Proboscis
Proboscis | ||||||||||||
---|---|---|---|---|---|---|---|---|---|---|---|---|
![]() Asian elephant ( Elephas maximus ) |
||||||||||||
Temporal occurrence | ||||||||||||
Zealand ( Paleocene ) to date | ||||||||||||
60 to 0 million years | ||||||||||||
Locations | ||||||||||||
|
||||||||||||
Systematics | ||||||||||||
|
||||||||||||
Scientific name | ||||||||||||
Proboscidea | ||||||||||||
Illiger , 1811 |
The mammoths (Proboscidea) are an order of mammals that today with the African elephant , the forest elephant and the Asian elephant three types within a family , the elephant belonging. The order was named after its trunk as the most striking external feature. Further characteristics of today's representatives can be found in the generally large and massive physique with columnar legs, the voluminous head and short neck as well as the tusks , which result from the enlarged upper incisorsdeveloped. The recent elephants are widespread in the tropical regions of Africa south of the Sahara , in South and Southeast Asia as well as in parts of East Asia and use a variety of different landscapes. They live in complex social associations with herds of mother and young animals as well as solitary males, which can sometimes also form bachelor associations. Complex communication takes place between the individual individuals. The diet consists mainly of plants, here both grasses and leaves , fruits and the like. The exact composition varies with the seasons. As a rule, a cub is born several years apart and grows up in the herd.
The origin of the order goes back to the Paleocene around 60 million years ago. The oldest forms are known from northern Africa. These are still relatively small, partly aquatic animals without proboscis and tusks. Both features emerged later. In the course of the tribal history, different families emerged, of which the Deinotheriidae , the Mammutidae , the Gomphotheriidae and the Stegodontidae are the best known. The families and their members reflect the diversity of the proboscis. The proboscis reached Eurasia over a land bridge around 20 million years ago at the latest and spread there. Some representatives migrated to America , so that the proboscis had an almost worldwide distribution, with the exception of Australia , Antarctica and most of the islands far from the mainland. The animals adapted to the most varied of habitats, ranging from the tropical and wooded areas of origin to high mountain landscapes and arctic open lands. The elephants represent the youngest link in the evolution of the phyla and first appeared around 7 million years ago in the Upper Miocene . At the end of the Pleistocene , the majority of the proboscis became extinct.
The scientific name of the proboscidea comes from the year 1811. Initially, the group was associated with a wide variety of ungulates . An assumed closer family relationship to the manatees and the slivers first appeared in the second half of the 19th century. This community, later known under the name Paenungulata , was later substantiated both in terms of skeletal anatomy as well as genetically and biochemically . In the transition from the 20th to the 21st century, molecular genetics in particular showed that the proboscis are more closely related to other originally African animals. The resulting family group, to which, in addition to the Paenungulata, various insectivorous animals such as the elephant , the Tenre-kartigen and the aardvark , was named Afrotheria . Henry Fairfield Osborn did outstanding work in the study of proboscis in the first third of the 20th century .
features
General and habitus

Today's proboscis are the largest animals living on land. The body weight ranges from 2 to over 6 t with a shoulder height of 2 to 4 m. The animals are characterized by a massive body, a massive head on a short neck and columnar legs. The most noticeable external features can be found in the extremely long trunk , which gives it its name, as well as in the tusks and the large, lamellar-like molars . The body is usually only slightly hairy. A special feature of the soft tissue anatomy is the temporal gland on the side of the eyes, which only occurs in elephants. In their phylogenetic past, the proboscis showed a fairly high degree of variability. The body size ranged from around 8 kg small animals from the earliest phase of the order to gigantic forms with a body weight of up to 16 t. With regard to body size and individual external features such as the tusk shape, there is a clear sexual dimorphism in today's species , which can also be detected in some extinct forms.
Both the trunk and the tusks developed evolutionarily only gradually. As a common characteristic ( synapomorphy ) of all proboscis, among other things, the generally enlarged central incisors of the upper row of teeth - from which the tusks later emerged -, the loss of the anterior premolars and enamel prisms with a keyhole-like cross-section can be emphasized.
Skeletal features
skull


As an anatomical feature, the very large head of the proboscis has a cranial roof interspersed with air-filled cavities. These honeycomb- shaped chambers, which are separated from one another by thin bone platelets, run through the frontal , parietal and nasal bones as well as the central jawbone . They not only reduce the weight of the entire skull , but at the same time lead to an enormous increase in the volume of the skull surface. This increase in volume was necessary, on the one hand, to ensure the hold of the head, including the continuously growing tusks, via the powerful neck muscles, and, on the other hand, to give the strong masticatory muscles for the massive lower jaw the attachment surface. The development of such air-filled skull began phylogenetically very early in the trunk animals and, in some representatives as barytherium early as the Oligocene , possibly as early as the late Eocene proven.
In numerous early proboscis animals, the lower jaw is characterized by an elongated symphysis , which connects the two halves of the jaw at the front end. The stretching of the lower jaw often resulted from the formation of the lower tusks, the alveoli of which were attached to the side of the symphysis. As a result, these early proboscis show a rather elongated facial area and can thus be regarded as longirostrin ("long-snouted"). In several lines of the proboscis, such as the Mammutidae , the Gomphotheriidae and the Elephantidae , the symphysis and thus the facial area were shortened in the further course of the tribal history, mostly associated with the reduction of the lower tusks. These forms are called brevirostrin ("short-snouted").
denture
Generally
The original permanent proboscis teeth still had the complete dentition of the higher mammals with 44 teeth consisting of three incisors, one canine , four premolars and three molars per jaw branch. Therefore, the following can be used for original Rüsseltiere dental formula are given: . In the course of phylogenetic evolution, the number of teeth decreased continuously. This led to the loss of the canine, the rear incisors and the anterior premolars to the teeth of today's elephants, which have only one incisor in the upper row of teeth (tusk) and three molars per jaw arch. There are also three milk premolars. The dental formula of modern Rüsseltiere is therefore: . In addition, some older representatives of the crown group of the proboscis ( Loxodonta , Elephas and Mammuthus ) still had two permanent premolars. The loss of permanent premolars occurred independently of one another in the respective lines. The three deciduous premolars are also referred to as deciduous molars by some experts due to their strong similarity to the molars, but are premolars from an ontogenetic point of view.
Anterior dentition and tusks

The most striking skeletal element are the tusks, which today's elephants generally have in the upper row of teeth. Many early representatives of the proboscis also had tusks in the lower jaw. In the case of the Deinotheriidae and some other representatives, they only occurred there. Their shape is very variable. For example, they can be bent upwards or downwards and twisted, or they can run more or less straight or have a shovel shape. Sometimes they are close together or far apart. The length is also different. In early proboscis they were more like tusks that protruded vertically at the top and often horizontally at the bottom, whereas in some mammoths and mammoths they were 4 to 5 m long. In principle, the tusks are the formation of the incisors , which hypertrophied in the course of the tribal history in connection with the general reduction in the number of teeth in the teeth of the proboscis and thus grew into the largest teeth of all known extinct or still living animals. The upper tusks emerged from the second incisor (I2) of the branch of the jaw, while the origin of the lower tusks has long been discussed among experts, but has now been identified with the first incisor (I1) of the branch of the jaw. Exceptions can be found in the Moeritheriidae , in which the lower tusks also come from the second incisors, while in the Barytheriidae each branch of the jaw had two tusks, making a total of eight. These corresponded to the first two incisors of the mandibular arch. The gradual elongation of the tusks throughout the tribal history also brought about functional changes. Today elephants use their large tusks in a variety of ways, for example to help with food intake, to transport heavy objects or in rival fights. Originally, however, the tusks were primarily used for food intake. The vertical position in the upper row of teeth and the horizontal one in the lower one created a kind of scissors with which food plants could be cut.
Since the tusks of the proboscis often have a relatively similar structure, an exact allocation is rather difficult for individual finds. However, there are differences in the details. Structurally, the tusks consist of three units. Inside is the pulp , the main growth zone. This is enveloped by the dentin , which makes up the majority of a tusk and corresponds to the actual "ivory". It is a mixture of carbonate - hydroxyapatite crystals, which are connected with collagen fibers . The former are mainly responsible for the hardness of the tusks, the latter for their elasticity. As a result, the dentine is strongly mineralized and free of cells . It is formed in small canals that are arranged radially around the center of the tusk. As a rule, these canals have varying shapes, but they meet at acute angles. The canals closer to the center are usually larger than those at the edge. As a result, there are differences in the density and absolute number of canals within a tusk. These canals are partially broken through by dentin ribs that run parallel to the core of the tusk. This general dentin structure shows individual variations within the proboscis. Representatives of the Mammutidae have a higher proportion of dentin ribs towards the core of the tusk, while the tusks of the Gomphotheriidae and Elephantidae are more homogeneous both near the pulp and towards the edge. It is unclear here which of the two represents the more original variant. The outer shell of the tusk is formed by a thin layer of dental cement . Here, too, there are individual deviations between the various proboscis. In the case of lines that are older in ancestral history, a surrounding layer of tooth enamel is often still formed, which surrounds the tusk in the form of a band. In the Elephantidae, this enamel layer is mostly completely absent.
The tusks of today's elephants have an internal pattern of alternating light and dark colored areas as a further conspicuousness. In the longitudinal direction of the teeth they result in a band pattern, but in the tooth cross-section they are arranged in a checkerboard manner. Due to the round shape of the tusk, the checkerboard pattern consists of rhombic surfaces with a radial to tangential arrangement, which gives the visual impression of a complex spiral structure. The angles at which the rhombuses lie against each other differ between the species and thus have taxonomic value. The pattern is called "Schreger lines" and is not known in mammals of other orders with tusk-like formations. The origin of the "Schreger lines" is controversial. According to various theories, they arise either from the arrangement of the dentin tubules or from the special orientation of the collagen fibers. Histological examinations were able to detect the "Schreger lines" also in other trunk animals such as anancus and stegodon , all of which belong to a closer relational environment of the elephants. Remarkably, however, they do not occur in phylogenetically older forms such as Deinotherium . Some researchers therefore believe that it is a unique characteristic of elephantoid proboscis that emerged only once during the evolution of the proboscis. However, "Schreger lines" are also documented in Gomphotherium and Mammut .
