Gaia (space probe)
Gaia (space probe) | ||||||||||||||||||||||||||||||||||
---|---|---|---|---|---|---|---|---|---|---|---|---|---|---|---|---|---|---|---|---|---|---|---|---|---|---|---|---|---|---|---|---|---|---|
Gaia space telescope |
||||||||||||||||||||||||||||||||||
NSSDC ID | 2013-074A | |||||||||||||||||||||||||||||||||
Mission goal | Collection of astrometric data | |||||||||||||||||||||||||||||||||
Client |
![]() |
|||||||||||||||||||||||||||||||||
Launcher | Soyuz-ST with Fregat upper stage | |||||||||||||||||||||||||||||||||
construction | ||||||||||||||||||||||||||||||||||
Takeoff mass | 2030 kg | |||||||||||||||||||||||||||||||||
Instruments | ||||||||||||||||||||||||||||||||||
Two telescopes, complex camera with astrometric field, 2 photometers, spectrograph |
||||||||||||||||||||||||||||||||||
Course of the mission | ||||||||||||||||||||||||||||||||||
Start date | December 19, 2013, 9:12 UTC | |||||||||||||||||||||||||||||||||
launch pad | Center Spatial Guyanais , ELS | |||||||||||||||||||||||||||||||||
|
Gaia is a space telescope owned by the European Space Agency (ESA) that performs a high-precision three-dimensional optical survey of the whole sky. Objects with a magnitude of 3 to a magnitude of 20 are recorded, which is why the brightest stars in the night sky such as Sirius or Alpha Centauri are not recorded. Around one percent of the stars in our Milky Way are recorded astrometrically , photometrically and spectroscopically with unprecedented accuracy. In addition to the exact three-dimensional position of each object, repeated observation also determines its direction of movement. For objects with a magnitude of 16 or brighter, the spectrum is also analyzed, from which the radial speed , spectral class , temperature, actual luminosity and other data can be determined.
The Gaia mission is the scientific successor to the Hipparcos mission of the ESA (1989–1993) and should ultimately determine positions up to 200 times more precisely, examine 10,000 times more objects and produce 100,000 times more data than the previous mission. For this purpose, the space probe is in an orbit around the sun-earth Lagrange point L 2 .
The name Gaia was an acronym for " G lobales A strometrisches I nterferometer for A strophysik ". It characterizes the optical interferometry technology originally planned for this telescope . The name was retained despite the change in the measuring principle in the course of planning, but the spelling was changed from GAIA to Gaia . The name is also a reference to the earth mother goddess Gaia in Greek mythology.
ESA confirmed Gaia as a prioritized mission in 2000 and commissioned the construction of the spacecraft in 2006. The probe and payload were built by European companies. The start was on December 19, 2013. Originally the end of the mission was planned for July 25, 2019, but it has been provisionally postponed to the end of 2022.
In September 2016, Gaia DR1 , the first preliminary catalog with more than one billion stars, was published. The second catalog Gaia DR2 from April 25, 2018 contains almost 1.7 billion objects, further improved and expanded catalogs have been announced. All data are accessible to the general public in a database.
Scientific goals
The high-precision measurement of celestial objects requires undisturbed observation. Observations from the earth are subject to many sources of interference, for example vibrations of the telescope from microquakes or distortions due to the refraction of light in the air layers, turbulence in the earth's atmosphere, changes in the measured values due to temperature fluctuations, changes in air pressure, moisture content, observation angles, etc. Only observations from space are possible the desired precision possible.
The positional and parallax accuracy for bright stars ( G-band magnitude <15) will be better than 25 µas (micro arc seconds ) and for the weakest stars (at G = 20) it will drop to around 300 µas, depending on the spectral class. The latter value is still better than the most accurate measurements on very bright stars so far (500 to 2000 µas, carried out as part of the Hipparcos mission). In addition, brightness and colors are to be measured with high accuracy for over a billion objects. For the brightest 100 to 200 million stars up to a magnitude of 16, Gaia should also provide well-resolved spectra from which radial velocity, temperature, surface gravity and chemical composition can be determined.
Astronomers expected the Gaia measurements to reveal a multitude of previously unknown celestial objects. According to estimates in advance of the mission, Gaia should find objects of the following sizes and describe them with data:
- More than 1 billion objects up to a magnitude of 20 are measured in the microsecond range and their magnitude is determined.
- up to a million asteroids and comets within our solar system
- 10,000–50,000 exoplanets outside our solar system
- 50,000 brown dwarfs
- several hundred thousand white dwarfs
- over 20,000 supernovae
- hundreds of thousands of active galaxies and 500,000 quasars
Milky Way

The Gaia mission aims to clarify the origin and development of the Milky Way . For this purpose, Gaia should determine the positions, distances ( parallaxes ) and movements ( proper movements , radial velocities ) of approximately one billion from the more than 100 billion stars of the Milky Way with an accuracy that was previously unattainable ; each star is recorded about 70 times during the nominal duration of the mission. This is an average of 40 million star observations per day. The aim is to gain new knowledge about the distribution of stellar matter, interstellar matter and dark matter from the movements of the stars and their shadowing by dust and gas clouds .
Parallaxes can be determined with Gaia up to the 20th magnitude . For stars up to the 15th magnitude, distances near the center of the Milky Way (8 kpc) can still be determined with an accuracy of about 20 percent.
Star evolution
In addition to information about the structure and development of the Milky Way, it is hoped that these data will provide new insights into the internal structure , formation and development of stars . By determining more precise position data and distances, the absolute luminosity of the individual stars can be determined much more precisely than before. The measurement data collected by Gaia is intended to provide information about where, when and how the stars were formed and how they enrich their surroundings with matter when they die. By determining spectra and directions of movement, groups of stars can be found that have a similar age and a common origin.
Variable stars
The repeated measurements allow a computer-aided acquisition of variable stars such as Cepheids and RR-Lyrae stars with additional data and spectra. In addition, unexpected photometric changes such as B. detected by occultations or by supernovae.
Double stars and multiple stars
The mission is to resolve numerous double and multiple stars and improve their understanding. The vibrations of unresolved double stars that superimpose the parallax measurements and motion measurements can be used to elucidate such systems. Periodic photometric changes are recorded and analyzed.
Exoplanets
The observations allow the recording of exoplanets with masses similar to Jupiter and multi-year orbital times based on the movements of the star around the common barycenter, including determination of the masses. The transition area between large exoplanets and brown dwarfs is determined more precisely.
Solar system objects
Gaia detects all objects in the sky, except those with a very high apparent speed of more than 15 mas / s. Movements are mainly recorded in the scanning direction. Strong movements across the scanning direction cause the object to wander out of the scan window during the exposure period and to be discarded as a faulty measurement. However, since Gaia scans in different directions during the course of the mission, it is possible to capture the same object at a more favorable angle. The comparatively strong movement of objects in the solar system ( Solar System Objects , SSO) causes them to appear elongated rather than punctiform.
Main belt asteroids
The planets of the solar system are too big and mostly too bright to be seen by Gaia. Asteroids and comets are well suited to capture, and Gaia will be very precise orbital data for many of these objects. Gaia will also find new objects that are far from the plane of the ecliptic or within the earth's orbit. The Gaia project issues messages ("Science Alerts") so that the objects found can be followed up by earth-based observations and enough observations are made so that the orbit data can be calculated precisely enough and the objects are not lost again. For objects that approach each other during the observation phase, Gaia performs mass calculations. Most of the objects found are expected to be main belt asteroids.
Objects close to the earth
In total, several thousand near-earth objects (NEOs) are expected to be measured, both asteroids and comets. Since Gaia records objects from a different perspective than from the earth, some objects can also be measured that are hardly observable from the earth because they are outshone by the sun. Gaia is also supposed to find objects within Earth orbit. The measurement of orbital disturbances will enable the calculation of the mass of approximately 150 asteroids.
Kuiper belt objects
Most of the objects in the Kuiper Belt are too faint to detect, but the largest objects are found. In 2009 around 800 objects were known in the Kuiper Belt. Trans-Neptunian objects and centaurs are generally very faint, so only about 65 of them are known to be brighter than 20 mag and 138 are known to be brighter than 21 mag. Accordingly, Gaia can only find a few new of these objects, but also those in the direction of the Milky Way that are difficult to discover or that have a great inclination and are far from the ecliptic. Gaia also recognizes binary objects among these .
Local group
Gaia's ability to resolve is sufficient to capture the brightest stars in the local group . A number of neighboring galaxies of the Milky Way can be registered in this way, such as the Andromeda Galaxy and the Magellanic Clouds . For distant dwarf galaxies there will be only a few of the brightest stars, for the neighboring galaxies there will be thousands to millions of stars. Globular clusters and dwarf galaxies such as Fornax , Sculptor and Sextans are captured with thousands of stars. The interaction of galaxies is being explored, in particular how the Milky Way interacts with the Magellanic Clouds. Even star movements within dwarf galaxies can be recorded. All of these observations are suitable for determining the influence of dark matter on star movements.
