L -Gulonolactone Oxidase
L-gulonolactone oxidase | ||
---|---|---|
Properties of human protein | ||
Mass / length primary structure | in humans without translation | |
Identifier | ||
External IDs |
|
|
Enzyme classification | ||
EC, category | 1.1.3.8 , oxidoreductases | |
Response type | oxidation | |
Substrate | L - gulonolactone + O 2 | |
Products | Ascorbic acid + hydrogen peroxide | |
Occurrence | ||
Parent taxon | Vertebrates | |
Exceptions | Dry-nosed primates , guinea pigs , real bony fish , some passerine birds and bat families | |
Orthologue | ||
human | mouse | |
Entrez | 2989 | 268756 |
Ensemble | ENSG00000234770 | ENSMUST00000059970 |
UniProt | P58710 | P58710 |
Refseq (mRNA) | NG_001136.2 | NM_178747.3 |
Refseq (protein) | NP_848862.1 | |
Gene locus | Chr 8: 27.42 - 27.45 Mb | Chr 14: 65.99 - 66.01 Mb |
PubMed search | 2989 |
268756
|
L- gulonolactone oxidase ( GULO , Gulo or GLO ), also known as L- gulono-γ-lactone oxidase , is an enzyme from the group of oxidases that isvery importantfor the production of ascorbic acid (vitamin C) in higher organisms. It catalyzes the last step of the biosynthesis of ascorbic acidwith the selective oxidation of L- gulonolactone (also called L- gulono-1,4-lactone or L- gulono-γ-lactone). The L -Gulonolactonoxidase is found in almost all vertebrates (vertebrates), and - according to current knowledge (2013) - even with very many invertebrates (invertebrates).
The L -Gulonolactonoxidase is by expression of a gene , the gulo produced gene. A genetic defect triggered by a mutation means that the affected organism can no longer produce ascorbic acid. Without adequate vitamin C intake through food, such organisms develop hypovitaminosis C - called scurvy in humans . In humans and numerous other vertebrate groups, u. a. In all real bony fish (Teleostei), most taxa of the bats (Chiroptera) and some taxa of passerine birds (Passeriformes) as well as all guinea pigs (Caviidae) the genetic inability to produce ascorbic acid corresponds, however, to a normal state acquired in the course of evolution. Vitamin C deficiency symptoms only occur in exceptional situations due to a food that is generally rich in vitamin C. While in humans and the other affected amniotes Gulo is present as a pseudogene and is therefore also called GULOP or GuloP ( P stands for 'pseudo'), it is no longer detectable in real bony fish.
Only the loss of function of L- gulonolactone oxidase makes ascorbic acid a ' vitamin ' for the species concerned by definition . For all other species with functional L- gulonolactone oxidase, ascorbic acid is just a metabolite .
Function and description
Ascorbic acid is vital ( essential ) for all plants and animals . As autotrophic organisms, plants do not have any exogenous sources to meet their ascorbic acid requirements. They are therefore all dependent on their own synthesis of ascorbic acid. On the other hand, animals that are fundamentally heterotrophic can in principle meet their ascorbic acid requirements by eating plants, for example. Nevertheless, the vast majority of vertebrates are able to synthesize ascorbic acid themselves. With the very large number of invertebrates, the knowledge about which species are able to synthesize ascorbic acid is still very sketchy and in some cases contradictory. The biosynthesis of ascorbic acid in plants is fundamentally different from that in animals. In higher plants, for example, in the last synthesis step, L - galactono-1,4-lactone is the substrate for the enzyme L- galactono-1,4-γ-lactone dehydrogenase (GLDH). L- gulonolactone oxidase does not play a role in the biosynthesis of ascorbic acid in plants.
In animals, biosynthesis begins with D - glucose (grape sugar). It is enzymatically converted into ascorbic acid in four stages via the intermediate products D - glucuronic acid , L - gulonic acid and L- gulono-1,4-lactone. The enzyme L- gulonolactone oxidase is required for the last step of biosynthesis in animals . It catalyzes the oxidation of L- gulono-1,4-lactone to ascorbic acid. This reaction also requires oxygen, which is supplied to the ascorbic acid-producing cells via the blood vessels . Together with two hydrogen atoms, which are removed from the ring system of L- gulonolactone in the 3,4-position during the reaction, hydrogen peroxide is formed as a by-product of the reaction .
Organisms that lack the enzyme L- gulonolactone oxidase or that do not function properly due to a mutation cannot produce ascorbic acid themselves. These organisms depend on the ingestion of sufficient quantities of ascorbic acid from food. Otherwise, they will fall ill with a vitamin C deficiency.

L- gulonic acid ( 1 ) is converted to L- gulonolactone ( L- gulono-1,4-lactone) ( 2 ) under the catalytic influence of a glucono-lactonase ( A ) . In the last step, L- gulonolactone oxidase ( B ) catalyzes the selective oxidation of L- gulonolactone to 2-keto- L- gulonlactone ( 3a ), which tautomerizes spontaneously to ascorbic acid ( 3b ) . Hydrogen peroxide (H 2 O 2 ) is formed as a by-product of the oxidation . If an organism lacks the enzyme L- gulonolactone oxidase, it cannot produce ascorbic acid itself. Then it is dependent on the exogenous intake of ascorbic acid for survival . Otherwise, he will fall ill due to the vitamin C deficiency, which will lead to death after several months.
L- gulonolactone oxidase is a microsomal enzyme. In rats and other gulo-positive mammals, it is found in the microsomes of the hepatocytes (liver cells). It is a membrane-bound enzyme, the active side of which protrudes into the lumen of the microsome. The oxidized substrate - ascorbic acid - is released extraluminally in the direction of the endoplasmic reticulum . The hydrogen peroxide that is also produced during the reaction is reduced by equivalent amounts of glutathione . The preferred substrate for L- gulonolactone oxidase is L- gulono-1,4-lactone. In addition, it is also able to catalyze the oxidation of L - galactonolactone , D - mannonolactone and D - altronolactone . In contrast, the oxidation of other γ-lactones , such as L - idonolactone or D- gluconolactone , is not catalyzed. Obviously, the suitable substrates must have a hydroxy group on the second carbon atom . The Michaelis constant (K m value) of L- gulonolactone oxidase is in the range from 0.007 to 0.15 mM. In principle, the electron transfer from L- gulonolactone oxidase is not limited to oxygen as the electron acceptor . Other oxidizing agents such as phenazine methosulfate or potassium hexacyanidoferrate (III) can also oxidize L- gulono-1,4-lactone to ascorbic acid using L- gulonolactone oxidase. L- gulonolactone oxidase isolated from the rat liver consists of 440 amino acids and has a molar mass of 50,605 g / mol. The gene coding for this enzyme has an open reading frame of 1320 nucleotides .