Rear dentition and change of teeth
The structure of the molars is mainly used for the systematic classification of the proboscis. The chewing surface of these molars is very diverse and adapted to the respective way of life of the animals. The most important basic types are as follows:
Tooth type | description | Examples | photo |
---|---|---|---|
bunodont | Basic type of proboscis teeth; the chewing surface pattern consists of larger conical cusps, which are arranged in pairs across the longitudinal axis of the tooth; in the middle of the longitudinal axis of the tooth there is a deep groove and divides the pairs in half; Small side humps are based on the main humps, the number of which can increase from two to four or more per half-list in the course of the development of the tribe; there are also ridges and ridges that sometimes block the longitudinal groove and the transverse furrows between the ridges; the arrangement of the cusps, secondary cusps and locking strips is partly irregular, so a clover leaf pattern emerges when chewed off; Phylogenetically younger proboscis continue to develop the bunodonte pattern, so the individual half-lists can be offset to one another ("anancoidia"), form a V (" chevroning ") or there are numerous furrows and small bumps ("ptychodontia" or "choerodontia") | early proboscis such as Eritherium and Saloumia , Moeritheriidae , Gomphotheriidae |
Molar from Gomphotherium |
zygodont | developed out of the bunodont type; the cusps form a sharp ridge, the longitudinal groove and the transverse furrows are often not blocked by ridges and ridges; the side humps are in line with the main humps, so that straight yokes are created, some of which remain visible even after they have been chewed off; generally, zygodontic molars are wider than bunodontic ones | Mammutidae |
![]() Mammut molar |
lophodont | also emerged from the bunodont type; the conical humps are fused together by sharp ridges, which in turn are formed from a high increase in the number of secondary humps | Numidotheriidae , Phosphatheriidae , Barytheriidae , Deinotheriidae Stegodontidae |
![]() Molar of Deinotherium |
lamellodont | again derived from the bunodont pattern; the tooth is formed from lamellar enamel folds ; Like the lophodont teeth, the enamel lamellae result from the increasing number of sinus cusps | Elephantidae |
![]() Molar of Mammuthus |
There are also individual transitional forms such as bunolophodont , bunozygodont or zygolophodont . The earliest proboscis such as Eritherium had a bunodont pattern. The individual tooth shapes then developed independently of one another within the various proboscis lines. As a result, stem forms of some lines such as Losodokodon in the Mammutidae showed even more distinct bunodontic molars, from which the typical zygodontic pattern of the later forms developed. The proboscis molars can be derived from the originally tribosphenic teeth of the higher mammals, the chewing surface of which is strongly topographically structured. The tribosphenic teeth consist of a raised area, called trigon for the upper jaw and trigonid for the lower molar teeth, on which the three main cusps are located. These main cusps are in turn designated as Para-, Proto- and Metaconus on the upper and as Paraconid, Protoconid and Metaconid on the lower molars; In numerous derivations from the tribosphenic basic structure of the molars, a fourth cusp, the hypoconus, has mostly formed independently of one another in many mammalian lines on the upper molars. The trigon is followed by a lower section with the minor cusps, which in turn forms the so-called talon in the upper jaw and the talonid in the lower molar teeth. On the often more voluminous molars of the lower jaw, the talonid has some larger cusps, such as the hypoconid and the entoconid. In the proboscis teeth, the front part comprises the trine or the trigonid, the rear part the talon or talonid. In relation to the maxillary molars, the anterior pair of cusps corresponds to the paraconus and the protoconus with the protoloph as a transverse ridge or yokes (on the mandibular molars consequently the protoconid and the metaconid as well as the protolophid). The second pair of cusps is formed by the metaconus and the hypoconus, both of which are connected by the metaloph (on the lower molars, the hypoconid and entoconid as well as the hypolophid). The pairs of cusps that follow to the rear then emerge from the talon / talonid. A longitudinal groove that runs centrally over the entire length of the tooth divides the pairs of cusps or ridges or yokes into two half-ridges / half-yokes, the tongue-side area of which is referred to as entoloph (entolophid) and the cheek-side area as ectoloph (ectolophid). This is particularly noticeable in bunodont and zygodont , but less so in lophodont and lamellodont molars. However, all proboscid molars therefore have two partial tooth surfaces that are more or less stressed due to the animals' chewing mechanism. The more worn part is named pretrit, the weaker part is named posttrit. In the upper jaw, the more heavily chewed tooth part is always on the tongue side, the less thick part on the cheek side. With the mandibular molars it is exactly the opposite.
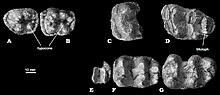

Another special feature is the increasing complexity and relative and absolute enlargement of the molars. The first two molars of the earliest proboscis had a total of two pairs of cusps, so they were bilophodontic (with two ridges or yokes). The third molar often had three ridges, even if the third was partially poorly developed. The cusps of the two (front) ridges correspond to the trine or the trigonid. As the process progressed, the number of ridges on the first two molars initially increased to three, which resulted in trilophodontic teeth (with three ridges or yokes). Here, the two anterior molars began to differentiate from the posterior one, since the latter usually has a higher number of ridges, initially between four and five (generally the posterior lower molar has more ridges than the posterior upper one). The new additional pairs of cusps were created from the talon or talonid, each additional ridge is basically just a duplication of the previous one. In modern elephants, this developed to the extreme, since, for example, the rear molar tooth within the mammoth can have up to 30 ridges. The molars of the more modern proboscis lines are the largest of the mammals with lengths of sometimes over 20 cm and sometimes more than 5 kg.
Today's proboscis have high-crowned ( hypsodontal ) molars, which means that the height of the crown of a molar is significantly greater than its width, sometimes by double. The massive increase in crown height is a development of modern elephants and represents an adaptation to hard grass food, favored by the spread of the grasses in the course of the Miocene. In spite of this, a certain increase in the height of the crown can be seen in individual older proboscis lines such as the Gomphotheriidae. However, the majority of the representatives of the proboscis had low-crowned, i.e. brachyodontic molars. A special feature of the developed proboscis is the shape of the enamel, which consists of three layers: an outer layer of radially aligned prisms , a middle layer of Hunter-Schreger bands (variable light or dark striped enamel bands with differently directed prisms) and an inner layer of a three-dimensional network of interwoven prism bundles. There are differences to the earliest proboscis such as the Phosphatheriidae , the Moeritheriidae or the Phiomiidae in which the enamel is usually composed of only two layers, with the two upper layers often, but not always, occurring. Deviating from this, the teeth of the Deinotheriidae mainly consist only of the interwoven prismatic bundles. The latter formation in particular is viewed as a special hardening stage of the tooth enamel, which means that it can better withstand the horizontally acting forces during the chewing process. According to this, the particularly hard variant of the tooth enamel developed in the proboscis well before the point in time at which higher tooth crowns formed, which can be understood as a type of pre-adaptation .
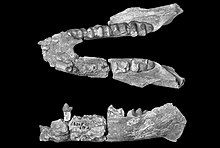

Today's elephants do not change their teeth vertically, as in most mammals, but horizontally. As a result, of the three premolars and three molars per jaw arch, usually only one to one and a half are functioning at the same time. These broken teeth are heavily worn down when the plant food is chewed. Meanwhile, new tooth material forms in the back of the dentition and is continuously pushed forward. This means that if a tooth is lost, the elephant has a new one available. This process can be repeated a total of five times after the first deciduous premolar has erupted, which corresponds to six generations of teeth (three deciduous premolars and three molars). The horizontal change of teeth resulted from the enlargement of the teeth as a result of the formation of additional ridges and the shortening of the jaw during the evolution of the proboscis animals, so that not all teeth could be accommodated at the same time. This feature first appeared in the course of the Oligocene. Before this drastic change, the proboscis changed their teeth in the vertical mode, comparable to other mammals. With these representatives, all teeth of the permanent dentition were accordingly in function at the same time. The change in the change of teeth may be associated with a general change in eating habits and in the chewing process. The Deinotheriidae changed their teeth vertically, while the Gomphotheriidae, which appeared at the same time and were almost the same size, changed their teeth horizontally. The latter had significantly larger teeth with more ridges than the former, which enabled a wider range of food in terms of signs of wear and the like.
Body skeleton
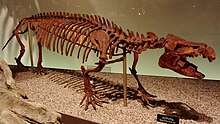
Special skeletal anatomical features are the columnar limbs that stand vertically under the body, with the upper and lower parts of the extremities forming an angle of 180 °. This distinguishes the proboscis from numerous other mammals, whose legs are arranged at a slight angle. The clearly vertical position, which was already fully developed in the Deinotheriidae, supported the enormous increase in weight of the early representatives of this order. Ancient trunk animals from the Eocene such as Numidotherium and Barytherium had significantly more angled legs. In addition, the limbs with their long upper extremity sections show adaptations to the high weight ( graviportal ) compared to short lower ones . The ulna and radius are not fused together, but fix each other when the front leg is turned. The thighbone is narrowed in front and behind. Furthermore, the long bones do not have a bone marrow cavity , but the space is filled with cancellous bone , which gives the legs greater strength. The blood formation takes place in the interstices. The individual carpal and tarsal bones are arranged in series, that is, they do not overlap on the joint surfaces, but lie one behind the other ( taxeopod ). The front and rear feet usually have five rays each. Another special feature of their feet is a sixth "toe", which is attached to the back of the thumb or the big toe . The attachment point is located at the upper ( proximal ), inward-pointing joint end of the metacarpus I (thumb) or the metatarsus I (big toe) and consists of cartilage material that is partially ossified. It is used to support the other toes in stabilizing the high body weight. The formations on the forefoot are referred to as prepollex ("fore-thumb") and on the rear foot as prehallux ("fore-toe"). These are also already detectable in the Deinotheriidae and thus go back to the early Miocene. The development of this anatomical peculiarity is related to the enormous increase in body size of the proboscis at that time, which is connected with the turning away from the more amphibious way of life of the early Proboscidea representatives to a purely terrestrial one. This also changed the general foot position. Original proboscis had more horizontally oriented foot bones and were functionally adapted to the sole passage . In today's elephants, the toes form a semicircle and are more clearly perpendicular. This allows the elephants to be seen as tiptoe . The sixth "toe" formed as a support element during the redesign of the foot anatomy and the massively increasing body mass at the same time.