Unresolved galaxies and quasars
Gaia is supposed to record millions of distant galaxies and obtain photometric data from them. The quasars found should serve as orientation points for optical and radio astronomical reference systems.
Astrophysics and basic research
A possible change in the gravitational constant G over time (more precisely:) should be recorded with an accuracy of better than 10 −13 / year. The relativistic deflection of light by the force of gravity of the sun is to be measured with a relative accuracy of around a millionth and the deflection of light by the force of gravity of the planets is to be detected directly for the first time.
Gaia could also provide information about the distribution of dark matter in the galaxy, for example there are speculations ( Lisa Randall ) that there could be a concentration in the galactic plane.
costs
The costs for the entire mission from the preliminary studies on take-off, ground control and payload to the nominal end of the mission in July 2019 are estimated at 740 million euros. The probe itself cost 450 million euros. Not included are costs of around 250 million euros for the DPAC consortium , which operates the scientific data reduction . These costs are borne by the participating countries and institutes, not by ESA. Members of the DPAC come from 20 European countries: Belgium, Denmark, Germany, Estonia, Finland, France, Greece, Ireland, Italy, the Netherlands, Austria, Poland, Portugal, Slovenia, Spain, Switzerland, Sweden, the Czech Republic, Hungary, the United Kingdom, also from Algeria, Brazil, Israel and the United States. However, ESA is making a significant contribution to DPAC by providing the central data and computing center ESAC in Villafranca del Castillo, near Madrid.
Industrial participation
In February 2006, ESA commissioned EADS Astrium , now part of Airbus Defense and Space , to build Gaia. On May 11, 2006, the construction contract for Gaia was signed between ESA and Astrium.
Astrium was chosen as general contractor for the development and construction of the probe and the payload. The payload was built under the responsibility of Airbus DS in Toulouse , the mechanical service module by Airbus DS in Friedrichshafen and the electrical service module by Airbus DS in Stevenage . There were around 80 contracts with 50 companies from 15 European countries; three were completed with companies in the United States. About 2500 to 3000 people were involved in the project.
Spacecraft

The probe consists of three main parts: sun shield, supply unit and payload . A cylindrical structure is located above the sun shield, which houses the supply unit and the payload.
Sun shield
The almost circular arrangement of solar cells and “parasol” dominates the hat-like appearance of Gaia. The sun shield consists of twelve broad struts, between which 48 triangular areas spanned when the shield was unfolded. The probe is 3-axis stabilized and will use its slow rotation to continuously scan the sky moving through the field of view. The payload and supply unit are always in the cooling shadow of the “parasol” during scientific operation. When the sun shield is unfolded, Gaia has a diameter of 10 m.
Gaia is passively cooled, there are no radiators or cooling elements. Gaia only cools down through heat radiation to such an extent that it is in equilibrium with the general ambient radiation. The average solar radiation is approx. 1361 W / m². October 2014 sunspots caused a measurable reduction of solar radiation by about 3 W / m² and a cooling of the sun shield to 0.15 ° C . Seasonal changes in the distance from the sun lead to a change in solar radiation of ± 3.4% and a temperature change of ± 1.2 ° C; the daily rotation causes a periodic temperature change of 2 ° C in the area of the antenna. On November 6, 2015, a passage through the moon caused a cooling of about 1.5 ° C for about 10 hours. The thermal inertia of the system and the insulation measures mean that a passage through the moon has no noticeable effect on the temperatures of the measuring instruments.
Supply unit
The supply unit is located between the sun shield and the payload and contains essential components such as drive units, position control, fuel tanks, power supply and cabling, atomic clock , video processors , computer, data storage and data transmission system. In order to keep the probe stable and free from vibrations, it has no moving parts with the exception of the valves for the engines. The components of the supply unit are mounted on a frame made of carbon fiber reinforced plastic . Parts of the payload, mainly the electronic components, require a certain minimum temperature and are therefore housed in the area of the supply unit. The supply unit has a diameter of 3 m and a height of 1 m.
payload
The payload has a diameter of approximately 3 m and a height of 2 m. The mirrors for the telescopes and the camera are attached to a ring-shaped structure, the “torus”; all of these components together essentially form the payload. The torus consists of ceramic-like silicon carbide , was assembled from 17 individual parts and soldered to a special material at 1000 ° C. Silicon carbide is very strong, very hard, light and has very low thermal expansion and high thermal conductivity. The payload is operated at a temperature of around −110 ° C and is located in a tent made of carbon fiber reinforced plastic and aluminum sandwich panels , which should keep the temperature inside as constant as possible and at the same time protect the mirrors and the camera against micrometeorites , particles and radiation . The tent has two openings for the telescopes.
Power supply
The probe has 12.8 m² high-efficiency solar panels made of three-layer gallium arsenide cells. 7.3 m² are permanently installed, 5.5 m² are attached to the sun shield and unfolded together with it. These modules were folded up during take-off. A 60 Ah lithium-ion battery was used for the start-up phase and initial operation. There is a current control that ensures that the maximum power consumption is not exceeded at any time. Since the probe is operated continuously at a 45 ° angle to the sun, the solar modules cannot achieve their full performance in normal operation. Gaia has a total power consumption of 1720 W, of which the payload consumes approximately 830 W.
communication
Gaia only communicates via the X-band . There are two omnidirectional low gain antennas with a transmission rate of a few kbps only for telemetry data on opposite sides of the X-axis. They can transmit and receive from any position and are used for the start-up phase and emergency communication if for any reason the main antenna cannot be used.
A conventional high-gain parabolic antenna would be unsuitable as the main antenna for data transmission to earth, since the axis of rotation does not point to earth during the observation phases and a movable antenna would affect the measurement results due to vibrations. Instead, the scientific telemetry data is transmitted through a high-gain phased array antenna (PAA), which is attached to the bottom of the service module on the hot side of the sun shield. The shape corresponds to a hollow fourteen-sided truncated pyramid, which achieves an antenna gain of 16.8 dB . Each of these fourteen side surfaces has two arrays, each consisting of six radiators. These radiators are operated with 28 solid-state amplifiers with an output of 59 watts (≈ 17.7 dBW ). Each of these sub-units divides the signal in such a way that the phase shift composed of all 14 sub-units causes a corresponding total emission. The total isotropic radiation is greater than 32 dBW for the largest range of the radiation angle of 30 degrees. This allows a data rate of 8.7 megabits per second for transmission to the ground station. The gross data rate is 10 megabits per second; Part of this is used for error correction . The antenna is also used for tracking with the radio telescopes of the ground stations and for orbit reconstruction measurements from the ground.
Positions of the three deep space antennas (DSA) of the ESA's ESTRACK network |
Via the phased array antenna, Gaia can communicate with each of the three deep space antennas of the ESTRACK network for about 8 to 11 hours per day , as long as the probe is in the field of view of the ground station. For the mission, the reception capacities of the three 35 meter antennas were expanded in advance. The average downlink time is approximately 8 to 14 hours per day; thus Gaia uses the network the most of all missions.
Engines and attitude control
Gaia has two different systems for propulsion and attitude control.
- Gaia has two times eight chemical thrusters with 10 Newtons of thrust each , in order to be able to enter the Lissajous orbit around L 2 and to periodically correct the orbit. These form the Chemical Propulsion Subsystem (CPS) and use the two components dinitrogen tetroxide (NTO) and methylhydrazine (MMH).
- The measuring operation requires an extremely precise control of the position and the speed of rotation. For fine control during the measurement operation, Gaia has two times six cold gas nozzles with very small variable thrust of 10 to 150 micronewtons on board. These form the Micro Propulsion Subsystem (MPS) and use nitrogen as the compressed gas.
At the start, the probe carried around 400 kg of chemical propellants and two tanks each with 28.5 kg of nitrogen under a pressure of 310 bar.
There are no moving parts such as reaction wheels or gyroscopes for position control . Two independent A-STR star sensors are available for navigation in the cold area (facing away from the sun) and three sun sensors in the hot area, plus three redundant fiber optic gyros . The speeds at which the stars move over the focussing plane provide further data on the speed of rotation and the position in space. In science operations, only the star sensors are used in conjunction with the data from the camera to determine the exact position. The other sensors are only used for error detection and correction.
The system can handle unexpected changes in position, e.g. B. by a micrometeorite impact, compensate automatically within a very short time. Gaia can automatically switch between different forms of attitude control and between the two types of propulsion, thereby optimizing the use of engines and fuel.
