Breuers, Viktor Achter, Arndt von Haeseler, Thorsten Burmester, Heike Hadrys, J. Wolfgang Wägele, Bernhard Misof (2010): A Phylogenomic Approach to Resolve the Arthropod Tree of Life. In: Molecular Biology and Evolution. 27 (11) 2010, pp. 2451-2464. doi : 10.1093 / molbev / msq130
- ↑ Jerome C. Regier, Jeffrey W. Shultz, Andreas Zwick, April Hussey, Bernard Ball, Regina Wetzer, Joel W. Martin, Clifford W. Cunningham: Arthropod relationships revealed by phylogenomic analysis of nuclear protein-coding sequences. In: Nature. 463 (2010), pp. 1079-1083. doi : 10.1038 / nature08742
- ↑ Jianni Liu, Degan Shu, Jian Han, Zhifei Zhang, Xingliang Zhang: Origin, diversification, and relationships of Cambrian lobopods. In: Gondwana Research . 14 (2008), pp. 277-283. doi : 10.1016 / j.gr.2007.10.001
- ↑ Jerzy Dzik: The xenusian-to-anomalocaridid transition within the lobopodians. In: Bollettino della Società Paleontologica Italiana. 50 (1) 2011, pp. 65-74. Download
- ↑ Jianni Liu, Michael Steiner, Jason A. Dunlop, Helmut Keupp, Degan Shu, Qiang Ou, Jian Han, Zhifei Zhang, Xingliang Zhang: An armored Cambrian lobopodian from China with arthropod-like appendages. In: Nature . 470 (2011), pp. 526-530 doi : 10.1038 / nature09704
- ↑ Dieter Waloszek, Junyuan Chen, Andreas Maas, Xiuqiang Wang: Early Cambrian arthropods - new insights into arthropod head and structural evolution. In: Arthropod Structure & Development. 34 (2005), pp. 189-205. doi : 10.1016 / j.asd.2005.01.005
- ↑ Jun-Yuan Chen: The sudden appearance of various animal body plans during the Cambrian explosion. In: International Journal of Developmental Biology 53. (2009), pp. 733-751 doi : 10.1387 / ijdb.072513cj
- ↑ Graham E. Budd, Maximilian J. Telford: The origin and evolution of arthropods. In: Nature. 457 (12) 2009, pp. 812-817. doi : 10.1038 / nature07890
- ↑ Martin Stein, Dieter Waloszek, Aandreas Maas: Oelandocaris oelandica and its significance to resolving the stemlineage of Crustacea. In: S. Koenemann, R. Vonck (Eds.): Crustacea and Arthropod Relationships. Crustacean Issues 16 (2005), pp. 55-71.
- ↑ Martin Stein, Dieter Waloszek, Aandreas Maas, Joachim T. Haug, Klaus J. Müller (2008): The stem crustacean Oelandocaris oelandica re-visited. In: Acta Palaeontologica Polonica. 53 (3) 2008, pp. 461-484. Download (PDF; 2.1 MB)
- ^ Gerhard Scholtz, Gregory D. Edgecombe: The evolution of arthropod heads: reconciling morphological, developmental and palaeontological evidence. In: Development, Genes and Evolution. 216 (2006), pp. 395-415. doi : 10.1007 / s00427-006-0085-4
- ↑ Jean Vannier, Jun-Yuan Chen, Di-Ying Huang, Sylvain Charbonnier, Xiu-Qiang Wang (2006): The Early Cambrian origin of thylacocephalan arthropods. In: Acta Palaeontologica Polonica. 51 (2) 2006, pp. 201-214. Download (PDF; 1.4 MB)
- ^ NE Vaccari, GD Edgecombe, C. Escudero: Cambrian origins and affinities of an enigmatic fossil group of arthropods. In: Nature. 430 (2004), pp. 554-557. doi : 10.1038 / nature02705