Occurrence and evidence

The gulo gene, which codes for the enzyme L- gulonolactone oxidase, is found in almost all vertebrates. It is primarily used by cells in the liver or the kidneys expressed . These two organs are the main producers of ascorbic acid in vertebrates. In the course of evolution, a change in ascorbic acid synthesis from the kidneys to the liver took place independently of one another in different lines of development in vertebrates. Thus, in fish , amphibians , reptiles and evolutionarily older bird - orders as well as the egg-laying mammals ( monotremes , monotremes) produced ascorbic acid in the kidneys. In contrast, the production of ascorbic acid takes place in the evolutionary younger bird orders and in the higher mammals (placentalia) in the liver. Marsupials (Marsupialia) produce ascorbic acid in both the kidneys and the liver. The transition to larger liver may be the result of a higher selection pressure to under stress conditions , the homeostasis to better maintained.
Gulo is expressed by many organisms at a later stage in their individual development. For example, rat fetuses are only able to produce ascorbic acid from day 16. The expression of gulo can be increased by various stimuli. This includes, for example, glycogenolysis (the breakdown of glycogen ). Various drugs such as barbiturates , phenazone or aminophenazone , as well as carcinogens such as methylcholanthrene or benzo [ a ] pyrene , increase gulo expression in test animals. The reason for this is probably the increased need for glucuronic acid to detoxify these xenobiotics . Obviously, all enzymes in the glucuronic acid pathway are upregulated.
Some species are unable to synthesize ascorbic acid on their own. According to the current status, the cause for this is always a defect in the gulo gene or its deletion .
Up until the 1970s, the classic detection method for a defective or missing gulo gene consisted of feeding test animals as free from ascorbic acid as possible and then examining them for symptoms of vitamin C deficiency. Thereafter, in vitro developed techniques in which one, for example from liver or kidney of the to be tested species with tissue homogenates L -Gulono-1,4-lactone, - the precursor molecule of the ascorbic acid in the biosynthesis - offset and the catalytic under the The influence of L- gulonolactone oxidase determined the amount of ascorbic acid formed. Both are indirect methods of detecting the presence of L- gulonolactone oxidase. Modern methods of gene expression analysis from Gulo are based, for example, on Gulo-specific antibodies and Western Blot , as well as on fluorescence in situ hybridization .
Invertebrates and Basal Vertebrates
In random tests on invertebrates ("Invertebrata") and fish, initially no indications of the activity of L- gulonolactone oxidase or, in general, of the ability of these animals to be able to synthesize ascorbic acid were found. One of these species is, for example, the desert locust ( Schistocerca gregaria ). In the 1970s, these results gave rise to the view that fish, insects and other invertebrates are fundamentally unable to produce ascorbic acid. Since it was known that L- gulonolactone oxidase is present in active form in modern amphibians , the hypothesis was supplemented by the postulate that L- gulonolactone oxidase was only present in the course of the vertebrate land shift , which occurred for the period before approx. 416 to 359 Millions of years ago it is believed to be a newly acquired characteristic. The need for ascorbic acid, so the argumentation, is significantly higher due to the increased oxidative stress associated with going ashore.
In establishing this hypothesis, however, older studies that were in direct contradiction to it were not taken into account. As early as 1922, for example, the “model organism” Drosophila melanogaster (black-bellied fruit fly) was found to function without ascorbic acid in the diet. The same applies to the red cotton bollworm ( Pectinophora gossypiella ) and the moth Argyrotaenia velutinana . In addition, were systematic errors committed. The examined fish all belonged to the real bony fish (Teleostei), which are, however, a relatively strongly derived group of the ray fins (Actinopterygii). After the ability to synthesize ascorbic acid could be demonstrated in the following years in the more primordial ray fins, lung fish (Dipnoi), sharks (Selachii) and rays (Batoidea), the assumption that this ability in vertebrates was only acquired evolutionarily by going ashore was no longer durable. After active L- gulonolactone oxidase was detected in 1998 in the sea lamprey ( Petromyzon marinus ), a very original vertebrate, it can also be assumed that ascorbic acid synthesis is an original feature of all vertebrates, which only got lost in a few lines of development. In invertebrates, however, the knowledge of the ability of ascorbic acid synthesis is still too incomplete to be able to determine at present (2013) when this ability, made possible by L- gulonolactone oxidase, first appeared in the course of evolution.
"Higher" vertebrates without L- gulonolactone oxidase
In all vertebrates that are unable to synthesize ascorbic acid themselves, the cause is always the gulo gene, the gene product of which catalyzes the last step of the biosynthesis to ascorbic acid. In none of these animals is a genetic defect in one of the other three enzymes involved in ascorbic acid biosynthesis the cause. The explanation for this is that a defect in Gulo only affects the synthesis of ascorbic acid, while a genetic defect with regard to other enzymes would interrupt the biosynthesis of other substances. For example, a genetic defect that prevented the production of glucono-lactonase would not only interrupt the synthesis of L- gulonolactone, but also, among other things, the pentose phosphate pathway and the breakdown of caprolactam . The Gulo gene subject, compared to the other genes of the Ascorbinsäurebiosynthese, a much smaller selection pressure. A loss of function has less fatal consequences and is evidently even without negative effects in some organisms. Several vertebrate lineages are negative for L- gulonolactone oxidase. These are all real bony fish (Teleostei), some families of passerine birds (Passeriformes) and bats (Chiroptera), all species from the guinea pig family (Caviidae) and all species belonging to the subordination of the dry-nosed primates (Haplorhini), including that of humans. In the real bony fish, guinea pigs and dry-nosed primates, the genetic defect is so serious that it can be classified as irreversible in evolutionary terms. In contrast, the original gulo pseudogene has apparently been reactivated in some bat and passerine bird species in the course of evolution. According to the current state of knowledge, the food of the species concerned obviously played no role in this “gene reactivation”. It is therefore believed that the loss of the ability to synthesize ascorbic acid is a neutral trait .