Instruments
Telescopes
Gaia carries three main scientific instruments, which are jointly supplied by two reflecting telescopes with widely separated fields of view in the sky. The three-mirror Korsch telescopes are built as a Schiefspiegler and have rectangular, 38 kg concave primary mirrors measuring 145 cm × 50 cm (M1, M'1). The convex secondary mirrors (M2, M'2) are 35 cm × 16 cm in size. From there, the light hits the two concave tertiary mirrors (M3, M'3) measuring 65 cm × 28 cm. These direct the light onto the two flat combiner mirrors M4 and M'4 measuring 19 cm × 7 cm, which combine the light from both telescopes and pass it on to M5. From there it reaches the focussing plane via M6. M5 and M6 are flat and have a size of 55 cm × 34 cm.
All ten mirrors are made of sintered silicon carbide and have a highly reflective, protected silver surface. The telescopes have a focal length of 35 m. The two secondary mirrors have actuators that can move the mirrors in a range of 5 °, but these were only in operation during the calibration phase and after a heating period. All instruments look at the same sky sections separated by 106.5 °, which are each depicted by one of the two telescopes. The two fields of view are around 1.4 ° × 0.7 °, so they cover around four times the area of the sun or full moon disk in the sky.
The mirror blanks come from Boostec from Bazet in France. Sintered silicon carbide does not produce smooth surfaces when ground; After the preliminary grinding, the mirrors were therefore coated with an additional layer of silicon carbide by Schunk Kohlenstofftechnik in Heuchelheim in a special process. Silicon carbide has a Mohs hardness of 9.6, which is close to that of diamond; the final processing was accordingly very time-consuming. The mirrors were ground to a precision of 10 nm, and the mirrors on both sides had to be very similar. The two primary mirrors were ground by Sagem near Paris, the two M2 and M4 as well as M5 and M6 mirrors were machined by AMOS in Liège , Belgium, and the two M3 mirrors by Carl Zeiss Optronics in Oberkochen , Germany. The mirrors were eventually coated with silver by Sagem, with the exception of the two M3 mirrors, which were completed by Zeiss.
camera
The light from both telescopes falls on a common focusing plane. There, the objects are recorded together by a field of 106 highly sensitive CCD detectors with dimensions of 6 cm × 4.7 cm and a resolution of 4500 × 1966 pixels each . Together, the sensors have around a billion pixels; this is the highest resolution camera ever operated in space. The CCDs record wavelengths of 330–1050 nm, that is, in addition, a range in the UV and infrared that goes beyond the perception of approx. 400–760 nm of the human eye. The CCDs are mounted on a 20 kg plate made of silicon carbide and measuring 1.0 m × 0.5 m.
Astrometry
The two rows of 7 Skymapper CCDs each recognize which object comes from which telescope. The Skymapper CCDs are read out completely, objects are recognized, classified according to brightness and the size of the observation window is determined. During the initial processing of the CCD data, a strong data reduction is carried out by ignoring black pixels, non-punctiform or faint objects with a magnitude of greater than 20.7 during further processing. The relevant objects are cut out as a mini image with a few surrounding pixels, their position is noted, given a time stamp and processed individually. Each object recognized in this way must be confirmed in the first astrometric field AF1 during the next measurement. If no object is recognized there at the corresponding point, the object is discarded. In this way, artifacts from space radiation and charged particles are sorted out at an early stage.
A field of 62 of these CCD detectors in a 7 × 9 grid registers the celestial objects several times. The detector field records the star positions in the sky with a precision of sometimes better than 30 µas (micro- arc seconds ). Taking all measurements into account, a parallax error is expected for an object at the end of the mission. B. for a star of the class M6V with a brightness of 15 mag is 9 µas. The accuracy should be 20 to 50 times higher than the previous Hipparcos mission. (See also: Problems section .) The distance can be determined by measuring the parallax . Through the multiple measurements of stars during the life of the probe, the angular speeds of star movements can be derived.
The instrument measures the G-band magnitude in the wave range between 330 and 1050 nm, in which on the one hand the mirrors reflect and on the other hand the sensors are sensitive. The observed brightness together with the calculated distance and the spectroscopically determined spectral shift enables the actual luminosity of an object to be determined.
Two CCDs (BAM) are used to constantly measure the basic angle of 106.5 ° between the two telescopes. Two laser beams work as an interferometer. A laser serves as a backup and to confirm that it is working correctly. Knowledge of minimal deviations from the basic angle is necessary for an exact position determination. The two sensors were also used to measure radiation. The two wave front sensors should monitor the optical quality of the two telescopes and ensure that both telescopes are optimally focused.
Photometry
Gaia has two photometers. 14 CCD detectors in two rows measure brightness and colors in a wide range of wavelengths. The first row of seven CCD detectors for the blue photometer (BP) uses a prism and covers the blue wavelength range 330 to 680 nm. The second row of CCD detectors for the red photometer (RP) uses a different prism and covers the red wavelength range from 640 to 1050 nm. The spectral resolution is between 15 nm and 60 nm and is comparatively low, so that one does not usually speak of “spectra” here, but rather of spectrophotometry .
The central task of these photometric measurements is to be able to characterize each and every one of the billion stars observed by Gaia. i.e. to measure its temperature , surface gravity and metallicity . These properties of the stars (in addition to their position, distance and speed) are important to e.g. B. To draw conclusions about the history of star formation in the Milky Way. In addition, photometry can be used to distinguish stars from other celestial objects such as asteroids, galaxies or quasars, which are also observed by Gaia. Photometric data are necessary for a color-corrected calculation of the brightness and to correct the other measurements. By shifting the spectrum to red or blue, an object can have a different brightness value because part of the spectrum is outside the sensitivity range of the sensors.
Since the spectra take up a relatively large area on the sensor, not all objects can be measured photometrically with every transit. Overlaying several objects plays a bigger role, so that generally not every observation can be assigned a photometry. There is a procedure to ensure that overlays are handled appropriately.
Spectroscopy
The radial velocity spectrometer (RVS) uses the same combined field of view as the astrometric and photometric instruments. It works with twelve CCD detectors that record the line spectra of the stars, from which the speeds of the stars along the line of sight can be derived. Together with the photometer, an exact classification of many of the observed objects will be possible. The measurement of the radial velocities of many stars is necessary for the achievement of the scientific goals of the Gaia mission. Only with such measured radial velocities can models of the gravitational potential of our Milky Way or the history of star formation be experimentally restricted.
At 11500, the radial velocity spectrometer has a far higher spectral resolution than the photometer, but only covers a small wavelength range from 845 to 872 nm. In this wavelength range, stars show characteristic absorption lines of the so-called calcium triplet in the range of 849.8, 854.2 and 866.2 nm. The high resolution makes it possible to measure the wavelengths of these three calcium absorption lines, so that a comparison of the wavelengths with Laboratory values allow the radial velocity of the star to be determined using the Doppler effect . The triplet can also be evaluated for classification in the spectral class .
This spectroscopic measurement is possible for objects that are brighter than a magnitude of 17. Due to the high resolution, the radial velocity spectrometer is limited to the brighter stars. It is likely that around 50 to 100 million stars are bright enough for the radial velocity spectrometer, whereas photometry can measure almost all objects with a magnitude <21 mag due to its higher sensitivity. Nevertheless, Gaia's radial velocity spectrometer with many millions of stellar spectra will deliver by far the largest catalog of stellar spectra currently available.
To calibrate the spectrometry and photometry, most of the previously common comparison stars were z. B. the pole sequence unsuitable because they are too bright; some even turned out to be double stars. For the mission, therefore, new, fainter comparison stars of different spectral types were sought. 1420 stars with well-known radial velocities made up a preliminary list of comparative stars, the Gaia Spectrophotometric Standard Star Catalog (SPSS) . It is possible that as observation progresses, some of them will be deleted. The final list of comparison stars will only be available at the end of the mission.
Probes own data processing
The observations require very precise time recording; this is achieved by an on-board, highly stable 10 MHz rubidium atomic clock . Each measurement is given an exact time stamp. The CCSDS standards for time recording were extended to the picosecond range especially for this mission. The data is processed by a computer system with a modular architecture that corresponds to the arrangement of the detectors. For data acquisition, the data processing system has seven video processing units (VPU), one unit for each row of detectors. Each VPU contains a special CWICOM preprocessor (CCSDS Wavelet Image COMpression ASIC) developed by Astrium, which is mainly responsible for the rapid compression of the data and the generation of the data packets. A SCS750 PowerPC board from Maxwell Technologies in San Diego, USA, is responsible for the majority of the processing . Each board has three PowerPC-750FX processors working in parallel , the calculation results of which are constantly compared via a logic for automatic error correction. In this case, the radiation-hardened variant of the processor was not used for performance reasons. Errors caused by space radiation are automatically corrected and a faulty processor is restarted within 1 ms without affecting running programs. Each of the seven VPUs has a computing capacity of 1000 MIPS . All data is processed in real time without intermediate storage, and the sensors are synchronized and read out at exactly the same speed as the objects move over the detectors. The failure of one of the seven video processing units would have little effect on the results. At the beginning of the mission there were frequent resets of the VPU, with an update of the VPU software to version 2.8 in April 2015 the problem was fixed.