Soft tissue anatomy
Trunk
The most striking characteristic of the proboscis is the proboscis , which arises from the fusion of the upper lip with the nose and is already formed in today's elephants in the embryonic stage . The two nasal passages emerge at the lower end of the trunk. It consists of up to 150,000 longitudinal, circular or oblique muscle fibers from a large number of different muscles and, as a sensitive grasping organ, performs numerous functions. Primarily it bridges the distance between the head and the ground, which the short neck of the animals can no longer achieve. This makes it essential for food intake by tearing off plants growing in the ground or by absorbing water and leading it to the mouth. Conversely, it can also be used to reach higher objects such as leaves in the treetops. In addition, it serves as a respiratory and transport organ and is also very important as a tactile and sensory organ in social communication. As a purely muscular formation, the trunk occupies a larger part of the facial area of the skull. Its formation resulted in a massive restructuring and shortening of the bony substructure. Above all, this includes a significant reduction in the nasal bone, which usually only exists as a short extension. The nostril, on the other hand, is extremely large. The redesign of the facial skull gave the massive trunk muscles extensive attachment points.
In fossil proboscis, the presence of a proboscis can only be deduced indirectly from the facial anatomy, mainly the nasal region. Accordingly, the earliest forms did not yet have a proboscis. The first signs of a proboscis can be found in Numidotherium from the Lower to Middle Eocene. The development possibly began with only short, rather tapir-like proboscis, which emerged from a very flexible upper lip and only gradually lengthened as the body increased in size. Even for the sometimes huge representatives of Deinotherium , a rather short trunk is assumed due to the high position of the nostril towards the top of the skull, but it must have been long enough to reach the bottom. Some researchers assume that the proboscis developed independently several times within the proboscis animals.
brain
The proboscis belong to the few groups of mammals in which a brain of more than 700 g developed, comparable to primates and whales . Today's elephants have a brain volume of 2900 to 9000 cm³. Based on a body weight of 2.2 to 6.6 t, this corresponds to an encephalization quotient of 1.1 to 2.2, the average value is 1.7. In comparison, the encephalization quotient in humans is around 7.5. The structure of the elephant's brain is complex. It consists of around 257 billion nerve cells , which is 3 times the amount of the human brain. In contrast to this, around 98% of the elephants' nerve cells are formed in the cerebellum . In addition, the temporal lobe is more developed than in humans. The brains of extinct proboscis animals are only seldom passed down, with the exception of individual ice mummies from the woolly mammoth ( Mammuthus primigenius ). For other fossil forms, information on the dimensions of the brain is only available in exceptional cases, which was then largely obtained on the basis of pourings from the interior of the brain skull. It was shown here that some dwarfed elephants such as the Sicilian dwarf elephant ( Palaeoloxodon falconeri ) had a comparatively large brain that reached around 1800 cm³ with a body weight of 190 kg and thus led to an encephalization quotient of around 3.75.
The oldest known skull spout belongs to Moeritherium , which belongs to the transition from the Upper Eocene to the Lower Oligocene. His brain volume was estimated to be 240 cm³, with an assumed body weight of 810 kg. The encephalization quotient can thus be given as around 0.2. A proportionally comparable relationship between brain size and body weight was developed in the palaeomastodon of about the same age . Its brain volume was 740 cm³, the body weight was 2.5 t and the encephalization quotient was 0.3. The brain volume then gradually increased in the course of the tribal history, which apparently took place in parallel with the increase in body weight. However, faster brain growth can be determined compared to body size, since the encephalization quotient is also increased. However, this did not take place at the same speed in all lines of the proboscis. The Mammutidae, which form a relatively original line within the proboscis, but appeared around the same time as the modern elephants and only disappeared in the Pleistocene, had a relatively small brain. Zygolophodon only had an encephalization quotient of 0.5, resulting from a brain of around 5130 cm³ and a body weight of up to 16 t. The American mastodon ( Mammut americanum ) as the end member of the mammoth development had values of 3860 to 4630 cm³ for the brain volume, 6.4 to 8.0 t for the body weight and correspondingly 0.30 to 0.74 for the encephalization quotient. It is unclear what caused the significant increase in brain volume in the proboscis. Compared to the earliest proboscis from the Eocene and Oligocene, all of the more modern lines from the Miocene show significantly larger brains, also in terms of body weight. The time phase in Africa was characterized by increasing desiccation due to climate change. In addition, a land bridge to Eurasia, which made a faunal and in consequence of the mammoths and other large herbivores in was competition occurred.
Coat
The three elephant species living today have only a sparse coat of fur. Longer and denser tufts of hair are only formed on the chin, the tip of the trunk and the end of the tail. The almost missing hair coverage is a result of the enormous body size of the animals, their distribution in warm tropical climates and the resulting thermoregulation . It usually occurs through the skin, ears and sometimes through fluctuating body temperature. A thick coat, however, was necessary for the woolly mammoth due to its occurrence far north in arctic and subarctic regions and is documented by numerous mummified carcasses from the permafrost area . The fur is made up of a thick, frizzy undercoat and long outer hair . Structurally, the hair of today's elephants and woolly mammoths are similar. They have a conical shape that becomes more pointed towards the end and are characterized by a largely missing pith. Their length varies from 10 to 13 cm, their color from yellowish-brown to dark brown to blackish, whereby a varying hair color in woolly mammoths can also be genetically determined. To what extent phyllo-phonic older and sometimes significantly smaller proboscis had fur cannot be said with certainty at the moment, a large part of the known forms lived under tropical climatic conditions. For the American mastodon, which was native to the temperate coniferous and mixed forests of North America, remnants of fur have survived, which apparently also consist of dense undercoat and long outer hair.
distribution
Today's elephants are widespread in the largely tropical landscapes of Africa south of the Sahara and South , Southeast and East Asia. Their habitat is diverse and, depending on the species, includes dense tropical forests , open savannah and bush areas, as well as desert-like regions. The origin of the group is most likely in Africa, where it was first detectable in the Paleocene around 60 million years ago. At that time, the continent formed a common land mass with the Arabian Peninsula , but was not yet connected to other parts of the world by land bridges. Such land bridges were not built until the Lower Miocene , more than 20 million years ago, when the Tethys Ocean to the north closed, creating a connection to today's Eurasia . The Mammutidae and Gomphotheriidae , who first set foot on Eurasian soil, were among the first to emigrate . Some representatives, such as Zygolophodon or Gomphotherium , also reached the North American continent via North Asia , where independent lines of development then emerged. The first emigrants were followed by the Deinotheriidae , although they did not spread as far as the Mammutidae and Gomphotheriidae, but remained restricted to Eurasia. The first wave of emigration took place around 20 to 22 million years ago. The occurrence of proboscid animals outside of Africa is known as the Proboscidean datum event , although this event , which was originally regarded as a singular event, according to recent studies, consisted of at least half a dozen individual phases. In North America proboscis have been documented since the Middle Miocene a good 16 million years ago. In the course of the formation of the Isthmus of Panama and the formation of a closed American land mass in the Pliocene 3 million years ago, the Great American Fauna Exchange took place , as a result of which some representatives of the Gomphotheriidae also colonized South America .
The proboscis were once spread over a large part of the Old and New World , only the Australian and Antarctic continents and most of the islands far from the mainland, such as Madagascar and New Guinea , they never reached. Their habitat included not only the tropical landscape areas as with today's representatives, it extended far north into the Arctic region , especially in the Pleistocene . As a rule, the various proboscis used lowlands as habitats , some relatives such as Cuvieronius had also opened up mountainous highlands. Some islands near the coast were also reached, where the proboscis then developed typical dwarf forms. Until the late Pleistocene, the proboscis were widespread with several families over America, Eurasia and Africa. Today they can only be found with a family in Africa and South Asia in the form of elephants.
Way of life and ecology
Social behavior and reproduction

The elephant's way of life is relatively well documented. There is a complex social system within the individual species. The mother-young animal community forms the basis. Several of these small units form a family group or herd consisting mainly of related animals. In addition, there are higher-level associations such as family associations, clans and the like, which, however, in contrast to the herd, are often only of a temporary nature. Male animals, on the other hand, are largely loners or gather in bachelor groups. To what extent such a group formation can be transferred to the extinct representatives is in many cases uncertain and at best has been partially documented for the members of the youngest lineages. Trace fossils from the Baynunah formation in the United Arab Emirates show that at least a certain social structure was already formed in the Miocene around 8 to 9 million years ago . Here there are at least 13 tracks of proboscis animals, which run parallel to each other over a width of 20 to 30 m over a distance of 190 m. Due to the size of the individual step seals and the length of the stride, several larger and one relatively small individuals can be kept apart, the individual size of the polluters is within the range of variation of today's elephants. The track group is crossed by a single track of an extremely large animal, which is one of the longest continuous tracks in the world with a length of 260 m. The connected, parallel tracks probably represent a herd consisting of old and young animals, the individual transverse tracks can therefore be traced back to a solitary male animal. Since the step seals themselves do not reveal any further anatomical details and the Baynunah formation harbors various proboscis such as Deinotherium and Stegotetrabelodon , it is unclear to which form they belong (there is also the possibility that they come from different species or genera).