Real bony fish (Teleostei)

The terrestrial vertebrates (Tetrapoda) are principally a gulo-positive large group, but contain some taxa - especially among mammals - which also have no functional L- gulonolactone oxidase. These are listed separately below.

Originally it was assumed that fish are generally unable to synthesize ascorbic acid and that this ability first developed in the early terrestrial vertebrates in the course of evolution. Based on extensive research, it is now known that all fish, with the exception of the real bony fish (Teleostei), produce ascorbic acid in their body. They do this with the help of L- gulonolactone oxidase, which is produced in the kidneys of all gulo-positive fish. Ascorbic acid synthesis is an ancestral trait found in vertebrates that was lost to the common ancestor of the teleost animal about 200 to 210 million years ago. The gene loss that causes this trait is apparently complete. Using the BLAST algorithm , the Gulo sequence, or remnants of it, could not be found in any of the fully sequenced genomes of a teleost animal . In comparison, based on the protein sequence of the L- gulonolactone oxidase of the domestic fowl ( Gallus domesticus ), there is a 74% agreement with that of the white sturgeon ( Acipenser transmontanus ) and even with the tubular sea squirt ( Ciona intestinalis ) a 48% agreement. The gulo gene, which codes for L- gulonolactone oxidase, is thus highly conserved across many taxa . The reason why no remnants of the gulo gene are found in the teleostier genome is either that the pseudogene mutated beyond recognition over the 200 million years or that the gene was deleted .
Passerines (Passeriformes)
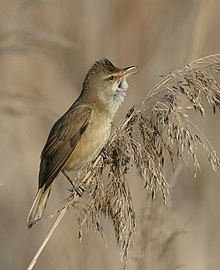

The order of the passerine birds (Passeriformes) is a comparatively young taxon in evolutionary terms . Some species are unable to synthesize ascorbic acid on their own. Others synthesize ascorbic acid in the liver and not, as in many other bird species, in the kidneys. The transition to synthesis in the liver, and the loss of function in some species of passerine birds, is considered by some authors to be "evolutionary progress".
Closer examination of the tribal history makes it clear that those passerines that are unable to synthesize ascorbic acid are not monophyletic . Assuming that the inability to synthesize ascorbic acid is the ancestral condition of the passerine birds, the ability was regained four times in different lineages, and was lost again once (with Terpsiphones ). On the other hand, if one assumes that the ability to synthesize ascorbic acid is the ancestral state, then this ability was lost three times in different lineages and was regained three times.
Bats (chiroptera)
After investigations on the bat species Vesperugo abramus and the fruit bat genus Pteroptus found that they are not able to synthesize ascorbic acid, a total of 34 bat species from 6 different families were examined in detail for this ability in 1976 . After no gulo activity was found in these animals, the premature conclusion in 1976 was that this was generally the case with bats. This assumption had to be revised in 2011: In the case of the flying fox species Rousettus leschenaultii and the bat species Himalayan round-leaf nose ( Hipposideros armiger ), it was initially discovered, surprisingly, that the gulo gene is not a pseudogene in these animals . With a bat-specific polyclonal gulo antibody , L- gulonolactone oxidase could finally be detected in these two bat species - they are therefore able to produce ascorbic acid. Compared to a mouse, the production of L- gulonolactone oxidase is reduced by a factor of six or four. Based on the phylogeny of bats, which is generally accepted today, it can be concluded that the originally inactive gulo gene was reactivated evolutionarily in these two species . In contrast to teleosts, for example, this is possible because the sequence of the gulo gene is very well preserved in both species and differs only slightly from that of gulo-positive mammals. The reactivation of the gene probably only required mutations in areas that are involved in regulating the expression of the gene. The fact that the activity is significantly lower than that of a mouse suggests that further mutations would be required to increase the expression rate. On the other hand, the further evolutionary development of the gulo gene in these two species can also proceed in exactly the opposite direction, namely that it is on the way to a no longer active pseudogene. When Kalong -Flughund ( Pteropus vampyrus ) which is gulo-negative, in the genome of the exons were 3 to 8 as well as 11 and 12 found. The sequence is free of indels and stop codons , so that the gene structure is still largely intact. However, the associated amino acid sequence has eight mutations at positions that are fully conserved in eleven other mammalian species. It is therefore assumed that even if this gene is possibly expressed, the gene product - L- gulonolactone oxidase - is not functional. The state of the gulo gene in the Kalong flying fox is possibly an example of a gene that cannot be reactivated in the course of evolution because too many reverse mutations would be necessary. The changes in the gulo gene of the bats are relatively recent in evolutionary terms. For example, the loss-of-function mutation in the genus Pteropus did not occur until about 3 million years ago.
Guinea pigs (Caviidae)
Guinea pigs are Gulo-negative, and this feature is associated with a special episode of medical history: As early as 1907, the two Norwegian doctors Axel Holst and Theodor Frølich discovered that guinea pigs develop a clinical picture with a certain diet consisting exclusively of grain or bread corresponds to that of scurvy in humans. They succeeded for the first time in specifically transferring the vitamin C deficiency disease to an experimental animal. In addition, they were able to show that the test animals did not become ill with a one-sided diet with white cabbage , carrots or dandelions . If they allowed the fed oats or barley to germinate beforehand, the guinea pigs did not become ill either. If they dried the germinated grain before feeding or heated it to 37 ° C, the anti-scurvy properties were lost again. With their experiments, Holst and Frølich succeeded in proving that scurvy is a deficiency disease . 19 years after Holst and Frølich's experiments, ascorbic acid was discovered by Albert von Szent-Györgyi Nagyrápolt .
By comparative sequence analyzes of the gulo gene of rats, mice and guinea pigs, starting from a point in time of the separation ( divergence time ) of the guinea pig line (corresponds to the large group of porcupine relatives ) from the rat-mouse line (corresponds to the large group of beaver relatives , mouse relatives and flying squirrels ) at about 72 mya , the time of the loss-of-function mutation of the gulo gene in guinea pigs is dated to about 14 mya. The comparatively low age and the type of other mutations in the gulo pseudogene clearly show that this loss of function must have arisen independently of that in other mammals, for example the dry-nosed primates. In the guinea pig gulo pseudogene, exons 1 and 5 were completely lost and exon 6 partially lost, while in the dry-nosed primates seven of the original twelve exons were lost. The nature of the first mutation that led to the loss of function of L- gulonolactone oxidase is still completely unclear in both guinea pigs and dry-nosed primates.