A shared, separately operated 800 Gbit solid-state mass storage device is used to store the results . After subtracting the bits for the Reed-Solomon error correction , the effective storage capacity is 120 gigabytes. On average, 40 million objects are observed every day, 400 to 500 million measurements are carried out and 40 GB of data are produced. Measurements in the galactic plane recorded 270 million objects and 3 billion measurements on February 28, 2015. The probe's own computer systems are the most powerful to date that have ever been used in space.
By December 31, 2018, the 1620th day since the start of scientific data collection on July 25, 2014, 116,023,842,167 objects had been recorded by the sensors, of which there were 1,143,663,587,072 astrometric measurements by the 62 astrometric and the 14th Skymapper CCDs. There were 231,915,375,586 photometric measurements by the 14 blue and red photometer CCDs. The RVS instrument for calculating the radial velocity recorded 22,300,583,616 spectra and 7,432,109,980 objects. An object in the sense of the data processing of the Gaia mission is defined as a window several pixels in size and in densely populated regions of the sky can also contain several light sources such as e.g. B. contain stars, quasars and asteroids.
Start and test phase

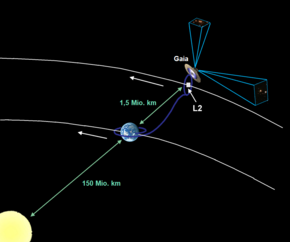
begin
The launch took place on December 19, 2013 at 9:12 a.m. UTC with a four-stage Russian Soyuz ST rocket with a Fregat upper stage from the Center Spatial Guyanais in French Guiana . Originally planned for November 20, 2013, it has been postponed for technical reasons. The launch mass of the probe was 2030 kg, of which 710 kg for the payload, 920 kg for the service module and 400 kg of fuel.
The rocket reached an orbit at an altitude of 175 km using the Fregat stage. Eleven minutes later, the Fregat stage was re-ignited and put the probe on a transfer orbit. The Fregat was disconnected 42 minutes after taking off, and the sunshield was extended after just under 90 minutes.
Test phase
On January 8, 2014, Gaia reached its orbit around the Sun-Earth Lagrange point L 2 . The L 2 point is about four times the lunar distance from the sun and about 1.5 million km behind the earth. This gravitational equilibrium point runs at a fixed distance with the earth around the sun and enables a more undisturbed view of the universe than from a lower earth orbit. Gaia took a Lissajous orbit at a distance of 263,000 km × 707,000 km × 370,000 km around L 2 to ensure that it would not enter the penumbra of the earth for at least six years . The latter would impair the energy supply and temporarily reduce the image quality due to the thermal expansion of the optical components when the temperature changes. The probe was extensively tested for about half a year during the commissioning phase, as was the data transmission, data processing and position determination.
calibration
The test phase ended on July 18, 2014. This was followed by a calibration phase of 28 days, during which the ecliptic poles were intensively measured, and the collection of scientific data began at the same time. During this time, Gaia was operated in Ecliptic Poles Scan Law mode (EPSL), in which the two poles were measured twice with each revolution. The Ecliptic Pole Catalog (EPC, later Gaia Ecliptic Pole Catalog , GEPC) was created to measure the poles . The GEPC-V.-3.0 catalog contains 612,946 objects from a field of one square degree each at the north and south poles. The northern pole is relatively poor in stars and contains 164,468 objects, while the southern pole is still in the area of the Large Magellanic Cloud and contains 448,478 objects.
Nominal operation
Following the calibration, the measurements were extended to the entire sky. Since then, Gaia has been in the Nominal Scanning Law (NSL), in the regular "scan mode". About once a month the hydrazine engines had to correct the course of the probe. The interval between corrective maneuvers has been extended to three to four months over time.
Ground control
The ground control (Mission Operations Center, MOC) is located at the European Space Control Center (ESOC) in Darmstadt . The ground control uses only the telemetry data and is responsible for tracking the exact location and any course corrections. It is also responsible for planning and allocating the corresponding download capacities of the ESTRACK network, in consideration of the needs of other simultaneously running missions. The data from all three antennas come together at the ground control and are forwarded from there to the European Space Astronomy Center (ESAC). The ESOC also processes the data from the Ground-Based Optical Tracking Unit (GBOT) to determine the position and will perform the reconstruction of the probe position, which forms the basis for the precise calculations of the object positions.
Scientific operation
While ESOC controls the spacecraft and controls communication, all scientific control lies with the Science Operation Center (SOC). All evaluations of the scientific data as well as the scientific operations, the storage, management and distribution of the data are carried out with the help of the Spanish ground station in Cebreros and the ESAC in Villafranca.
Observation strategy
The observation is made from a monitored Lissajous orbit around the Lagrange point L 2 . During the observation phase, the probe rotates continuously with high precision at a constant speed around its own axis, the speed of rotation being synchronized with the readout speed of the sensors. In six hours, the satellite with its two observation fields records objects in a narrow strip of sky of 360 ° at right angles to the axis of rotation. Since the two observation fields are 106.5 ° apart, an object passes through both observation fields one after the other at a distance of 106.5 minutes. The individual sensors are swept over in a time of 4.4 seconds, and this time is also the exposure time. The axis of rotation does not point in a fixed direction in space, but moves very slowly in a circular motion and describes a circle in 63 days, so that the observation strip moves on and the entire sky is scanned. During the entire observation period, the probe is at an angle of 45 ° with the sun shield facing the sun. Objects must be smaller than approximately 500 to 600 mas in diameter to be recorded, which excludes the planets and some of their moons as well as some asteroids from the detection.
Ground-Based Optical Tracking Unit
For exact calculations, the position of the probe must be known very precisely at all times, in particular the length of the baseline for the parallax measurement must be known. The absolute speed in relation to the barycenter of the solar system must be known up to 2.5 mm / s and the absolute position accurate to 150 m. A very precise measurement method is the Delta-DOR method , which requires two antennas that are far apart. Delta DOR can determine the position at this distance to within 22 meters. However, it is not possible to make two of the antennas of the ESTRACK network available for the entire observation time, because other missions also have to access the antennas from time to time, and only a small part of the sky can be observed by two antennas overlapping. A radar measurement from an antenna alone gives an accuracy of 2000 m in position and 10 mm / s in speed as well as 75 m and 1 mm / s radially.

(VST at the far end of the mountain plateau)
This problem is solved by the Ground-Based Optical Tracking Unit (GBOT): During the entire mission time, optical telescopes regularly look at the probe and record its position and time so that the exact position of the probe can be calculated for any point in time can. This method has been successfully before the start of the much smaller WMAP probe and the Planck Space Telescope tested, both at L 2 operated. The position is evaluated relative to the reference stars. Since their positions, parallaxes and movements are only known more precisely after observation and evaluation, the position determination is repeated recursively with improved data : The more precise position determination of the probe in turn improves the accuracy of the position measurements of the reference stars and so on. GBOT cannot observe the probe in a period of five to seven days during a full moon, since the moon is in the direction of L 2 from Earth during this time and outshines Gaia. During this time, Delta-DOR measurements can compensate for the gaps so that there is no loss in the data quality of the position data. ESOC evaluates both the radar measurements and the observations from GBOT for orbit reconstruction.
Tracking telescopes include the 2.5 m VLT Survey Telescope (VST) on the Paranal in Chile, the 2 m Liverpool telescope on Roque de los Muchachos , La Palma, Spain, and the 2- Faulkes North and South m telescopes of the Haleakalā Observatory on Maui Island (Hawaii, USA) and the Siding Spring Observatory in Australia. These telescopes are partially automated. Some of them are also involved in the "Gaia Follow-up Network for Solar System Objects" (Gaia-FUN-SSO) in tracking the orbits of newly found objects in the solar system.
Gary Whitehead maneuver
On July 16, 2019, one day after the end of its nominal operating time, the probe was put into another orbit with the largest corrective maneuver since the launch phase. If the previous course had been maintained, the probe would have entered the earth's shadow in August and September 2019. In this case, the power supply and thus communication and research would have been interrupted. The resulting temperature changes would have had negative effects on the scientific community for several weeks. This maneuver was named after Gary Whitehead, a member of the control team who died recently. The maneuver used a special combination of control nozzles in which the orientation of the probe to the sun remains the same at all times, so that the sunlight does not fall on the cold part of the probe or the sensitive telescopes and the solar cells maintain their orientation. At the same time, this opportunity was used for extensive tests of various systems and for calibrations that would otherwise have interrupted scientific operations. In total, the control nozzles were fired nine times for a total speed change of 14 m / s. In the new orbit, the probe will not enter Earth's shadow until 2026. The corrective maneuver dragged on for a whole day so that the fuel could be evenly distributed in the tanks over and over again, using 10 kg of fuel. Following the maneuver, Gaia will be operated under reverse precession scanning law for one year . The axis of rotation precesses in the opposite direction, which improves the condition of the astrometric solution.