Intensive communication takes place on various levels within the individual social structures of the elephants. This is controlled, among other things, by optical signals such as posture and gestures or by chemical stimuli from the faeces and urine or secretions . Chemical signals are used to differentiate between family members and unfamiliar individuals. In addition, there is tactile communication by means of the trunk. Sound communication, which is very diverse and in many cases uses infrasound , is of outstanding importance . Above all, the social rumbling can be emphasized here, which covers frequencies from 10 to 200 Hz and serves to establish mutual contact. In addition, elephants are known for their cognitive performance, which, in addition to long-term memory, also includes learning foreign noises, manipulating the environment, social empathy, dealing with deceased conspecifics and self-reflection. Typically, such complex behaviors are difficult to identify with fossil forms. However, the structure of the inner ear can at least provide conclusions about the frequencies that may be perceived and thus the sound communication. Today's elephants have a cochlea with a double turn, which combined results in 670 to 790 °. The number of turns is often associated with the ability to perceive certain frequencies. In addition, the lamina spiralis secundaria is missing at the base turn, which means that the basilar membrane is quite extensive, which in turn is considered an adaptation to hearing in the infrasound range. For some of the earliest representatives with a known inner ear, such as Eritherium , Phosphatherium and Numidotherium from the Paleocene and Eocene , a slightly different structure can be reconstructed. Your cochlea has only one and a half turns and has a developed secondary lamina. Scientists therefore assume that the early proboscis were probably only sensitive to higher frequencies. Possibly with the Mammutidae , but at the latest with the advent of the relationship to the modern elephants, the inner ear known today was developed.
Chemical communication is also very important in the reproduction of elephants. Female animals emit pheromone signals that indicate the status of their rutting cycle and above all influence bulls in the musth . Musth occurs once a year in adult males. This is a hormone-controlled phase, characterized by a significant increase in testosterone . Externally, the musth has a strong ejection of scented secretions from the temporal gland . During the musth, bulls are very aggressive and there are fights for dominance over the mating privilege, which can end in fatal or serious injuries. The fact that the temporal gland was an external marker of the musth and thus a complex reproductive behavior also occurred in extinct forms was proven by ice mummies of the genus Mammuthus . For other representatives of the proboscis without soft tissue transmission, this can only be deduced to a limited extent. In some skeletal finds of the genus mammoth , bone damage in the form of broken ribs and the like can be detected, some of which caused the death of the respective individual and are comparable to injuries in today's elephant fights. According to further analyzes, the animals largely died in the late spring, i.e. at a certain time of the year, so that there may be an indication of the expression of hormone-controlled sexual behavior very early in the proboscis family tree. In Notiomastodon, a South American representative of the Gomphotheriidae, the musth probably also appeared, which is indicated by periodic growth anomalies on the tusk of a male individual, which began in early summer. The tusks of female individuals of the Mammutidae again show longer-lasting growth phases, which were interrupted about every three to four years. This coincides with the birth interval of the cows of today's elephants, which give birth to a calf every 4 to 8 years. The phased interruption of the growth spurt on the tusks of Mammut is explained by higher investment costs in the rearing of the offspring, for example through the production of breast milk , which means that important minerals for the formation of tusks were not available. The first onset of growth anomalies after around ten years corresponds to the sexual maturity of young female and male elephants.
nourishment
In general, trunk animals specialize in plant-based food. The variable design of the molars enabled the various representatives to develop numerous plant-based food sources. Today's elephants with their lamellodont teeth feed on soft plant components such as leaves , twigs , fruits or bark as well as on hard plant foods such as grasses . The respective proportion of the individual components varies with the season. The proportion of grass increases significantly, mainly during the rainy season. The mammoths , especially the woolly mammoth that lived in the arctic steppe landscapes, showed an even stronger grass and herb-based diet . Evidence of this is provided not only by the teeth with their extremely high number of lamellas, but also by the stomach contents and excrement remains of some well-preserved ice mummies in Siberia.
The grass-specialized way of life is a relatively modern development within the tribal history of the proboscis animals and goes hand in hand with the drying up of the climate and the resulting expansion of open steppe and savannah landscapes in the Miocene. In this context, there is not only the increasing number of lamellae on the elephant molars, but also the marked elevation of the tooth crowns, which made it possible to withstand the heavy abrasion when chewing the grass. Phylogenetically older proboscis lines mostly fed on a softer vegetable diet, which can be deduced from the chewing surfaces of the molars, among other things. Here the bunodont tooth patterns speak for a largely generalized vegetable diet , zygodont and lophodont teeth rather refer to foliage eaters. In addition, the low tooth crowns can be taken as an indication of such a preference. In addition to fossilized food residues, isotope analyzes and characteristic traces of abrasion on the teeth help to clarify the diet of extinct forms. The presumed preference for leaves and twigs based on the zygodont tooth pattern of the Mammutidae is confirmed by the various dung and intestinal contents of Mammut . These are dominated by remains of trees and shrubs as well as those of aquatic plants, but grasses are also included. Isotope tests provided a comparable result. Mixed coniferous forests served as preferred habitats. The most original representatives of the proboscis such as Barytherium and Moeritherium , on the other hand, lived mainly in swampy or water-rich areas and mainly ate aquatic plants, which was also determined on the basis of isotope analyzes.
Even before the Elephantidae, some forms of the Gomphotheriidae used the spreading open landscapes. However, their diet remained largely mixed, as their bunodont dentistry reveals. However, apparently individual members of the proboscis line switched to a more grass-based diet and anticipated the development of the elephants without, however, expressing their anatomical adaptations. Compared to the trilophodontic gomphotheria and some of the more recent tetralophodontic forms such as anancus , the early representatives of the Loxodonta and Mammuthus already had a significantly more flexible food intake within the modern elephant . This was determined through analyzes of the isotope ratios on teeth from the Langebaanweg site in south-western Africa. Possibly this adaptability ensured the survival of the genera until the end of the Pleistocene and beyond. A study on the diet of the forest-dwelling Stegodon in comparison to the early representatives of elephas in East Asia who used more open landscapes came to a similar result .
Ecological influences
The proboscis have a great influence on their immediate biotope . By debarking trees, eating leaves, kinking twigs and branches, tearing out bushes and small trees or splitting larger ones, today's elephants, for example, have a strong impact on the landscape. This allows you to open up closed areas, push back the edges of forests or keep open landscapes free. This is of great importance for the savannahs of East and South Africa. In addition, the animals transport seeds over significant distances and thus contribute to the spread of plants. But elephants also work on a smaller scale, in that their footsteps or their excrement create narrowly limited living and development spaces for other living beings. For this reason, elephants are considered ecosystem engineers . A similar function can also be assumed for extinct forms such as the mammoths of the mammoth steppe . It is possible that this behavior appeared very early in the proboscis family tree, which dates back to the early Miocene or even the Oligocene . At this point in time, the Deinotheriidae , among other things, were the first larger proboscis animals with very large tusks, whose traditional signs of wear and damage suggest that large areas of bark had been scraped off or that trees were splitting.
Systematics
External system
Internal systematics of Afrotheria according to Kuntner et al. 2011
|
The proboscis are an order within the superorder of the Afrotheria , which in turn represent one of the four main lines of the higher mammals . The Afrotheria are assigned to different groups whose area of origin is more or less on the African continent or who are among its original inhabitants. Their togetherness is based less on anatomical similarities, but mostly on the results of molecular genetic studies. Two large groups can be distinguished within the Afrotheria: the Paenungulata and the Afroinsectiphilia . The latter include the elephants and the tenre karts , and sometimes the aardvark is also assigned to them. The Paenungulata, on the other hand, include the proboscis, the manatees and the hyrax . In contrast to the Afrotheria as a whole group, the closer relationship of elephants, sirens and snakes finds support both genetically and morphologically and anatomically. However, the more precise relationships between the three groups are under discussion. On the one hand, the hyrax act as the sister group of the other two lines. In this case the elephants and manatees are incorporated into the common supergroup of the Tethytheria . Another view is that the hyrax and elephant are in a sister-group relationship, while the manatees take the position of the outer group. A third constellation is a closer bond between the hyrax and the manatee, with the elephants as sister taxons to both. According to molecular genetic studies, the origin of the Afrotheria lies in the Upper Cretaceous 90.4 to 80.9 million years ago. A good 15 million years later, the Paenungulata and Afroinsectiphilia separated from each other. The proboscis differentiated themselves only a little later. The genetic data obtained coincide relatively well with the fossil record , according to which the oldest representatives of the proboscis can be traced back to the Paleocene more than 60 million years ago.
Internal system
Internal systematics of the proboscis according to Cozzuol et al. 2012
|
The proboscis represent a relatively diverse group within the Afrotheria. Around 160 species are known today, including more than 130 from Africa, Asia and Europe, which are distributed over 50 genera . These, in turn, can be assigned to around a dozen different families . The vast majority of the known forms are extinct and are only known through fossil finds . Some of these were not discovered until after 2000, including important early forms such as Eritherium or Daouitherium . In addition to the elephants (Elephantidae) as the only surviving family with the three current species, the Stegodontidae , Gomphotheriidae , Mammutidae and Deinotheriidae are the best-known representatives of the family survived until the end of the Pleistocene . Very primitive members of the order, on the other hand, are incorporated into the Phosphatheriidae , the Numidotheriidae , the Moeritheriidae or the Barytheriidae .