ODS rats and sfx mice
ODS rats ( Osteogenic Disorder Shionogi ) are a mutated strain of albino rats ( Wistar rats ) in which the function of L- gulonolactone oxidase has come to a complete standstill due to a point mutation . A single GA mutation ( guanine versus adenine ) in nucleotide 182 leads to the fact that the amino acid cysteine in position 61 of the L- gulonolactone oxidase is replaced by tyrosine , which leads to the complete loss of function (loss-of-function mutation) of the oxidase Consequence.
In 2000, a strain of mice was first reported to be prone to spontaneous fractures. In these animals, known as sfx mice ( spontaneous bone fractures ), a genetic defect on chromosome 14 was initially found to be the cause. In 2005 it was discovered that it was a deletion of the gulo gene on this chromosome. If sfx mice are given a sufficient amount of vitamin C in their diet, the tendency to spontaneous bone fractures is lost.
ODS rats and sfx mice are used - in addition to guinea pigs - as model organisms , especially for experiments on vitamin C metabolism.
Dry-nosed primates (Haplorhini)
General

Currently (2013) it is assumed that the loss of function of gulo in the dry-nosed primates (Haplorhini) took place around 74 to 61 million years ago, relatively shortly after the line of the dry-nosed primates (old world monkeys, new world monkeys and goblin lemurs) separated from the lemur line (77.5 mya).
Since functionless pseudogenes are not subject to any selection pressure and mutations in these genes have no evolutionary advantage or disadvantage for the organism concerned, they typically have a high mutation rate. Therefore, by comparing identical gene segments, relationships between individual lines of development of the dry-nosed primates can be analyzed. In 1999, a Japanese research group compared a gene segment with 164 base pairs on exon 10 of GuloP in several primate species. The fewer base pairs in this section differ when comparing two species, the more closely they are related to each other. In the case of chimpanzees, humans' closest relatives, the differences to the GuloP gene in humans are actually smallest.
For the US biologist Jerry Coyne , GuloP is one of the most important pieces of evidence for evolution and an argument against so-called “ intelligent design ”. The loss of the function of Gulo and the mutation differences between the primates, which correlate with their degree of relationship, can, in his opinion, only be explained by the evolution and common ancestors of this species. Among other things, Coyne asks why a “designer” would incorporate a mechanism for ascorbic acid synthesis into humans, but then switch it off again by changing one of the genes responsible for it.
"Why would a creator put a pathway for making vitamin C in all these species, and then inactivate it?"
People ( homo sapiens )
GuloP is one of around 80 pseudogenes that have so far been found and characterized in humans. It is located on chromosome 8 gene locus 21.1. GuloP consists of about six exons , none of which code. This means that this gene does not serve as a template for the biosynthesis of a protein corresponding to the genetic code - the enzyme L- gulonolactone oxidase - which is why it is called a pseudogene. In comparison, the fully functional gulo gene in rats consists of twelve exons. The length of the transcript in humans is 748 base pairs. Of the twelve exons in the rat gulo gene, only exons 7, 9, 10 and 12 are found in humans. Exons 8 and 11 are probably deletion . The high number of mutations generally typical of pseudogenes is found in the exons obtained.
Until the 1970s, there was speculation that certain populations - especially the Eskimos - might be able to synthesize ascorbic acid in their bodies. From the daily diet, which at the time consisted almost exclusively of fish and meat, it seemed that the daily requirement for vitamin C could not be covered. Today we know that Eskimos - like all other people - do not have L- gulonolactone oxidase in their organism and consequently cannot synthesize ascorbic acid. The preparation of meat, often raw, but at most only mildly cooked, ensures that the vitamin C contained in it is largely preserved. Today it is assumed that around 15 to 20 mg of vitamin C are absorbed through the daily diet. An amount high enough to prevent scurvy. In addition, there are real vitamin C boosts from eating raw seal or reindeer liver. Consumption of amounts around 100 grams is sufficient to meet the daily need for vitamin C. Of the Eskimos is muktuk (whale skin) much appreciated and this long before you could demonstrate a high content of vitamin C by analysis. Maktaaq contains around 35 mg of vitamin C per 100 grams - a higher concentration of vitamin C than some citrus fruits . All in all, it is assumed that an Eskimo with a traditional diet consumes around 40 mg of vitamin C per day.
Causes for a loss of function
From an evolutionary point of view, only those species could lose the function of L- gulonolactone oxidase if they permanently ingest sufficient amounts of ascorbic acid through their diet. Otherwise a loss-of-function mutation in Gulo would be a significant selection disadvantage. All animal species that are unable to produce ascorbic acid themselves naturally eat rich in vitamin C. This is shown by studies on various species that are gulo-negative. While the recommended daily dose of vitamin C for adults in the United States is 1 mg per kg body weight per day, in the wild, for example, gorillas 20 to 30, coat howler monkeys 88 and Geoffroy's spider monkeys take in 106 mg vitamin C per kg body weight per day . The Jamaican fruit bat ( Artibeus jamaicensis ) even reaches a value of 258 mg / kg / day. Another indication of the lack of selection pressure in Gulo-negative species is that these animals have very different, but always vitamin C-rich diets. Conversely, no gulo-negative species have yet been found that have a low-vitamin C diet, for example through the exclusive consumption of plant seeds. An excess of ascorbic acid through the body's own synthesis, in addition to ascorbic acid, which is ingested through food, does not seem to offer any selection advantage. Supplementing the normal vitamin C-rich diet with vitamin C does not have any positive effects on guinea pigs. The selection pressure in many vertebrates is obviously very small for both loss and recovery of gulo activity.
The two-time Nobel Prize winner Linus Pauling dealt intensively with the question of why some species could lose the ability to synthesize ascorbic acid, even though this is potentially so vital. He put forward the thesis for humans that a direct, early ancestor of humans lived around 25 million years ago in an area in which the diet of this animal species was rich in ascorbic acid. Due to a mutation, the body's ability to synthesize ascorbic acid has been lost. Possibly this happened due to the loss of function of an enzyme. Since sufficient vitamin C was available through the diet, this mutation not only had no negative effects, but on the contrary meant a selection advantage. This resulted from the fact that these mutants no longer had to invest any resources in the construction and operation of the ascorbic acid biosynthesis.