By July 16, 2019, the 1817th day and the end of the nominal mission duration, 129,705,110,100 objects were recorded by the sensors, there were 1,278,521,799,553 astrometric measurements by the 62 astrometric and the 14 Skymapper CCDs. There were 258,759,786,958 photometric measurements by the 14 blue and red photometer CCDs. The RVS instrument for calculating the radial velocity recorded 25,125,452,190 spectra and 8,394,259,584 objects.
Mission extension, fuel supplies and mission end
The fuel carried extends beyond the nominal mission duration of 5 years + ½ year for the test phase. The planned nominal mission lasted until July 25, 2019. On November 22, 2017, the Science Program Committee (SPC) of ESA decided to continue operating Gaia beyond the nominal mission time until the end of 2020. In accordance with ESA's rules for science missions this extension was confirmed on March 20, 2018. On November 14, 2018, the further extension until 2022 was announced.
Mission extensions are always subject to funding by the ESA or the approval of the budget by the members involved.
In the event that the entry into the Lissajous orbit at the Lagrange point L 2 would not have taken place correctly, the probe has additional fuel for subsequent correction in order to be able to reach L 2 anyway. The chemical fuels could thus stabilize the probe at the L 2 point for decades , but it is expected that the nitrogen supplies for the cold gas engines will only last for 10 ± 1 years .
After the end of the mission, Gaia will leave the Lissajous orbit around the Earth-Sun-Lagrange point L 2 and turn into a stable Kepler orbit around the sun, this also applies in the event that the fuel is exhausted or the probe can no longer control itself leaves.
Technical limits
Limited computing capacity
Heavily populated sections of the sky such as neighboring galaxies or the densest areas of the Milky Way such as Baade's Window with a large number of objects in a small space pose a problem for internal data processing. Although the probe's own computing units have a high capacity, the number of processable objects per unit of time. Lighter objects are automatically prioritized so that the data from objects with less light is lost. However, Gaia will examine these regions several times with different specifications and each time record additional newly discovered objects. There is a limit of 1,050,000 objects per square degree for the densest areas.
Limited downlink capacity
Gaia produces a variable amount of data that must be received by the European 35 m antenna network ESTRACK . Of all missions, Gaia places the highest demands on the antenna network. Regions with few stars produce less data, regions with many stars produce more data. The daily workload is planned in advance in order to make optimal use of the antenna time required. The ESA expanded the reception capacities of the systems to up to 8.7 Mbit / s, but all three antennas are not sufficient if particularly densely populated sections have to be evaluated. At some times, for example when the field of view is close to the galactic plane, the amount of data even exceeds the amount that can be received by all three stations together. Since the sky is scanned several times, an intelligent data grid decides which of the less significant data is deleted.
Saturated sensors
For objects that are lighter than a magnitude of 3, the sensors can no longer output precise values. The number of these very bright objects is comparatively small. There are other ways to get the data you need for these objects so that the final star catalog will be complete for these objects too. The Skymapper CCDs are less sensitive so that this data can be used for the evaluation of bright objects. Objects with a magnitude of 6 or brighter generally have higher measurement uncertainties.
Radiation damage to the sensors
The sensors are subject to inevitable aging due to cosmic rays . Particles can cause permanent damage when they hit the sensors, which can be seen in the form of hot pixels or complete failure of individual pixels or entire rows of pixels. It is possible to detect defective pixels and exclude them from data processing. The values of these pixels are ignored and there is enough redundancy so that correct functioning can be maintained for the planned life of the probe. Most of the particles of the solar wind can be kept away from the sun shield, the remaining parts are high-energy galactic or extragalactic particles. Contrary to original fears, in reality the problem was roughly ten times lower than expected.
Problems
All of the probe's systems are working and the quality of the scientific data is in line with expectations. However, there were some minor glitches and restrictions common to such complex space missions.
Stray light
Shortly after the start, the scientists involved discovered stray light problems on Gaia. Light from the sun found a detour into the telescope's optics. After ice deposits on the edge of the sun shield were initially suspected, it turned out that, on the one hand, the aramid fibers used in the sun shield protrude over the edge of the shield in some places and cause light scattering here, and on the other hand, also through diffraction at the edge of the sun shield - and subsequent multiple reflections on the surfaces of the probe - light enters the telescope openings. Due to this error, there will be slight impairment of the observation of the faintest stars. In particular, the magnitude measurement of the faintest objects suffers in terms of accuracy, and the spectral measurements are also slightly influenced.
Ice contamination
Shortly after the launch, it was discovered that the stars in Gaia's detectors apparently quickly faded. A precipitate of ice crystals on the telescope mirrors was quickly assumed to be the cause. This was proven after just a few weeks by heating a mirror on a trial basis: the loss of light disappeared exactly when the predicted temperature was reached. From February to September 2014, the Gaia telescopes were heated up a total of four times in order to eliminate the recurring precipitation. It took longer each time to return, so that at the beginning of 2015 there was hope that only one or two more decontamination campaigns would be necessary before the source of the contamination was completely extinguished. Presumably, the cause of the contamination is moisture brought along by the earth in the warm supply part of the spacecraft. This problem causes a temporary loss of light and, during and after the heating phases, a certain loss of mission time and uniformity of the sky coverage. A significant impairment of the mission objectives is not expected.
Six-hour oscillation of the telescope geometry
The interferometer on board for checking the very important geometric stability of the instruments showed a periodic variation of the angle between the two telescopes of about one milli-arcsecond from the start. This oscillation is always present as soon as Gaia rotates with the planned 6-hour period. The oscillation of one milli-arcsecond corresponds to a mutual twisting of the two telescopes of only a few nanometers. It is very precisely periodic and strictly related to the orientation of Gaia relative to the sun. The Gaia astronomers expect that the effect can therefore be calibrated very precisely and thus calculated out of the measurements.
Defective nozzle
Already in the test phase it was shown that the valve of the nozzle 3B on the chemical engines cannot be opened. For the rest of the mission, the redundant nozzle 3A was permanently switched to and the control software was modified. There is no longer any direct redundancy for this nozzle. If 3A should also fail, the remaining nozzles can be used in such a way that the function of the missing nozzle is replaced.
Tracking
To determine its exact position, Gaia is regularly observed with telescopes during the observation phases. When Gaia arrived at her specified position, it turned out that she was at the lower end of the pre-calculated brightness range when viewed from Earth. In the scientific community, Gaia was more than two magnitudes weaker than the WMAP and Planck probes with a magnitude between 20 mag and 21.2 mag . The Ground-Based Optical Tracking Unit (GBOT) that works with telescopes had to change the tracking orders and, in some cases, use different telescopes. Originally 1 to 2 meter telescopes were planned, now 2 to 3 meter telescopes are in use. The new plan enables the orbit to be reconstructed at any time in cooperation with radio telescopes and all scientific goals can be achieved.
Failure of a main transmitter
On February 18, 2018, Gaia switched to safety operations and emergency communication with the low-gain antennas after error messages. The cause was the failure of one of the two main transmitters. The operation was then switched to the second transmitter and the science mission could continue. The cause of the transmitter failure was still unclear when it was announced.
Data Processing & Analysis Consortium (DPAC)
Processing the amount of data through the earth-based computer systems was a challenge. In contrast to other missions, the raw data cannot be used without further processing. ESA had to develop new software so that the data obtained could be efficiently processed, archived and prepared for use on the ground.
The data from the antenna network is first bundled and processed in ESAC and then forwarded for scientific processing to the "Data Processing & Analysis Consortium" (DPAC), a community of astronomers and software specialists organized in nine Coordination Units (CUs), which are also responsible for the calibration the probe is responsible. The center of DPAC with the central pooling of all data is located in Villafranca del Castillo in Spain and is provided and maintained by ESA. During the planned five-year mission, the probe is expected to produce a total of over a petabyte of data, which corresponds to the data capacity of 1.5 million CD-ROMs or 200,000 DVDs. The costs for the further processing of the data are borne by the national budget, not by the ESA.
Locations
The processing is done at six locations in different countries by a team of around 450 scientists and developers with their own data centers in Villafranca, Barcelona, Cambridge, Geneva, Turin and CNES in Toulouse. The CNES data center in Toulouse stores a complete dataset of all Gaia data as a backup in another location. Further teams of scientists and developers are developing computer-aided methods at various locations with which the tasks of the CUs can be mastered. The individual locations have their own budgets and make their own decisions about how they perform their tasks and what equipment they use to do so.
Working groups
The first group, CU1, is responsible for software development and drawing up the data processing strategy. The second unit, CU2, is responsible for the simulations that were necessary to test the software before using it and to practice using it. CU1 and CU2 were already active in the very early phases of the project, while the other CUs could only start their work after the start of the probe.
Three units are responsible for the further data processing of the various detector types. CU3 takes care of the astrometric data, position and direction of movement of objects in the sky. Most of the computing capacity is required for these tasks. CU3 takes over the path from receiving the raw telemetry data to the astrometric solution, as well as a first sighting of the material (first look), and issues the science alerts . CU5 focuses on the photometric data.