There are different approaches to order the families of the proboscis on a higher systematic level, the morphology of the molars is often used as a basis. However, this division into individual large groups is not entirely clear, which mainly applies to the early representatives. The elephantiformes are relatively undisputed . These are defined by the tooth structure of the first two molars, which have three, four or more transverse ridges ( tri- , tetra- , pentalophodontic teeth). Some authors such as Jeheskel Shoshani separate from these the more primitive Plesielephantiformes , which are characterized by only two ridges on the first two permanent molars ( bilophodontic teeth). Both groups can be viewed as subordinates. The position of the Deinotheriidae within the Plesielephantiformes is problematic because their second molar has a bilophodontic structure, but the first one is trilophodontic . The former is possibly not an original, but a derived characteristic. For this reason, the Plesielephantiformes are classified by some scientists as paraphyletic . Other authors such as Emmanuel Gheerbrant differentiate the early proboscis according to the chewing surface structure of the molars. They separate a Lophodont group from a Bunodont group. The former include forms such as Barytherium , Numidotherium , Phosphatherium or Daouitherium , the latter such as Moeritherium and Saloumia . Arcanotherium, on the other hand, mediates between the two groups of forms through the more bunolophodontic teeth. According to its character form barytherium , the Lophodonte group is also referred to as the "barytherioid group". However, this does not seem to be a natural community either.
Within the elephantiformes, the elephantimorpha form a partial order in which all proboscis are united that have the characteristic of the horizontal change of teeth . It thus includes the Mammutidae, Gomphotheriidae, Stegodontidae and Elephantidae, while other groups such as the Palaeomastodontidae and Phiomiidae are outside. The transition is probably formed by the genus Eritrea from the Upper Oligocene of northeastern Africa, in which this special tooth exchange is documented for the first time in ancestral history. There are currently individual difficulties with the more recent phylogenetic forms. The Gomphotheriidae as a whole group (superfamily of the Gomphotherioidea) are most likely paraphyletic , since from a phylogenetic point of view they also include the Stegodontidae and the elephants as the youngest lines of development. In order to unite the Elephantidae, Stegodontidae and Gomphotheriidae as a monophyletic group, Jeheskel Shoshani and Pascal Tassy introduced the Elephantida as a parent taxon in 1998 with the Gomphotherioidea and Elephantoidea as members. At the same time, some tetralophodontic gomphotheria ( Anancus , Tetralophodon and others) were separated from the Gomphotherioidea and assigned to the Elephantoidea. The Elephantida are opposed to the Mammutida , in which only the Mammutidae family is listed.
The systematic structure presented here is largely based on the elaborations by Jeheskel Shoshani and Pascal Tassy, which both authors presented in 2005, but are based on long-term research. While the classification of the oldest proboscis appears to be problematic in general, some other authors such as Malcolm C. McKenna and Susan K. Bell 1997 subdivide the younger lines of development of the elephantimorpha only into the mammutoid and the elephantoid, the latter then including the gomphotheria, stegodont and elephant respectively at the family level. Another model was presented in 2010 by William J. Sanders , who here - but only with reference to African proboscis - only highlights the Elephantoidea, which in addition to the groups already mentioned also contain the mammoths.
Internal systematics of the proboscis according to Buckley et al. 2019 based on biochemical data
|
Molecular genetic and biochemical methods are becoming increasingly important in the systematic classification of proboscis. Already at the end of the 20th century, the anatomically based close bond between the recent elephants and the extinct mammoths could also be genetically proven, but with the preliminary result of a closer relationship between Mammuthus and Loxodonta , the group of African elephants. In later and often repeated analyzes the relationship then shifted towards a closer relationship between the mammoths and the genus Elephas , which includes the Asian elephant. According to these in-depth studies, the elephants, as the youngest link in the evolution of the proboscis, diversified in the late Miocene . First Loxodonta and Elephas split off from each other a good 7.6 million years ago, the separation of the latter and Mammuthus took place about 6.7 million years ago. Later other elephant representatives such as palaeoloxodon were included. This opened up a complex early history of the elephants, which probably included numerous hybridization events during the initial splitting. However, even in recent phylogenetic history, intermingling has often occurred, as demonstrated by individual genetic studies on North American mammoths. Also at the end of the 20th century, the genome of the mammoth genus, which is much more primitive than the elephants, could be sequenced. This first result and also subsequent investigations confirmed the long independent development of the Mammutida and the Elephantida as large groups of the Elephantimorpha, previously determined by fossil finds. Mammoth's separation from the line that led to today's elephants dates back to the late Oligocene around 26 million years ago. The data were also supported by collagen analyzes. A study published in 2019 provided insight into the genetic and biochemical relationships of Notiomastodon as a South American representative of the Gomphotheriidae for the first time . Contrary to the often postulated closer position of the gomphotheria and elephants, however, there was a closer relationship to the Mammutidae.
Overview of the families and genera of the proboscis
The structure is based on the arrangements by Shoshani and Tassy from 2005 and individual more recent representations, some of which are based on them. In the following, newly introduced genera and higher taxonomic groups as well as further systematic work are taken into account. Above all, the Gomphotheriidae, and here in particular the American strain, have been revised several times. The South American representatives received more attention.
- Order: Proboscidea Illiger , 1811
-
- Plesielephantiformes Shoshani , 2001
-
-
- Eritherium Gheerbrant , 2009
- Daouitherium Gheerbrant, Sudre, Cappetta, Iarochène, Amaghzaz & Bouya , 2002
- Saloumia Tabuce, Sarr, Adnet, Lebrun, Lihoreau, Martin, Sambou, Thiam & Hautier , 2020
- Family: Phosphatheriidae Gheerbrant, Sudre & Tassy , 2005
- Phosphatherium Gheerbrant, Sudre & Cappetta , 1996
- Superfamily: Moeritherioidea Andrews , 1906
-
- Family: Moeritheriidae Andrews , 1906
- Moeritherium Andrews , 1901
- Superfamily: Numidotherioidea Shoshani & Tassy , 1992
-
- Arcanotherium Delmer , 2009
- Omanitherium Seiffert, Nasir, Al-Harthy, Groenke, Kraatz, Stevens & Al-Savigh , 2012
- Family: Numidotheriidae Shoshani & Tassy , 1992
- Numidotherium Mahboubi, Ameur, Crochet & Jaeger , 1986
- Superfamily: Barytherioidea Andrews , 1906
-
- Family: Barytheriidae Andrews , 1906
- Barytherium Andrews , 1901
- Superfamily: Deinotherioidea Bonaparte , 1845
-
- Family: Deinotheriidae Bonaparte , 1841
-
- Subfamily: Chilgatheriinae Sanders. Kappelmann & Rasmussen , 2004
- Chilgatherium Sanders, Kappelman & Rasmussen , 2004
- Subfamily: Deinotheriinae Sanders, Kappelman & Rasmussen , 2004
- Deinotherium (+ Prodeinoterium ) Kaup , 1829
-
-
- Elephantiformes Tassy , 1988
-
-
- Hemimastodon Pilgrim , 1912
- Eritreaum Shoshani, Walter, Abraha, Berhe, Tassy, Sanders, Marchant, Libsekal, Ghirmai & Zinner , 2006
- Family: Palaeomastodontidae Andrews , 1906
- Palaeomastodon Andrews , 1901
- Family: Phiomiidae Kalandadze & Rautian , 1992
- Phiomia Andrews & Beadnell , 1902
-
- Elephantimorpha Tassy & Shoshani , 1997
-
- Mammutida Tassy & Shoshani , 1997
-
- Superfamily: Mammutoidea Hay , 1922
-
- Family: Mammutidae Hay , 1922
- Losodokodon Rasmussen & Gutiérrez , 2009
- Eozygodon Tassy & Pickford , 1983
- Miomastodon Osborn , 1922
- Zygolophodon Vacek , 1877
- Sinomammut Mothé, Avilla, Zhao, Xie & Sun , 2016
- Mammut Blumenbach , 1799
- Elephantida Tassy & Shoshani , 1997
-
- Superfamily: Gomphotherioidea Hay , 1922
-
- Family: Gomphotheriidae Hay , 1922
-
- Pediolophodon Lambert , 2007
- Subfamily: Choerolophodontinae Gaziry , 1976
- Choerolophodon Schlesinger , 1917
- Afrochoerodon Pickford , 2001
- Subfamily: Amebelodontinae Barbour , 1927
- Progomphotherium Pickford , 2003
- Archaeobelodon Tassy , 1984
- Afromastodon Pickford , 2003
- Protanancus Arambourg , 1945
- Serbelodon Frick , 1933
- Amebelodon Barbour , 1927
- Konobelodon Lambert , 1990
- Torynobelodon Barbour , 1929
- Eurybelodon Lambert , 2016
- Platybelodon Borissiak , 1928
- Aphanobelodon Wang, Deng, Ye, He & Chen , 2017
- Subfamily: Gomphotheriinae Hay , 1922
- Gomphotherium Burmeister , 1837
- Serridentinus Osborn , 1923
- Subfamily: Rhynchotheriinae Hay , 1922
- Eubelodon Barbour , 1912
- Rhynchotherium Falconer , 1868
- Stegomastodon Pohlig , 1912
- Cuvieronius Osborn , 1923
- Notiomastodon (+ Amahuacatherium , Haplomastodon ) Cabrera , 1929
- Gnathabelodon Barbour & Sternberg , 1935
- Blancotherium May , 2019
- Subfamily: Sinomastodontinae Wang, Jin, Deng, Wei & Yan , 2012
- Sinomastodon Tobien, Chen & Li , 1986
- Superfamily: Elephantoidea Gray , 1821
-
- Tetralophodon (+ Morrillia ) Falconer ; 1857
- Anancus Aymard , 1855
- Paratetralophodon Tassy , 1983
- Family: Stegodontidae Osborn , 1918
- Stegolophodon Pohlig , 1888
- Stegodon Falconer , 1847
- Selenotherium Mackaye, Brunet & Tassy , 2005
- Family: Elephantidae Gray , 1821
-
- Subfamily Stegotetrabelodontinae Aguirre , 1969
- Stegotetrabelodon Petrocchi , 1941
- Stegodibelodon Coppens , 1972
- Subfamily Elephantinae Gray , 1821
- Primelephas Maglio , 1970
- Loxodonta Anonymous , 1827
- Stegoloxodon Kretzoi , 1950
- Palaeoloxodon Matsumoto , 1924
- Elephas Linnaeus , 1758
- Mammuthus Brookes , 1828
The position of Khamsaconus within the proboscis is not clear. Known about a Milchprämolaren from the Lower Eocene of the Ouarzazate Basin in Morocco Fund was originally developed by Jean Sudre and colleagues in 1993 to Louisinidae ordered a well with the elephant-shrews more closely related group. The reference to the proboscis comes mainly from Emmanuel Gheerbrant , which other authors took over in part. Later a relationship with the sleepers was also considered.