"These mutant animals would, in the environment that provided an ample supply of ascorbic acid have an advantage over the ascorbic-acid-producing animals, in that they had been relieved of the burden of constructing and operating the machinery for producing ascorbic acid."
The energy released by the loss of ascorbic acid synthesis was now available to the affected organisms for other purposes, which gave them an advantage over the non-mutants. With this approach, Pauling largely followed the life history theory and the less-is-more hypothesis. The latter says that genetic losses play an important role in evolution and can mean an evolutionary advantage.
Ascorbic acid regulates the hypoxia-induced factor 1α (HIF-1α) in higher organisms . With increased ascorbic acid levels, the production and activity of HIF-1α is significantly reduced. When activated, HIF-1α upregulates the expression of hundreds of stress genes. From these experimental observations, the hypothesis was developed that organisms that have lost the ability to synthesize ascorbic acid have an evolutionary advantage because they can regulate HIF-1α activity via the exogenous uptake of ascorbic acid. If the supply of ascorbic acid is sufficient, the transcription factor HIF-1α is less active than in the case of an ascorbic acid deficit. In this way the organism is apparently put in a position to recognize the supply status of ascorbic acid. It is known from studies of other pseudogenes that, although they do not deliver gene products (= proteins), they have important epigenetic functions in the expression of other genes. What role GuloP plays in this and whether it offers an evolutionary advantage is still largely unknown.
Another hypothesis assumes that the advantage of ascorbic acid self-sufficiency does not outweigh the disadvantages of ascorbic acid synthesis. In the oxidation of L- gulonolactone catalyzed by L- gulonolactone oxidase, hydrogen peroxide is produced as a by-product. For one molecule of the antioxidant ascorbic acid produced, one molecule of the oxidising agent hydrogen peroxide is produced. This in turn increases the oxidative stress and the need for glutathione in the cells which produce ascorbic acid. Glutathione is - besides ascorbic acid - the most important intracellular antioxidant. Following this hypothesis, with an adequate supply of exogenous ascorbic acid, the loss of L- gulonolactone oxidase activity was an evolutionary advantage. Against this hypothesis, however, the fact that the Gulo gene is mutated back in some species speaks against it . According to the current status (2013), it is more likely that the multiple loss and recovery of ascorbic acid synthesis is accidental, as is to be expected for a neutral characteristic. However, this characteristic is only neutral as long as there is sufficient vitamin C in the diet.
The loss of function of L- gulonolactone oxidase leads to a restriction of the diet. For the dry-nosed monkeys in particular, it is assumed that the loss of function led to further development of sensory abilities, changes in behavior and changes in metabolism in order to better adapt to the necessary diet. This may have led to the development of trichromatic vision in the monkeys , which offers an evolutionary advantage in foraging for food, including the color differentiation of fruits.
Individual evidence
- ↑ a b c d Gerald F. Combs: The Vitamins. 4th edition, Academic Press, 2012, ISBN 0-12-381981-4 , p. 236. Limited preview in Google book search.
- ↑ PL Conklin: Recent advances in the role and biosynthesis of ascorbic acid in plants. In: Plant, Cell and Environment. Volume 24, 2001, pp. 383-394, doi: 10.1046 / j.1365-3040.2001.00686.x .
- ^ RD Hancock, D. McRae et al. a .: Synthesis of L-ascorbic acid in the phloem. In: BMC plant biology. Volume 3, November 2003, p. 7, doi: 10.1186 / 1471-2229-3-7 , PMID 14633288 , PMC 317296 (free full text).
- ↑ a b c d e IB Chatterjee: Evolution and the biosynthesis of ascorbic acid. In: Science. Volume 182, Number 4118, December 1973, pp. 1271-1272, PMID 4752221 .
- ↑ a b c d e D. Glaubitz: Ascorbic acid and ascorbic acid synthesis in invertebrates - a comparative analysis. Dissertation, Free University of Berlin, 2004, pp. 1–20.
- ↑ N. Smirnoff, PL Conklin, FA Loewus: Biosynthesis of ascorbic acid in plants: a renaissance. In: Annual review of plant physiology and plant molecular biology. Volume 52, June 2001, pp. 437-467, doi: 10.1146 / annurev.arplant.52.1.437 , PMID 11337405 .
- ^ GL Wheeler, MA Jones, N. Smirnoff: The biosynthetic pathway of vitamin C in higher plants. In: Nature. Volume 393, Number 6683, May 1998, pp. 365-369, doi: 10.1038 / 30728 , PMID 9620799 .
- ↑ CL Linster, TA Gomez et al. a .: Arabidopsis VTC2 encodes a GDP-L-galactose phosphorylase, the last unknown enzyme in the Smirnoff-Wheeler pathway to ascorbic acid in plants. In: The Journal of biological chemistry. Volume 282, number 26, June 2007, pp. 18879-18885, doi: 10.1074 / jbc.M702094200 , PMID 17462988 , PMC 2556065 (free full text).
- ↑ V. Locato, S. Cimini, LD Gara: Strategies to increase vitamin C in plants: from plant defense perspective to food biofortification. In: Frontiers in plant science. Volume 4, 2013, p. 152, doi: 10.3389 / fpls.2013.00152 , PMID 23734160 , PMC 3660703 (free full text).
- ↑ IB Chatterjee, GC Chatterjee u. a .: Biological synthesis of L-ascorbic acid in animal tissues: conversion of D-glucuronolactone and L-gulonolactone into L-ascorbic acid. In: The Biochemical journal. Volume 76, August 1960, pp. 279-292, PMID 13692610 , PMC 1204705 (free full text).
- ^ John MC Gutteridge, Naoyuki Taniguchi: Experimental Protocols for Reactive Oxygen and Nitrogen Species , 2000, Oxford University Press, ISBN 0-19-850668-6 .
- ^ N. Smirnoff: L-ascorbic acid biosynthesis. In: Vitamins and hormones. Volume 61, 2001, pp. 241-266, PMID 11153268 (review).
- ↑ F. Puskás, L. Braun a. a .: Gulonolactone oxidase activity-dependent intravesicular glutathione oxidation in rat liver microsomes. In: FEBS letters. Volume 430, Number 3, July 1998, pp. 293-296, PMID 9688558 .
- ↑ a b G. Bánhegyi, M. Csala u. a .: Ascorbate synthesis-dependent glutathione consumption in mouse liver. In: FEBS letters. Volume 381, Number 1-2, February 1996, pp 39-41, PMID 8641435 .