Other teams are working on the evaluation of the data obtained. CU6 processes the spectroscopic data and uses it to determine radial velocities and compositions. Objects of the solar system, binary stars, exoplanets and extragalactic objects are examined by CU4. Variable stars are examined by CU7, CU8 divides all observed objects into certain classes. CU9 has the task of verifying and preparing the data for publication as well as publishing the preliminary catalogs and the final catalog, developing software and interfaces for the provision of the data and maintaining the associated servers . CU9 will continue to work indefinitely, even after the data has been completely processed and the other groups have become obsolete. CU9 will continue to develop new methods with which the data can be evaluated according to new or additional criteria.
Data processing
Astrometric Global Iterative Solution (AGIS)
At the center of the tasks is the mathematical procedure “Astrometric Global Iterative Solution” (AGIS), which gradually assembles billions of data into a map of the entire Milky Way and the universe. The accuracy of the data improves according to the number of measurements of an object. Data from all possible incoming objects are compared with already known objects. If it is a star, the object is measured several times at the same point; otherwise it is likely an asteroid or comet that has changed its position from the previous observations. All objects discovered in this way are compared with the orbits of known asteroids. If there is no match, it is potentially a newly discovered object. Movements of more than ≈ 1 as / s can already be recognized by comparing the different results from the field of astronomy sensors during an acquisition. The results are reported to the Gaia FUN SSO network, which tries to track the objects over a longer period of time in order to gain more orbit data and to prevent objects from being lost again.
Initial Gaia Source List (IGSL)
In the end, Gaia should deliver a catalog based entirely on its own data. For the initial phase, however, a way was needed to match the Gaia data with the objects from other star catalogs. For this purpose a separate catalog of 1,222,598,530 objects with the name The Initial Gaia Source List (IGSL) V. 3.0 was compiled from several earlier catalogs. Previous catalogs for calibrating the magnitudes could not be used, as most of these objects are too bright for detection with Gaia. For this reason, the IGSL catalog includes the Gaia Spectrophotometric Standard Star Catalog, a list of approximately 200 stars for photometric calibration.
Results

In contrast to the Hipparcos mission, there are no special rights to the data. All the results of the mission are to be published in several stages and no restrictions are to be imposed on the use of the data. All published data are available from the Gaia archive on the Internet.
Even before the first major publications, so-called science alerts were issued for certain objects if there was a special reason for astronomers to observe a certain object immediately. Such events are e.g. B. Occultations , the beginning of a supernova , discovery of near-Earth asteroids etc. Since September 2014, Gaia has been observing supernovae in other galaxies. In July 2015 a first map of star density was published.
Annual publications were planned during the planning phase of the mission, but the spacing between publications was later extended. The baseline models predicted approximately one billion observable objects with a magnitude of 20 or greater for Gaia. The second Gaia catalog already significantly exceeded the forecasts. As the number of objects increases, the need for computation increases disproportionately, the computation models had to be changed and the publication plans turned out to be too optimistic. The publications had to be postponed several times.
Indexing the objects
The objects in the Gaia catalogs are given a unique identification number (ID). Since the individual releases are independent of one another, these IDs can change between the individual releases. A clear specification of the objects is only possible by specifying the data release used together with the ID ( e.g. Gaia DR2 2123836077760594432 ).
Gaia Data Release DR1
On September 14, 2016, the first incomplete data sets were published with Gaia DR 1 as the result of 14 months of observation. All published objects had to have at least five observations, so there are clear gaps in certain areas. Double stars with a distance of less than 4 aa are not yet resolved with optimal results. The result of DR 1 is:
- Position and G-band magnitude (two parameters) for 1.1 billion stars, 400 million of which were not previously cataloged.
- Position, magnitude, parallax (distance), and angular velocity for more than 2 million stars using Tycho-Gaia Astrometric Solution (TGAS). Position data from the Hipparcos catalog and the Tycho-2 catalog were included and used together with the positions of Gaia to calculate the angular velocities.
- Intensity curves and specific properties of selected variable stars, including 2595 RR Lyrae stars and 599 Cepheids .
- Position and magnitude for more than 2000 quasars in the GCRF1 sub-catalog.
In the neighboring galaxy M33 , 2.4 million light-years away , Gaia recorded approximately 40,000 of the brightest of the galaxy's estimated 40 billion stars. The first publication with 1.1 billion objects despite observation gaps exceeded the prediction of around 1 billion objects by ten percent.
Gaia Data Release DR2
The Gaia DR2 catalog is another preliminary catalog with a publication date of April 25, 2018. It was generated from 22 months of observation time and contains almost 1.7 billion objects. Approximately 350 million objects of these have position and a G-band magnitude (two parameters), the remaining 1.3 billion objects also have information on parallax and angular velocity (five parameters). There are values for the red and blue photometer for around 1.3 billion objects and there is a radial velocity for 7.2 million objects. It contains 550,000 variable stars with light curves and roughly the same number of quasars that form the Gaia Celestial Reference Frame 2 as a reference frame. Around 160 million objects have values for effective temperature , 87 million have values for extinction and redshift , 76 million have values for radius and luminosity. In addition, 14,000 asteroids with orbital data are included.
Since Gaia DR2 was first published, an average of three to four scientific publications based on Gaia DR2 data have appeared daily. DR2 contains many newly discovered white dwarfs and the models for developing white dwarfs have been improved. It has been proven that white dwarfs develop a solid core when they cool down and that this process slows down the cooling process, which in turn has an impact on age determination. The data from DR2 allowed us to find out more about the Milky Way's past and how it interacted with other galaxies. DR2 was able to measure the rotational movements of bright stars within Andromeda and in the triangle nebula. The models of the movement of Andromeda and the Triangle Nebula towards the Milky Way have been improved. There is new knowledge about the star clusters within the galaxy. A number of new star clusters have been discovered, and some objects previously known as star clusters have been recognized as perspective clusters of stars that are not close together and do not belong together. A team of researchers used data from the Hubble telescope together with those from DR2 to determine the mass of the Milky Way and came up with a result of around 1,500 billion solar masses. A study was able to confirm the long-standing hypothesis that the Milky Way is a bar galaxy with direct measurements and assignment of stars to the bar. Since Gaia DR2, previously unrecognized star clusters and star groups have been identified, and it has also been recognized that newly formed stars from the same region form chain-like structures and maintain a similar movement pattern over large periods of time.
Gaia Early Data Release 3
The Gaia DR3 catalog was originally due to be released in the first half of 2021. It became apparent early on that the parts of DR3 would be ready for publication at different times.
In order to make the data available to science as early as possible, the publication was split up. The first part under the name Gaia Early Data Release 3 , Gaia EDR3 for short, is to come out towards the end of 2020 and contain improved astrometry and photometry such as: B. star locations , parallaxes, proper motion and three-band photometry. Due to the Covid-19 pandemic , the time of publication of EDR3 has been postponed by about 3 months. Gaia EDR3 is expected to contain approximately 1.8 billion objects, plus approximately 1.5 million quasars in the GCRF3 sub-catalog. The data are based on measurements carried out over a period of 34 months between July 25, 2014 and May 28, 2017, which corresponds to an observation period that is about 12 months longer than for Gaia DR2.
According to the original planning, Gaia DR3 with the remaining more complex data should be released in the second half of 2021, but will probably also be delayed due to the COVID-19 pandemic . In addition to the data from EDR3, DR3 should contain the spectroscopic and photometric object classifications for easily evaluable objects, RVS spectra and information on the stellar atmosphere, radial velocities, classifications for variable stars with photometric curves, catalogs of objects of the solar system with preliminary orbital data and individual data about their observations . Catalogs of extensive objects and multiple stars are to be added.
In autumn 2019 it was announced that DR3 will contain an additional catalog with all objects from an area in a radius of 5.5 ° around the Andromeda galaxy. This catalog, called Gaia Andromeda Photometric Survey (GAPS), is said to contain around 1 million objects.
more publishments
The final publication for the data obtained during the nominal duration of the mission was given at the beginning of the mission towards the end of 2022 (tentatively called Gaia DR4), however the preliminary publication date after Gaia DR2 has been withdrawn from the official websites. DR4 should contain all astrometric and photometric data, all variable stars, all double and multiple star systems, classifications and various astrometric data for stars, unresolved double stars, galaxies and quasars, a list of exoplanets, all epochs and transit dates for all objects.
After the mission is extended until the end of 2022, the new data will result in further publications. About three years after Gaia ceased operations, the last data should be published. The Gaia data will form the basis for research for more than ten years.
Trivia
The immersive fulldome film Billions of Suns - a journey through the galaxy tells the story of the Gaia mission. The film, produced in collaboration with ESA , was shown in 70 planetariums around the world.