Tribal history
Origins and evolution trends
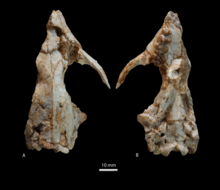
The proboscis are a relatively old order of mammals, the first representatives appeared in the Paleocene more than 60 million years ago. The origin of the group is not fully understood. However, there are some similarities with some " Condylarthra " -like ungulates of the early Paleogene Africa. Mention should be made here of Ocepeia from the Ouled Abdoun Basin in Morocco , which among other things shares the enlarged second and the reduced third upper incisor with the proboscis, as well as the air-filled cranial bones and the bilophodontic structure of the molars. The latter also applies to Abdounodus from the same find region. Structural differences in the formation of the humps, however, do not place both forms in the direct line of ancestors of the proboscis, but in the rather distant circle of relatives. Further skull analyzes, for example on the inner ear, show that the early representatives of the Paenungulata were phenomorphologically very similar and that greater differentiation only took place later. In the Eocene of today's South and Southeast Asia , the Anthracobunidae were widespread, which show some similarities to the proboscis through the structure of their teeth and feet. In contrast to most proboscis, however, there was also an anterior premolar. Partly the Anthracobunidae were carried out within the proboscis, today numerous researchers classify them as ancestral forms of the Tethytheria. According to another opinion, the Anthracobunidae are closer to the odd-toed ungulates .
Their diversity, the rich fossil record and the wide spatial and temporal distribution make the proboscis of great importance for biostratigraphy . The phylogenetic development can be roughly divided into three stages, combined with a respective diversification into numerous genera and species as well as adaptation to different ecological niches ( adaptive radiation ). General trends in the evolution of the proboscis are a marked increase in size - the oldest forms were less than a meter tall, while later forms reached up to more than 4 m shoulder height - enlargement of the skull, especially the roof of the skull as a starting point for a powerful neck and masticatory muscles, connected with the shortening of the jaw area, shortening of the neck area, formation of a trunk, hypertrophy of the respective second or first incisors with the formation of large tusks as well as the tendency to form large molars with simultaneous loss of the front premolars and largely also the front teeth as well the change in tooth exchange from vertical to horizontal, which is typical for mammals. It is also important that earlier proboscis were more leaf-eaters ( browser ), while the later forms were more specialized in grass food ( grazer ).
First radiation
The first radiation occurred 61 to about 24 million years ago and took place almost entirely in Africa and on the Arabian Peninsula , which was then connected to the continent. All previously known forms have been recorded from North Africa (including the Arabian Peninsula) and, to a lesser extent, from West Africa and East Africa . The representatives of these primal proboscis still had clearly bunodontic teeth with two transverse ridges on the first two and a maximum of four on the third molar, each with a high enamel cusp at the ends. Some forms also had a canine tooth per branch of the jaw. The vertical change of teeth that still occurs here is characteristic, so that all teeth were in use at the same time.
The oldest proboscis is currently considered to be Eritherium , which was first described in 2009 on the basis of individual skull fragments. It was an animal weighing only 3 to 8 kg that lived in northern Africa, where it has been recorded in the Ouled-Abdoun basin of Morocco. Characteristic of this representative are the poorly developed ridges on the chewing surface of the bunodontic molars, although a third ridge is already indicated on the last molar. From phosphatherium several teeth remains were also excavated in Ouled Abdoun-Basin since 1996th It lived about 55 million years ago and was barely bigger than a fox. Outwardly, the animals had little in common with later proboscis; their tooth structure, which was similar to that of Eritherium but has more developed ridges between the tooth cusps and thus has a tendency to lophodontia , suggests a close relationship. Numidotherium and Daouitherium , which are about the same age, show an even stronger tendency towards lophodontic tooth shapes . The former was documented with numerous skull and body skeleton parts in El-Kohol in Algeria , the latter in turn via a lower jaw in the Ouled-Abdoun basin. Moeritherium from the Eocene of North Africa was another early member of the proboscis. It was about the size of a tapir and had a pig-like head with an elongated upper lip and slightly elongated incisors in the upper and lower jaw. In addition to elephant features, the skull also bears common marks with that of the manatees . Furthermore, the genus is characterized by a very long body. With barytherium , the first enormous increase in body size within the proboscis line took place. The animals reached a shoulder height of 2.5 to 3 m and had a total of eight short tusks, two per jaw branch. Both forms are documented in a noteworthy number of finds from the Fayyum region in Egypt , the fossil remains there date from the transition from the Upper Eocene to the Lower Oligocene . With Moeritherium closely related is Saloumia , but of which so far only a molar tooth from the Senegal present. Due to the numerous forms of the Paleocene and Eocene described, the proboscis can point to one of the most complete fossil sequences from the early history of an order of the higher mammals.

The Deinotheriidae , which appeared for the first time in the course of the Oligocene and represent an early split, also belong to the first radiation phase . Characteristic for this group of proboscis are the tusks, which only appear in the lower jaw and curve downwards. They were used as tools for scraping off tree bark. The earliest representative, Chilgatherium from north-eastern Africa, was still relatively small, but so far only teeth have been recovered. In contrast, Deinotherium has a rich fossil record. The members of the genus continuously increased in size in the course of their tribal history and grew to over 4 m shoulder height, especially in the Pliocene and Pleistocene . In the Lower Miocene, with the closure of the Tethys and the creation of a land bridge to the north, the Deinotheriidae also immigrated to Eurasia . In Europe they became extinct in the course of the Pliocene, in Africa in the Lower Pleistocene around a million years ago. Due to their long existence and widespread distribution, the Deinotheriidae are one of the first successful groups of proboscis. From a research -historical point of view, the skull finds from Eppelsheim in Rhineland-Palatinate are important, as Deinotherium was first scientifically described here in 1829 and the position of the tusks was later correctly recognized. Some authors question whether the Deinotheriidae belong to the proboscis due to their tooth and dentition morphology and would like to see them more closely related to the manatees, but this view is only rarely shared.
Palaeomastodon and Phiomia were other very early species of proboscis from the Eocene and Oligocene of North Africa, primarily from the Fayyum. They also belong to the representatives of the first radiation, but are much more closely related to the later species of proboscis than to the earlier ones. At the moment there are still problems connecting the early forms with these two genera, since intermediate links are obviously still missing. Phiomia probably includesthe sister line to the later Gomphotheriidae (Gomphotheriidae), while Palaeomastodon represents that of the mammutids (Mammutidae). Differences between the two representatives can be found in tooth construction. So has phiomia three strips on the front molars, Palaeomastodon has, however, only the three lower, on the other hand the upper two.
The Mammutidae represent the last and one of the most important groups within the first radiation. According to molecular genetic studies, their line of development began at least 26 million years ago. The molars are zygodontic with a maximum of four enamel ridges on the last tooth. The special structure of the molars characterizes them as extensive leaf eater. Furthermore, these proboscis were characterized by two upper tusks, while older forms also had two smaller tusks in the lower jaw, which were only reduced in the course of further evolution and later lost. The trunk group has its origin in Africa. The oldest genus can be found here with the Losodokodon from the Upper Oligocene, which has only come down to us from Kenya on the basis of a few molars . Eozygodon has a more frequent record , which was also largely confined to Africa. The most important find material, with a fragmented partial skeleton, is from the sub-Miocene site of the Meswa Bridge, also in Kenya, from where the first description material comes. According to isolated indications, Eozygodon may also have appeared in Eurasia in the Middle Miocene , as the lower jaw of a non-adult animal from the Lengshuigou Formation in the Chinese province of Shaanxi suggests. In contrast, Zygolophodon, similar to the Deinotheriidae, has been passed down from Eurasia since the Lower Miocene, while the best-known genus, mammoth , was one of the first representatives of the proboscis to reach North America via North Asia . Here the American mastodon ( Mammut americanum ) formed, which lived at the same time as the representatives of the later genus Mammuthus and died out towards the end of the last glacial period in the Upper Pleistocene. Several species of mammoth have been recorded in Eurasia since the Upper Miocene . The best known is probably Mammut borsoni , a huge animal whose almost straight tusks are up to 5 m long and thus the longest among the proboscis. An almost complete skeleton is documented from Milia in Greece, among other places. The Eurasian line of Mammut largely disappeared again in the Pliocene and Lower Pleistocene. The generic name mammoth often leads to confusion, since its representatives are not closely related to the actual mammoth , whose generic name is Mammuthus .
Second radiation
The second radiation phase began in the Miocene. In the members of this group, the horizontal change of teeth is detectable for the first time. It should be emphasized, however, that the Mammutidae are mainly integrated into the first radiation phase, but the later representatives such as Mammut also have the characteristic of horizontal tooth change. The new mode of tooth replacement results in the shortening of the jawbones and the increasing complexity of the molars. This means that the molars become significantly larger, among other things, by increasing the number of ridges up to six. In addition, the basic bunodontic pattern of the occlusal surface continues to develop and lophodontic and, to a certain extent, zygodontic teeth are created. Possibly at the base of the second radiation is Eritrea from the late Oligocene of Northeast Africa. The remnants of the lower jaw found so far mediate in its tooth morphology between Phiomia or palaeomastodon and the later proboscis, but already shows the horizontal change of teeth. The most important groups of the second radiation phase are the Gomphotheriidae and the Stegodontidae , two families of proboscis which were originally combined with the Mammutidae to form the superfamily of the " mastodonts " (Mastodontoidea). From a historical research point of view, the “mastodons” shaped the second radiation phase, but today the term is only used as part of a generic name or colloquially for the American mastodon.