- ↑ a b K. Kiuchi, M. Nishikimi, K. Yagi: Purification and characterization of L-gulonolactone oxidase from chicken kidney microsomes. In: Biochemistry. Volume 21, Number 20, September 1982, pp. 5076-5082, PMID 7138847 .
- ↑ M. Nishikimi, BM Tolbert, S. Udenfriend: Purification and characterization of L-gulono-gamma-lactone oxidase from rat and goat liver. In: Archives of biochemistry and biophysics. Volume 175, Number 2, August 1976, pp. 427-435, PMID 822334 .
- ↑ GL Eliceiri, EK Lai, PB McCay: Gulonolactone oxidase. Solubilization, properties, and partial purification. In: J Biol Chem. Volume 244, Number 10, 1969, pp. 2641-2645, PMID 5769996 .
- ^ A b CL Linster, E. Van Schaftingen: Vitamin C. Biosynthesis, recycling and degradation in mammals. In: The FEBS journal. Volume 274, Number 1, January 2007, pp. 1-22, doi: 10.1111 / j.1742-4658.2006.05607.x , PMID 17222174 (review).
- ↑ T. Koshizaka, M. Nishikimi et al. a .: Isolation and sequence analysis of a complementary DNA encoding rat liver L-gulono-gamma-lactone oxidase, a key enzyme for L-ascorbic acid biosynthesis. In: J Biol Chem. Volume 263, Number 4, 1988, pp. 1619-1621, PMID 3338984 .
- ↑ SZ Wong, B. Ching et al. a .: Ascorbic Acid Biosynthesis and Brackish Water Acclimation in the Euryhaline Freshwater White-Rimmed Stingray, Himantura signifer. In: PloS one. Volume 8, number 6, 2013, p. E66691, doi: 10.1371 / journal.pone.0066691 , PMID 23825042 , PMC 3688944 (free full text).
- ↑ SD Gupta, C. Sen Gupta and a .: Enzymic synthesis of L-ascorbic acid from synthetic and biological D-glucurono-1,4-lactone conjugates. In: Analytical biochemistry. Volume 38, Number 1, November 1970, pp. 46-55, PMID 5478251 .
- ↑ EC Birney, R. Jenness, HD Hume: Evolution of an enzyme system: ascorbic acid biosynthesis in monotremes and marsupials. In: evolution. Number 34, 1980, pp. 230-239.
- ↑ a b c d e f g h i j k l m n o p q r G. Drouin, JR Godin, B. Pagé: The genetics of vitamin C loss in vertebrates. In: Current genomics. Volume 12, number 5, August 2011, pp. 371-378, doi: 10.2174 / 138920211796429736 , PMID 22294879 , PMC 3145266 (free full text).
- ↑ a b IB Chatterjee, AK Majumder u. a .: Synthesis and some major functions of vitamin C in animals. In: Annals of the New York Academy of Sciences. 258, September 1975, pp. 24-47, PMID 1106297 (review).
- ↑ a b J. Cui, YH Pan et al. a .: Progressive pseudogenization: vitamin C synthesis and its loss in bats. In: Molecular biology and evolution. Volume 28, Number 2, February 2011, pp. 1025-1031, doi: 10.1093 / molbev / msq286 , PMID 21037206 .
- ↑ L. Hasan, P. Vögeli u. a .: The L-gulono-gamma-lactone oxidase gene (GULO) which is a candidate for vitamin C deficiency in pigs maps to chromosome 14. In: Animal genetics. Volume 30, Number 4, August 1999, pp. 309-312, PMID 10467707 .
- ↑ RH Dadd: Ascorbic Acid and Carotene in the Nutrition of the Desert Locust, Schistocera gregaria Forsk. In: Nature Volume 179, 1957, p. 427, doi: 10.1038 / 179427a0 .
- ↑ G. Niedźwiedzki, P. Szrek u. a .: Tetrapod trackways from the early Middle Devonian period of Poland. In: Nature. Volume 463, number 7277, January 2010, pp. 43-48, doi: 10.1038 / nature08623 , PMID 20054388 .
- ↑ a b A. Nandi, CK Mukhopadhyay u. a .: Evolutionary significance of vitamin C biosynthesis in terrestrial vertebrates. In: Free radical biology & medicine. Volume 22, Number 6, 1997, pp. 1047-1054, PMID 9034244 .
- ↑ AW Bacot, A. Harden: Vitamin Requirements of Drosophila. I Vitamins B and C. In: The Biochemical journal. Volume 16, Number 1, 1922, pp. 148-152, PMID 16743060 , PMC 1259065 (free full text).
- ↑ ES Vanderzant, R. Reiser: Aseptic rearing of the pink bollworm on synthetic media. In: Journal of Economic Entomology. Volume 49, 1956, pp. 7-10.
- ↑ GC Rock: Aseptic rearing of the codling moth on synthetic diets: ascorbic acid and fatty acids requirements. In: Journal of Economic Entomology. Volume 60, 1967, pp. 1002-1005.
- ↑ DE Dykhuizen, KM Harrison, BJ Richardson: Evolutionary implications of ascorbic acid production in the Australian lungfish. In: Experientia. Volume 36, Number 8, August 1980, pp. 945-946, PMID 7439328 .
- ↑ YK Nam, YS Cho et al. a .: Isolation and transient expression of a cDNA encoding L-gulono-gamma-lactone oxidase, a key enzyme for L-ascorbic acid biosynthesis, from the tiger shark Scyliorhinus torazame. In: Aquaculture. Volume 209, 2002, pp. 271-284, doi: 10.1016 / s0044-8486 (01) 00731-1 .
- ↑ DM Fracalassi, ME Allen u. a .: Ascorbic acid biosynthesis in amazonian fishes. In: Aquaculture. Volume 192, 2001, pp. 321-332, doi: 10.1016 / S0044-8486 (00) 00455-5 .
- ^ R. Moreau, K. Dabrowski: Fish acquired ascorbic acid synthesis prior to terrestrial vertebrate emergence. In: Free radical biology & medicine. Volume 25, Number 8, November 1998, pp. 989-990, PMID 9840745 .