The German postal brought on the Issue 7 December 2017 a stamp Gaia to 0.45 € out.
literature
- Ulrich Bastian: Project Gaia: The six-dimensional Milky Way. Part 1: Why and what for Gaia is built for. In: Sterne-und-Weltraum.de. Issue 5/2013, pp. 36–44.
- Ulrich Bastian: Project Gaia: The six-dimensional Milky Way. Part 2: Where, when and how Gaia should work. In: Sterne-und-Weltraum.de. Edition 6/2013, pp. 48–55.
- Stefan Jordan: Gaia in the test phase. In: Sterne-und-Weltraum.de. Issue 5/2014, pp. 26–28.
- S. Clark (English), German translation Stefan Jordan: Gaia; The ESA Galactic Census , ed. ESA Communications, ISBN 978-92-9221-033-5 , ISSN 0250-1589 , August 2013.
Web links
- Factsheet about Gaia. ESA (English)
- Gaia News. ESA (English)
- List of acronyms for the Gaia Mission. Jos de Bruijne (English)
Individual evidence
- ↑ Fabian Schmidt: A space probe explores the Milky Way. Deutsche Welle, December 19, 2013, accessed December 20, 2013 .
- ↑ Gaia Collaboration, T. Prusti et al .: The Gaia Mission . In: Astronomy & Astrophysics . tape 595 , A1, 2016, pp. 3 , doi : 10.1051 / 0004-6361 / 201629272 (English, aanda.org [PDF]).
- ↑ a b c d e f g h i j k Gaia Collaboration, T. Prusti et al .: The Gaia Mission . In: Astronomy & Astrophysics . tape 595 , A1, 2016, pp. 3–5 , doi : 10.1051 / 0004-6361 / 201629272 (English, aanda.org [PDF]).
- ↑ a b c d Gaia - Science Performance. ESA, June 1, 2014, accessed July 30, 2016 .
- ↑ What is Gaia? Heidelberg University, Center for Astronomy, accessed on September 11, 2017 .
- ^ Objectives. ESA, accessed on March 19, 2018 .
- ↑ Coryn Bailer-Jones: Isolated Brown Dwarfs . In: Gaia - Taking the Galactic Census . August 25, 2009, p. 5 (English, cosmos.esa.int [PDF]).
- ↑ Vasily Belokurov: Supernovae . In: Gaia - Taking the Galactic Census . August 25, 2009, p. 17 (English, cosmos.esa.int [PDF]).
- ↑ Jean-Francois Claeskens, Alain Smette: Observations of quasar . In: Gaia - Taking the Galactic Census . August 25, 2009, p. 12 (English, cosmos.esa.int [PDF]).
- ↑ Science objectives. ESA, June 14, 2013, accessed September 10, 2017 .
- ↑ C. Fabricius, U. Bastian et al .: Gaia Data Release 1; Pre-processing and source list creation . In: Astronomy & Astrophysics . tape 595 , A3, 2016, pp. 10 (English, aanda.org [PDF]).
- ^ François Mignard: Minor Planets & Near-Earth Objects . In: Gaia - Taking the Galactic Census . August 25, 2009, p. 9 (English, cosmos.esa.int [PDF]).
- ^ Jean-Marc Petit: Trans-Neptunian Objects and Centaurs . In: Gaia - Taking the Galactic Census . August 25, 2009, p. 18 (English, cosmos.esa.int [PDF]).
- ↑ Nicola Davis: Dark matter and dinosaurs: meet Lisa Randall, America's superstar scientist. In: The Guardian. January 12, 2016 (commented by Gerry Gilmore of the Gaia Mission).
- ↑ a b ESA (Ed.): Media kit for Gaia Data Release 1 . SRE-A-COEG-2016-001, September 2016, p. 9 (English, sci.esa.int ).
- ^ ESA selects prime contractor for Gaia astrometry mission. ESA, May 11, 2006, accessed December 20, 2013 .
- ^ Sun block for space astrometry. ( Memento of January 30, 2012 in the Internet Archive ) EADS Astrium, January 16, 2012 (English).
- ↑ Gaia factsheet. ESA, November 28, 2013, accessed September 10, 2017 .
- ↑ Gaia spots a Sunspot. ESA, accessed on November 2, 2017 .
- ↑ Gaia's sensors scan a lunar transit. ESA, accessed on November 2, 2017 .
- ↑ a b c GAIA (Global Astrometric Interferometer for Astrophysics) Mission. eoPortal Directory, accessed on September 10, 2017 .
- ↑ a b c d Gaia Collaboration, T. Prusti et al .: The Gaia Mission . In: Astronomy & Astrophysics . tape 595 , A1, 2016, pp. 7 , doi : 10.1051 / 0004-6361 / 201629272 (English, aanda.org [PDF]).
- ↑ # 04: Gaia Phased Array Antenna delivered and integrated. ESA, accessed on August 12, 2017 .
- ^ A b Günther Glatzel: Gaia activates measuring instruments. Raumfahrer.net, January 2, 2014, accessed January 24, 2014.
- ↑ Service Module. ESA, June 14, 2013, accessed January 24, 2014.
- ^ Franco Boldrini: Leonardo Star Trackers - Flight Experiences and Introduction of SPACESTAR Product on GEO Platforms. 11th ESA Workshop on Avionics, Data, Control and Software Systems, Amsterdam, October 17-19, 2017 (English, PDF )
- ^ Payload module - Gaia Focal Plane. ESA, October 12, 2013, accessed December 12, 2018 .
- ↑ Gaia Collaboration: Gaia Data Release 1; Documentation release 1.2 . Ed .: European Space Agency and Gaia Data Processing and Analysis Consortium. December 18, 2017, p. 19-20 ( gaia.esac.esa.int [PDF]).
- ↑ a b Gaia mirrors ready to shine. ESA, accessed on August 13, 2017 .
- ↑ First Gaia mirrors completed. ESA, accessed on August 13, 2017 .
- ↑ Payload module. ESA, accessed December 25, 2013 .
- ^ The complete Gaia CCD array (flight model). ESA, accessed on August 20, 2017 .
- ↑ AstroViews 8: Gaia and the Measurement of the Galaxy (video). Spectrum of Sciences, January 3, 2014, accessed January 6, 2014 .
- ↑ Cosmic Ray detections on Gaia CCDs - Cosmos. ESA, accessed December 1, 2018 .
- ↑ Ralf Kohley: Cosmic rays on BAM CCDs data delivery note . GAIA - DE - TN - ESAC - RKO - 033. Ed .: esac. March 17, 2015 (English, cosmos.esa.int [PDF]).
- ^ CAL Bailer-Jones et al .: The Gaia astrophysical parameters inference system (apsis). Pre-launch description. In: Astronomy & Astrophysics. Manuscript No. apsis2013, September 10, 2013 (English, PDF ).
- ↑ M. Riello, F. De Angeli, DW Evans, G. Busso, NC Hambly, M. Davidson, PW Burgess, P. Montegriffo, PJ Osborne, A. Kewley, JM Carrasco, C. Fabricius, C. Jordi, C. Cacciari, F. van Leeuwen, G. Holland .: Gaia Data Release 2; Processing of the photometric data. Ed .: A&A. 616, A3, 2018, doi : 10.1051 / 0004-6361 / 201832712 .
- ↑ F. Crifo, G. Jasniewicz, C. Soubiran, D. Katz, A. Siebert, L. Veltz, S. Udry: Towards a new full-sky list of radial velocity standard stars . In: Astronomy & Astrophysics . tape 524 , A10, 2010, doi : 10.1051 / 0004-6361 / 201015315 (English, aanda.org [PDF]).
- ^ GAIA - Satellite Missions - eoPortal Directory. Retrieved July 22, 2019 (American English).
- ↑ SCS750 - Single Board Computer for Space. DDC, Document #: 1004741 | Rev. 8 (English, PDF ).
- ↑ Larry Longden, Chad Thibodeau, Robert Hillman, Phil Layton, Michael Dowd: Designing A Single Board Computer For Space Using The Most Advanced Processor and Mitigation Technologies. Maxwell Technologies, White Paper (English, PDF ).
- ↑ Gaia video processing unit test model delivered. ESA, accessed on August 13, 2017 .
- ↑ Gaia Collaboration: Gaia Data Release 1; Documentation release 1.2 . Ed .: European Space Agency and Gaia Data Processing and Analysis Consortium. December 18, 2017, p. 30 ( gaia.esac.esa.int [PDF]).
- ↑ Payload Module - Data Handling. ESA, accessed on August 6, 2017 .
- ↑ Gaia Collaboration, T. Prusti et al .: The Gaia Mission . In: Astronomy & Astrophysics . tape 595 , A1, 2016, pp. 13 , doi : 10.1051 / 0004-6361 / 201629272 (English, aanda.org [PDF]).
- ^ Program in Progress - Gaia . In: ESA Communications Department (Ed.): ESA Bulletin . No. 162 , April 2015, p. 49 (English, esa.int ).