The Gomphotheriidae can be highlighted as the most important trunk line of the second radiation phase. This is also first detectable in Africa, but the relatives spread at the latest from the Lower Miocene a good 22 million years ago across Eurasia to North America. The Gomphotheriidae form one of the most successful groups within the proboscis, as they split up into numerous subgroups in the course of the cooling of the climate and the associated expansion of open landscapes in the Miocene. Today they combine almost half of all known taxa , which in turn can be divided into several subfamilies. In general, the Gomphotheriidae are proboscis with four tusks, two in the upper and two in the lower jaw. Another feature is the largely bunodontic , but varied tooth structure of the molars, with the milk teeth and the first two permanent molars having three enamel ridges - which is why they were originally called trilophodontic gomphotheria - while the last molar has four, five or more ribs. To subdivide the Gomphotheriidae, among other things, the tusks are used. Thus, the Gomphotheriinae in the upper row of teeth have two tusks clearly pointing downwards, while those of the lower jaw are elongated and flattened. Your relatives are among the basal forms of the entire family. Coming from Africa about 20 million years ago, the character form Gomphotherium reached large parts of Eurasia and a little later also crossed to North America. One of the most important finds is the skeleton of Gweng near Mühldorf am Inn east of Munich, which represents an almost complete individual around 3 m high. The Choerolophodontinae , restricted to Africa and Eurasia , on the other hand, have short mandibular tusks that are significantly reduced in length, while the Amebelodontinae such as Platybelodon from Asia and Amebelodon from North America are equipped with greatly elongated and widened, shovel-like modified mandibular tusks. The upper tusks in the Amebelodontinae are only small in size. This development goes so far that, in contrast to most proboscis, the upper tusks are completely lost, as shown by Aphanobelodon from eastern Asia. The Rhynchotheriinae in turn resemble the Gomphotheriinae, but have laterally flattened mandibular tusks. They form a partially American branch that emerges from the original Gomphotherium -like forms, a typical representative here is the Stegomastodon . In the further course and favored by the Great American Faun Exchange around 3 million years ago, they also colonize South America, where independent forms then develop. The South American gomphotheria differ from their relatives in Eurasia and North America by their comparatively short rostrum and the higher domed skull. This creates short-snouted ( brevirostrine ) forms from the once long- snouted ( longirostrine ) gomphotheria . The skull remodeling resulted from the largely complete loss of the lower tusks. The genera Notiomastodon and Cuvieronius , known from South America, are therefore sometimes classified in their own subfamily, the Cuvieroniinae. The Sinomastodontinae in East Asia are experiencing a similar development . Some of the Gomphotheriidae species survived into the late Pleistocene.
From the trilophodont gomphotheria the tetralophodontic forms developed, which had four enamel ridges on each of the milk teeth and the anterior permanent molars. Tetralophodon and anancus , which appear for the first time in Africa and Eurasia in the Middle and Upper Miocene, are important here . Their somewhat more modern skull features put both genera in closer relation to the Stegodontidae and Elephantidae and thus the superfamily of the Elephantoidea. Both genera are characterized by greatly reduced mandibular tusks, some of which are only pronounced in the deciduous dentition. The laterally offset inguinal structure of the molars is also characteristic of Anancus . Anancus is presumably a Eurasian descendant of Tetralophodon , which later immigrated back to Africa. Independent of this ancient line of development, gomphotheria-like forms with a higher number of enamel ridges evidently emerged in America, such as the late Miocene Pediolophodon from Nebraska, which only has four enamel folds on the second molar, but three on the first.
The second large group within the second radiation phase comprises the Stegodontidae, which developed in the Middle Miocene about 15 million years ago in East and Southeast Asia from gomphotheries with bunodont molars. The oldest form is stegolophodon . From this the later and dominant genus Stegodon emerged, which has typical ribbed molars consisting of up to nine ridges and sometimes high-crowned molars. As a rule, the stegodonts only have upper, closely set tusks, the lower ones are largely reduced or no longer developed in the shortened lower jaws. The proboscis group was widespread over a large part of Eurasia. The focus is to be found but in the eastern and southeastern Asia, here originated on some other islands such as on Flores also verzwergte forms. In the late Miocene and early Pliocene it also occurs in Africa, but remains a rare element of fauna there. America, however, did not reach the Stegodontidae.
Third radiation

The third phase of radiation began in the Upper Miocene 7 million years ago and includes the group of elephants, the only elephant family that has survived to this day. Significantly shortened and strongly arched skulls are typical of the animals. The molars are noticeably elongated and have a lamellar structure with a number of lamellae varying between eight and 30. The lamellae are flat and no longer raised as prominently as in the previous groups of proboscis. In the course of the radiation phase, not only does the tooth crown increase steadily, the number of lamellae also increases while the enamel thickness per lamella is reduced. These are typical adaptations to a diet that is increasingly dominated by grasses. The lower tusks are also largely regressed and the upper ones lack an enamel shell as a characteristic feature.
The Stegotetrabelodontinae are considered to be the more original group, which was largely only found in Africa from the late Miocene a little more than 7 million years ago to the Pliocene . The oldest form of the more modern Elephantinae is found Primelephas , which appears almost simultaneously in eastern and central Africa. It is followed by Mammuthus , Loxodonta and Palaeoloxodon at a relatively short time . A major problem with these early elephants is the fact that the found material, which has been handed down in a very fragmented manner, does not always allow a reliable differentiation. Loxodonta , the genus of today's African elephants, remains restricted to Africa throughout its tribal history, whereas Mammuthus and Palaeoloxodon also set foot on Eurasian soil around 3 million years ago. Here, independent development lines form out the best-known representatives are at Mammuthus the woolly mammoth ( Mammuthus primigenius ), in palaeoloxodon the European Forest Elephant ( palaeoloxodon antiquus ). There is a comprehensive fossil record for both species. From the former, in addition to individual finds through to entire “mammoth cemeteries”, there are also various ice mummies from the permafrost of North Asia, which have survived to this day thanks to the animals' adaptation to the Arctic climate . Among other things, several skeletal remains of the latter, some of them complete, came to light in the Geiseltal . Mammuthus was the only elephant form that migrated to America almost 2 million years ago, where it began to develop independently. Elephas and thus the genus of the Asian elephants, on the other hand, can be detected for the first time in the end of the Pliocene in South Asia. Generally speaking, the elephants are very large animals. With the steppe mammoth ( Mammuthus trogontherii ) and the prairie mammoth ( Mammuthus columbis ), some of the largest documented proboscis forms emerged, each with a shoulder height of around 4.5 m. In contrast, numerous islands in the Mediterranean and some in the Pacific harbored various dwarfed forms, with some pygmy elephants reducing their body size to such an extent that they are only around 2 to 7% of the size of the original forms.
Finale
In the Neogene , especially in the Pleistocene , there was a worldwide distribution of the proboscis to all continents, except Australia and Antarctica , with numerous species. This spread can only have taken place on the assumption of extensive migrations over land bridges, which were formed around 20 million years ago between Africa and Eurasia and around 3.5 million years ago between North and South America. With the widespread distribution of the proboscis during the Miocene, their diversity increased and numerous species and genera emerged. Adaptations to different landscape areas probably prevented too strong competition among one another. However, a first decline can already be seen in the late Miocene, around 8 million years ago. This is associated with the general cooling and stronger seasonalization of the climate as well as the associated spread of C 4 grasses . The changes resulted in a larger part of the habitats being less productive than before under warmer conditions and with the dominance of C 3 plants . The effect was then intensified around 3 million years ago with the gradual onset of the Ice Age , which led to the extinction of numerous lines of proboscis. Nevertheless, at the end of the Pleistocene, from 50,000 to around 12,000 years ago, there were still almost a dozen species of proboscis: mammoth , stegomastodon , notiomastodon , cuvieronius , stegodon , loxodonta , palaeoloxodon , elephas and mammothus . For most representatives of the proboscis during the approximately 60 million years of tribal history, an exact extinction scenario cannot be created due to insufficient finds, but the late Pleistocene forms offer this possibility due to the greater fossil occurrence. The disappearance is well documented, especially for Mammut , Notiomastodon and Mammuthus and to a certain extent also for Cuvieronius and Palaeoloxodon . While Loxodonta and Elephas exist to this day, some of the other proboscis died out in the transition from the Pleistocene to the Holocene . Mammuthus and Palaeoloxodon partially survived into the Middle Holocene. The extinction of most of the species of proboscis, but also of other large mammals up to the beginning of the Holocene, was possibly accompanied by the spread of modern humans ( Homo sapiens ). However, in addition to humans, the strong climatic fluctuations in the warm and cold phases of the last cold period and various other factors can also be possible causes. The reasons for the so-called Quaternary extinction wave , which may take a longer period of time, are subject to a strong scientific dispute. It is assumed that Cuvieronius had its last appearance in both North and South America before the arrival of humans and possibly died out in competition with other proboscis.