- ↑ R. Moreau, K. Dabrowski: Body pool and synthesis of ascorbic acid in adult sea lamprey (Petromyzon marinus): an agnathan fish with gulonolactone oxidase activity. In: PNAS. Volume 95, Number 17, August 1998, pp. 10279-10282, PMID 9707638 , PMC 21499 (free full text).
- ↑ YS Cho, SE Douglas a. a .: Isolation and characterization of cDNA sequences of L-gulono-gamma-lactone oxidase, a key enzyme for biosynthesis of ascorbic acid, from extant primitive fish groups. In: Comparative biochemistry and physiology. Part B, Biochemistry & molecular biology. Volume 147, Number 2, June 2007, pp. 178-190, doi: 10.1016 / j.cbpb.2007.01.001 , PMID 17317254 .
- ^ A b R. Moreau, K. Dabrowski: Biosynthesis of ascorbic acid by extant actinopterygians. In: J Fish Biol. Volume 57, 2000, pp. 733-745, doi: 10.1111 / j.1095-8649.2000.tb00271.x .
- ↑ K. Dabrowski: Primitive Actinopterigian fishes can synthesize ascorbic acid. In: Experientia. Volume 50, number 8, 1994, pp. 745-748, doi: 10.1007 / BF01919376 .
- ↑ a b c MY Lachapelle, G. Drouin: Inactivation dates of the human and guinea pig vitamin C genes. In: Genetica. Volume 139, Number 2, February 2011, pp. 199-207, doi: 10.1007 / s10709-010-9537-x , PMID 21140195 .
- ↑ a b C. Martinez del Rio: Can Passerines Synthesize Vitamin C? In: The Auk. Volume 114, number 3, pp. 513-516, doi: 10.2307 / 4089257 .
- ↑ CR Chaudhuri, IB Chatterjee: L-ascorbic acid synthesis in birds: phylogenetic trend. In: Science. Volume 164, Number 3878, April 1969, pp. 435-436, PMID 5777214 .
- ↑ EC Teeling, MS Springer a. a .: A molecular phylogeny for bats illuminates biogeography and the fossil record. In: Science. Volume 307, number 5709, January 2005, pp. 580-584, doi: 10.1126 / science.1105113 , PMID 15681385 .
- ↑ S. Dutta Gupta, PK Choudhury, IB Chatterjee: Synthesis of l-ascorbic acid from d-glucurono-1,4-lactone conjugates by different species of animals. In: International Journal of Biochemistry. Volume 4, Number 21, 1973, pp. 309-314, doi: 10.1016 / 0020-711X (73) 90053-0 .
- ↑ RN ROY, BC GUHA: Species difference in regard to the biosynthesis of ascorbic acid. In: Nature. Volume 182, Number 4631, August 1958, pp. 319-320, PMID 13577829 .
- ↑ a b EC Birney, R. Jenness, KM Ayaz: Inability of bats to synthesize L-ascorbic acid. In: Nature. Volume 260, Number 5552, April 1976, pp. 626-628, PMID 1264230 .
- ↑ J. Cui, X. Yuan et al. a .: Recent loss of vitamin C biosynthesis ability in bats. In: PloS one. Volume 6, number 11, 2011, p. E27114, doi: 10.1371 / journal.pone.0027114 , PMID 22069493 , PMC 3206078 (free full text).
- ↑ A. Holst, T. Frölich: Experimental studies relating ship beri-beri to scurvy. II. On the etiology of scurvy. In: The Journal of Hygiene. Volume 7, 1907, pp. 634-671, PMID 4606855 .
- ↑ Adolphe-Auguste Lesage, Rudolf Fischl: Textbook of the diseases of the infant. Georg Thieme, 1912, p. 375.
- ↑ H. Schaumann : The Etiology of Beriberi II. Chapter 3: Insufficient food. Archive for Ship and Tropical Hygiene, 1914, p. 125. Restricted preview in the Google book search.
- ↑ Tina König: Development of nutritional research in pigs (until 1930). (PDF; 3.6 MB) Dissertation, University of Veterinary Medicine Hannover, 2004, p. 165.
- ↑ Wolfgang Schaumann: Charles Darwin - Life and Work John Wiley & Sons, 2012, ISBN 3-527-66072-0 , p. 51. Restricted preview in the Google book search.
- ↑ a b PH Fabre, L. Hautier u. a .: A glimpse on the pattern of rodent diversification: a phylogenetic approach. In: BMC Evolutionary Biology. Volume 12, 2012, p. 88, doi: 10.1186 / 1471-2148-12-88 , PMID 22697210 , PMC 3532383 (free full text).
- ↑ a b T. Kawai, M. Nishikimi u. a .: A missense mutation of L-gulono-gamma-lactone oxidase causes the inability of scurvy-prone osteogenic disorder rats to synthesize L-ascorbic acid. In: The Journal of biological chemistry. Volume 267, Number 30, October 1992, pp. 21973-21976, PMID 1400508 .
- ↑ a b M. Nishikimi, R. Fukuyama u. a .: Cloning and chromosomal mapping of the human nonfunctional gene for L-gulono-gamma-lactone oxidase, the enzyme for L-ascorbic acid biosynthesis missing in man. In: The Journal of biological chemistry. Volume 269, Number 18, May 1994, pp. 13685-13688, PMID 8175804 .
- ↑ F. Horio, K. Ozaki et al. a .: Requirement for ascorbic acid in a rat mutant unable to synthesize ascorbic acid. In: The Journal of Nutrition. Volume 115, Number 12, December 1985, pp. 1630-1640, PMID 4067654 .
- ↑ F. Horio, K. Ozaki et al. a .: Ascorbic acid requirement for the induction of microsomal drug-metabolizing enzymes in a rat mutant unable to synthesize ascorbic acid. In: The Journal of Nutrition. Volume 116, Number 11, November 1986, pp. 2278-2289, PMID 3098936 .
- ↑ WG Beamer, CJ Rosen u. a .: Spontaneous fracture (SFX): a mouse genetic model of defective peripubertal bone formation. In: Bone. Volume 27, Number 5, November 2000, pp. 619-626, PMID 11062347 .
- ^ Y. Jiao, X. Li et al. a .: A deletion causing spontaneous fracture identified from a candidate region of mouse chromosome 14. In: Mammalian genome. Volume 16, Number 1, January 2005, pp. 20-31, doi: 10.1007 / s00335-004-2414-0 , PMID 15674730 .