- ↑ a b Gaia Mission Status Numbers. ESA, accessed December 31, 2018 . This page is updated several times a day with new figures.
- ^ Arianespace successfully launches the Gaia scientific satellite. Arianespace, December 19, 2013, accessed February 23, 2016 .
- ↑ Gaia factsheet. ESA, November 28, 2013, accessed October 17, 2017 .
- ↑ Gaia enters its operational orbit. ESA, January 8, 2014, accessed January 8, 2014 .
- ↑ Günter Glatzel: Gaia has reached target orbit. Raumfahrer.net, January 8, 2014, accessed January 24, 2014.
- ↑ The flight dynamics expertise behind Gaia's critical maneuver. Gaia Blog (ESA), January 7, 2014, accessed January 24, 2014.
- ^ François Mignard: Gaia - Taking the Galactic Census. The L2 orbit. (PDF) (No longer available online.) ESA, August 25, 2009, archived from the original on March 4, 2016 ; accessed on December 20, 2013 .
- ↑ Gaia Collaboration: Gaia Data Release 1; Documentation release 1.1 . Ed .: European Space Agency and Gaia Data Processing and Analysis Consortium. February 17, 2017, p. 78-87 ( gaia.esac.esa.int [PDF]).
- ↑ Gaia Collaboration: Gaia Data Release 1; Documentation release 1.2 . Ed .: European Space Agency and Gaia Data Processing and Analysis Consortium. December 18, 2017, p. 31 ( gaia.esac.esa.int [PDF]).
- ^ Scanning Law. ESA, accessed on August 6, 2017 .
- ^ Martin Altmann et al .: GBOT - Ground Based Optical Tracking of the Gaia satellite . In: SPIE Astronomical Telescopes + Instrumentation . 2014, p. 2 (English, obspm.fr [PDF]).
- ↑ ESA (Ed.): Media kit for Gaia Data Release 1 . SRE-A-COEG-2016-001, September 2016, p. 22 (English, esa.int ).
- ^ Martin Altmann et al .: Ground Based Optical Tracking of Gaia . In: Proceedings of Gaia Follow-up Network for Solar System Objects (Workshop held at IMCCE-Paris Observatory 2010. November 29 - December 1) . S. 27 (English, gaiafunsso.imcce.fr [PDF]).
- ↑ NASA's WMAP poses for ESA's Gaia. ESA, accessed on August 13, 2017 .
- ↑ The reference stars (attitude stars) are single bright stars. For the first calculations they are listed with their previously known positions in the Attitude Star Catalog , which is part of the Initial Gaia Source List (IGSL).
- ↑ [email protected]: Tracking Gaia to Map the Milky Way - ESO's VST is helping determine the orbit of the spacecraft for the most accurate map ever of more than a billion stars. Retrieved August 15, 2019 .
- ↑ Gaia Collaboration: Gaia Data Release 1; Documentation release 1.1 . Ed .: European Space Agency, Gaia Data Processing and Analysis Consortium. S. 162 ( gaia.esac.esa.int [PDF]).
- ↑ Gaia's biggest operational since launch and commissioning. ESA, accessed July 15, 2019 .
- ^ Whitehead Eclipse Avoidance Maneuver marks Gaia's start of mission extension. ESOC, accessed July 22, 2019 .
- ↑ Green light for continued operations of ESA science missions. ESA, accessed December 31, 2017 .
- ^ Extended life for ESA's science missions. ESA, accessed on November 29, 2018 .
- ↑ a b Gaia Collaboration, T. Prusti et al .: The Gaia Mission . In: Astronomy & Astrophysics . tape 595 , A1, 2016, pp. 18 , doi : 10.1051 / 0004-6361 / 201629272 (English, aanda.org [PDF]).
- ↑ Gaia's snapshot of another galaxy. ESA, accessed on August 5, 2017 .
- ↑ JHJ de Bruijne, M. Allen, S. Azaz, A. Krone-Martins, T. Prod'homme, D. Hestroffer: Detecting stars, galaxies, asteroids and with Gaia . In: Astronomy & Astrophysics . tape 576 , A74, 2015, doi : 10.1051 / 0004-6361 / 201424018 (English, aanda.org [PDF]).
- ↑ Gaia Collaboration, T. Prusti et al .: The Gaia Mission . In: Astronomy & Astrophysics . tape 595 , A1, 2016, pp. 11 , doi : 10.1051 / 0004-6361 / 201629272 (English, aanda.org [PDF]).
- ↑ Stefan Jordan: The sky surveyor Gaia is plagued by small mishaps. In: Spektrum.de . February 4, 2014, accessed December 12, 2018.
- ^ A. Mora et al .: Gaia: focus, straylight and basic angle. In: Proceedings of the SPIE. Volume 9904, No. 99042D, 2016, doi: 10.1117 / 12.2230763 (English, PDF ).
- ↑ a b c d Commissioning review: Gaia ready to start routine operations. ESA, accessed on August 13, 2017 .
- ↑ Gaia Collaboration, T. Prusti et al .: The Gaia Mission . In: Astronomy & Astrophysics . tape 595 , A1, 2016, pp. 15 , doi : 10.1051 / 0004-6361 / 201629272 (English, aanda.org [PDF]).
- ^ Martin Altmann et al .: GBOT - Ground Based Optical Tracking of the Gaia satellite . In: SPIE Astronomical Telescopes + Instrumentation . 2014, p. 6 (English, obspm.fr [PDF]).
- ↑ Gaia status update: safe mode and recovery. ESA, accessed April 12, 2018 .
- ↑ The DPAC Consortium. ESA, accessed on August 13, 2017 .
- ^ RL Smart: The Initial Gaia Source List and the Attitude Star Catalog GAIA-C3-TN-OATO-RLS-004-02 . Ed .: Gaia DPAC Data Processing & Analysis Consortium. October 17, 2013 ( dms.cosmos.esa.int ).
- ↑ Gaia Archives. ESA, accessed April 13, 2018 .
- ↑ Gaia discovers its first supernova. ESA, accessed December 12, 2018 .
- ↑ Counting stars with Gaia - Annotated Image . ESA. Retrieved July 16, 2015.
- ↑ a b Davide Castelvecchi: Detailed map shows Milky Way is bigger than we thought. Nature News, September 14, 2016, accessed September 16, 2016 .
- ↑ a b Gaia Data Release 1 (Gaia DR1). ESA, accessed on August 6, 2017 .
- ↑ Gaia's billion-star map hints at treasures to come. ESA, September 14, 2016, accessed December 12, 2018.
- ↑ Gaia Collaboration: Gaia Data Release 1; Summary of the astrometric, photometric, and survey properties . In: Astronomy & Astrophysics . tape 595 , A2, 2016, doi : 10.1051 / 0004-6361 / 201629512 (English, aanda.org [PDF]).
- ↑ An extragalactic star-forming region. ESA, accessed April 13, 2018 .
- ↑ Gaia Data Release 2 (Gaia DR2). ESA, accessed February 11, 2018 .
- ↑ Gaia reveals how Sun-like stars turn solid after their demise. Retrieved July 21, 2019 (UK English).
- ^ Gaia clocks new speeds for Milky Way-Andromeda collision. Retrieved July 21, 2019 (UK English).
- ^ Rethinking everything we thought we knew about star clusters. Retrieved July 21, 2019 (UK English).
- ↑ Hubble & Gaia accurately set weigh the Milky Way [heic1905]. Retrieved July 21, 2019 (UK English).
- Jump up ↑ Laura L. Watkins, Roeland P. van der Marel, Sangmo Tony Sohn, N. Wyn Evans .: Evidence for an Intermediate-mass Milky Way from Gaia DR2 Halo Globular Cluster Motions . In: The Astrophysical Journal . tape 873 , 2, article id. 118, doi : 10.3847 / 1538-4357 / ab089f , arxiv : 1804.11348 ( arxiv.org [PDF]).
- ↑ Gaia starts mapping our galaxy's bar. Retrieved August 31, 2019 (UK English).
- ↑ Gaia untangles the starry strings of the Milky Way. Retrieved August 31, 2019 (UK English).
- ↑ News 2020 - Gaia - Cosmos. Retrieved March 25, 2020 .
- ↑ a b Gaia Early Data Release 3 (Gaia EDR3). Retrieved August 22, 2020 .
- ↑ a b Data Release scenario. ESA , 2019, accessed October 1, 2019 .
- ↑ News 2019 - Gaia - Cosmos. Retrieved October 1, 2019 .
- ^ Journey to a Billion Suns. Stargarten, Adam Majorosi, 2014, accessed December 12, 2018.
- ↑ Journey to a Billion Suns has been shown at these places world-wide. planetariumshow.eu, accessed on November 23, 2016 .
- ↑ Gaia, postage stamp at € 0.45. Deutsche Post AG, accessed on December 12, 2018.
- ^ Release of a German Gaia stamp. ESA, December 8, 2017, accessed December 12, 2018.