- ↑ a b Y. Jiao, J. Zhang and a .: Differential gene expression between wild-type and Gulo-deficient mice supplied with vitamin C. In: Genetics and molecular biology. Volume 34, Number 3, July 2011, pp. 386-395, doi: 10.1590 / S1415-47572011005000031 , PMID 21931508 , PMC 3168176 (free full text).
- ↑ C. Vergely, F. Goirand et al. a .: Vitamin C deficiency exerts paradoxical cardiovascular effects in osteogenic disorder Shionogi (ODS) rats. In: The Journal of Nutrition. Volume 134, Number 4, April 2004, pp. 729-735, PMID 15051818 .
- ↑ D. Smith, G. Asmundsson et al. a .: The osteogenic disorder Shionogi (ODS) rat: a new model for the study of vitamin C metabolism. In: United States Department of Agriculture - Agricultural Research Service June 1995.
- ↑ Y. Ohta, M. Nishikimi: Random nucleotide substitutions in primate nonfunctional gene for L-gulono-gamma-lactone oxidase, the missing enzyme in L-ascorbic acid biosynthesis. In: Biochimica et Biophysica Acta . Volume 1472, Numbers 1-2, October 1999, pp. 408-411, PMID 10572964 .
- ^ A b Jerry A. Coyne : Why Evolution is True. Oxford University Press, 2009, ISBN 0-19-923084-6 , pp. 72-73. limited preview in Google Book search.
- ^ A b X. Wang, WE Grus, J. Zhang: Gene losses during human origins. In: PLoS biology. Volume 4, number 3, March 2006, p. E52, doi: 10.1371 / journal.pbio.0040052 , PMID 16464126 , PMC 1361800 (free full text).
- ↑ ZD Zhang, A. Frankish u. a .: Identification and analysis of unitary pseudogenes: historic and contemporary gene losses in humans and other primates. In: Genome biology. Volume 11, number 3, 2010, p. R26, doi: 10.1186 / gb-2010-11-3-r26 , PMID 20210993 , PMC 2864566 (free full text).
- ↑ GULOP .
- ↑ Gene database of the National Center for Biotechnology Information (NCBI): GULOP gulonolactone (L-) oxidase, pseudogene (Homo sapiens (human)). Retrieved September 1, 2013.
- ↑ Ensembl : Transcript: GULOP-001 ENST00000454030 Accessed September 1, 2013.
- ↑ L-gulonolactone oxidase. In: Online Mendelian Inheritance in Man . (English).
- ^ RA Gibson, AJ Sinclair: Are Eskimos obligate carnivores? In: Lancet. Volume 1, Number 8229, May 1981, p. 1100, PMID 6112464 .
- ↑ a b Kenneth J. Carpenter: The History of Scurvy and Vitamin C. Cambridge University Press, 1988, ISBN 0-521-34773-4 , p. 231. Restricted preview in Google book search.
- ↑ JR Geraci, TG Smith: Vitamin C in the Diet of Inuit Hunters From Holman, Northwest Territories. (PDF; 288 kB) In: Arctic. Volume 32, Number 2, 1979, pp. 135-139.
- ↑ K. Fediuk, N. Hidiroglou and a .: Vitamin C in Inuit traditional food and women's diets. In: Journal of Food Composition and Analysis. Volume 15, number 3, 2002, pp. 221-235, doi: 10.1006 / jfca.2002.1053 .
- ↑ K. Milton, R. Jenness: Ascorbic acid content of neotropical plant parts available to wild monkeys and bats. In: Experientia. Volume 43, Number 3, March 1987, pp. 339-342, PMID 3104078 .
- ^ JI Pollock, RJ Mullin: Vitamin C biosynthesis in prosimians: evidence for the anthropoid affinity of Tarsius. In: American journal of physical anthropology. Volume 73, Number 1, May 1987, pp. 65-70, doi: 10.1002 / ajpa.1330730106 , PMID 3113259 .
- ^ M. Levine: New concepts in the biology and biochemistry of ascorbic acid. In: The New England Journal of Medicine . Volume 314, Number 14, April 1986, pp. 892-902, doi: 10.1056 / NEJM198604033141407 , PMID 3513016 (review).
- ^ A b Tom Hager: Linus Pauling: And the Chemistry of Life. Oxford University Press, 2000, ISBN 0-19-513972-0 , p. 122. Limited preview in Google Book Search.
- ↑ DA Roff: Contributions of genomics to life-history theory. In: Nat Rev Genet 8, 2007, pp. 116-125, PMID 17230198 (review).
- ↑ NPO: Incarnation: Advantage through loss of genes? At: scinexx on January 14, 2006.
- ^ MV Olson: When less is more: gene loss as an engine of evolutionary change. In: American Journal of Human Genetics . Volume 64, number 1, January 1999, pp. 18-23, doi: 10.1086 / 302219 , PMID 9915938 , PMC 1377697 (free full text) (review).
- ^ HJ Knowles, RR Raval et al. a .: Effect of ascorbate on the activity of hypoxia-inducible factor in cancer cells. In: Cancer Research . Volume 63, Number 8, April 2003, pp. 1764-1768, PMID 12702559 .
- ^ A. Grano, MC De Tullio: Ascorbic acid as a sensor of oxidative stress and a regulator of gene expression: the Yin and Yang of vitamin C. In: Medical hypotheses. Volume 69, Number 4, 2007, pp. 953-954, doi: 10.1016 / j.mehy.2007.02.008 , PMID 17376607 .
- ^ A b MC De Tullio: The Mystery of Vitamin C. In: Nature Education. Volume 3, Number 9, 2010, 48.
- ↑ L. Poliseno, L. Salmena et al. a .: A coding-independent function of gene and pseudogene mRNAs regulates tumor biology. In: Nature. Volume 465, number 7301, June 2010, pp. 1033-1038, doi: 10.1038 / nature09144 , PMID 20577206 , PMC 3206313 (free full text).
- ^ B. Halliwell: Vitamin C and genomic stability. In: Mutation research. Volume 475, Number 1-2, April 2001, pp. 29-35, PMID 11295151 (review).
- ^ Terrence W. Deacon : A Role for Relaxed Selection in the Evolution of the Language Capacity. In: John C. Avise , Francisco J. Ayala (Eds.): In the Light of Evolution IV: The Human Condition. Volume 4, National Academy of Sciences , National Academies Press, 2010, ISBN 0-309-18528-9 , p. 287 limited preview in the Google book search.