Research history
Taxonomy and Etymology
The genus Elephas had already been officially introduced in 1758 by Linnaeus (1707–1778) in his Systema Naturae, which was important for zoological nomenclature , but at that time included both Asian and African elephants. Frédéric Cuvier (1773-1838) then separated the genus Loxodonta in 1825 , although the name itself only found recognition through a publication two years later. In 1821, John Edward Gray (1800-1875) summarized the elephants in the family of the Elephantidae . Ten years before Gray, in 1811, Johann Karl Wilhelm Illiger (1775–1813) had used the term Proboscidea for the elephant, which is based on the most striking characteristic of the animals, the trunk. As a result, he identified them in German as "trunk animals". The name Proboscidea is derived from the Greek name προβοσκίς ( proboskis ), which is now generally translated as "trunk". The Greek prefix προ- ( pro- ) expresses a location (“to be in front of something”), the Greek word βοσκή ( boskḗ ) means something like “fodder” or “pasture” (as a verb βόσκειν ( bóskein ) “pasture” or "feed"). The proboscis as a functional organ is thus related to the intake of food. Other authors also simply translate proboscis as "in front of the mouth".
Pachyderms, ungulates and African animals - To the higher systematics
The systematic position of the elephants was assessed very differently over time. According to Linnaeus, they belonged to a group called Bruta, which included manatees , sloths and pangolins , and which was characterized by missing front teeth . At the end of the 18th century, Johann Friedrich Blumenbach moved the elephants to the group of Belluae, which Linnaeus had already conceived, and placed them alongside such different ungulates as tapirs , rhinos , hippos and pigs . He described the Bellue as large, clumsy animals with little body hair. For the further course of the 18th and 19th centuries, its compilation should prove to be the basic relationship between the proboscis animals. In 1795, Étienne Geoffroy Saint-Hilaire (1772–1844) and Georges Cuvier (1769–1832) combined them to form the pachydermata, to which Cuvier later added the peccaries , hyrax and some extinct forms. The pachydermata only lasted for a short period in the history of systematics, but they have a long resonance, since in a popular view their name as "pachyderms" survives to this day. But they were not the only attempts at structuring in the 19th century. Illiger himself had put the trunk animals into a unit called multungulata ("many-hoofed") in his paper from 1811, but which conceptually corresponded to the pachydermata. Gray, in turn, took over Illiger's organizational unit in 1821 and additionally set the elephant aside with the “ mastodons ”, an ancient group of elephants. He integrated the proboscis into a superordinate group called Quadripedes ("four-footed"), which included as an extremely broad group all quadruped mammals, in addition to the ungulates also the predators , rodents , insectivores , articular animals and others. The construct of the pachydermata, on the other hand, was first broken up in 1816 by Henri Marie Ducrotay de Blainville . In a table-like overview, he distinguished several groups of ungulates. De Blainville separated animals with an even number of toes ( onguligrades à doigts pairs ) from those with an odd number ( onguligrades à doigts impairs ). He included the elephants as the only members of a higher group called Gravigrades, but placed them on the side of the unpaired ungulates. More than three decades later, in 1848, took on Richard Owen 's approach de Blainvilles and established both the cloven-hoofed animals (Artiodactyla) and the perissodactyls (Perissodactyla), which he finally split the Pachydermata.
Unaffected by the splitting of the Pachydermata, the assumed relationship between the proboscis and the ungulates remained largely unchanged. In contrast, Theodore Gill noted in 1870 a closer bond between the manatees and the sleepers, although he did not give this relationship a special name. Other authors have given similar relationships with different names. Edward Drinker Cope , for example, used Taxeopoda in the 1880s and 1890s, while Richard Lydekker spoke of the Subungulata in the 1890s and Max Schlosser in the 1920s. Most of the terms used turned out to be problematic. Copes Taxeopoda originally contained ungulates with a serial foot structure (proboscis, hyrax and some extinct forms), which differed from the other ungulates with alternating foot structures (even-toed ungulates, odd-toed ungulates). He separated the latter as Diplarthra. In his final concept, however, he added the primates to the Taxeopoda. The Lydekker and Schlosser subungulata were similar in their composition to Cope's Taxeopoda, but the name had already been used by Illiger in 1811 for the guinea pig relatives . George Gaylord Simpson therefore established the Paenungulata in his general taxonomy of mammals in 1945 as a new superordinate group for the elephants, hyrax and manatees along with various extinct forms. Simpson saw the Paenungulata as part of the Protungulata. In contrast, in 1997 Malcolm C. McKenna and Susan K. Bell led the Paenungulata (named here as Uranotheria) including the elephants generally within the Ungulata.
In numerous systematics, the Paenungulata were considered more closely related to the odd-toed ungulates. With the increasing emergence of biochemical and molecular genetic investigation methods at the end of the 20th century , the perspective changed. As early as the early 1980s, protein analyzes indicated not only a close relationship between elephants, snakes and manatees, but also with the aardvark , which further investigations supported. In the mid-1990s, genetic tests then confirmed the homogeneity of the Paenungulata. These initial findings were followed by further analyzes in the transition to the 21st century. They revealed ultimately that the elephants, hyraxes and sea cows and aardvarks belong to a group that also tenrecs , golden moles and shrews includes. These are groups of animals originally distributed in Africa, the entire community was consequently referred to as Afrotheria . The results could be reproduced later, a further consolidation of the view resulted from the isolation of a specific retroposon , the so-called Afro SINE , which is common to all representatives of the Afrotheria.
"Mastodons" and Co. - For the internal structure
By the first third of the 19th century, all the important taxonomic units were named with the introduction of the genera Elephas and Loxodonta , the family of the Elephantidae and the order of the Proboscidea. Blumenbach identified the first fossil representative in 1799 with the mammoth , which was to be followed by numerous others in the course of the 19th century with Mammuthus 1828, Deinotherium 1829, Gomphotherium 1837, Stegodon 1847 or Anancus 1855. In the last years of the 18th century, Georges Cuvier, who was one of the first advocates of comparative anatomy , contrasted today's elephants with extinct representatives for the first time. The generic name “ Mastodon ”, which he officially established in 1817, goes back to Cuvier, and he had used it as early as 1806 as “ Mastodonte ” in a modified form . Within his new genus, Cuvier differentiated two species: " Mastodon " giganteum "and" Mastodon " angustidens . With the former, Cuvier referred to today's American mastodon, with the latter a form of gomphotherium . As a result, he united two, from today's point of view, not closely related proboscis forms under one genus, which are characterized on the one hand by a zygodont and on the other hand by a bunodont tooth pattern. The term “ mastodon ” became widely accepted in the next 150 years or more. Charles Frédéric Girard introduced the "Mastodontidae" family in 1852 (although Gray had already used the term "Mastodonadae" in 1821).
In the first three decades of the 20th century, Henry Fairfield Osborn (1857–1935) shaped research on the proboscis. The focus of his interest was above all the fossil remains, which were also the central subject of an expedition organized by him and carried out in the spring of 1907 by the American Museum of Natural History to the Fayyum area in Egypt. As a result of his research activities, he published several articles on the phylogeny of the proboscis in the 1920s and 1930s . His two-volume complete work The Proboscidea was published posthumously in 1936 and 1942 and forms a more than 1,600-page compilation of his research on the subject. Osborn distinguished over 350 species and subspecies in more than 40 genera and 8 families. The naming of the individual groups followed an Osborn principle and not according to the procedure common in zoology (such as the priority rule or the rule that family names are based on valid generic names). For example, he designated the Deinotheriidae with Curtognathidae and the Gomphotheriidae with Bunomastodontidae. In principle, however, Osborn identified four large groups of forms within the Proboscidea with the Moeritherioidea, Deinotherioidea, Mastodontoidea and Elephantoidea. Osborn saw the first two groups as the semi-aquatic original group, the latter two as forest and open land inhabitants. In the Mastodontoidea he united the zygodont "real mastodons" (Mammutidae) and the bunodont forms (Gomphotheriidae). In his opinion, the zygodont mastodonts came from Palaeomaston , the bunodonts, however, from Phiomia . Later, with his The Proboscidea Compendium, Osborn introduced the Stegodontoidea as the fifth large group, which he believed to be closely related to the zygodont mastodons and which he regarded as the original group of elephants. In addition, he underpinned the theory he had already developed in the transition from the 19th to the 20th century about an African origin of the proboscis, which he saw confirmed by the bone finds from the Eocene and Oligocene discovered in the Fayyum.
Only a few years later, however, George Gaylord Simpson rejected Osborn's scheme in his mammalian taxonomy. He criticized Osborn's failure to observe nomenclature rules. In his own attempt at structuring, he transferred Osborn's names into the common nomenclature and thereby also distinguished four large groups, to which he gave the names Moeritherioidea, Barytherioidea, Deinotherioidea and Elephantoidea. Within the latter, he led three families: He divided the mastodons into the Gomphotheriidae and the Mammutidae and placed them next to the Elephantidae, to which he assigned not only today's elephants, but also the stegodons. The Barytherioidea remained problematic in Simpson's eyes, which other authors sometimes classified as standing outside the proboscis.
In the further course of the 20th century, researchers such as John M. Harris and Vincent J. Maglio played a key role in researching elephants and their fossil relatives. In an overview of the African elephant fauna from 1978, they presented a revised system of order, which they largely limited to the elephantiformes and separated out more primitive groups such as those of the Moeritheria, Barytheria and Deinotheria. Within their “trunk” trunk animals, they differentiated the superordinate groups of the Mammutoidea and the Gomphotherioidea, with the former including the Mammutidae and Stegodontidae, the latter including the Gomphotheriidae and Elephantidae. In 1997 McKenna and Bell divided the Proboscidea largely in the classical sense and reintegrated the older groups such as Barytheria, Moeritheria and Deinotheria into the order. Like the authors before, they divided the younger groups into two lines, one of which included the Mammutoidea, the other the Elephantoidea, to which all other trunk animals belonged (gomphotheria, stegodonts and elephants). The systematic subdivision of proboscis animals, which is widely used today, goes back to Jeheskel Shoshani and Pascal Tassy and was published in 2005. Almost ten years earlier, the two scientists had already published a monograph together , which, like Osborn's work , is entitled The Proboscidea . In the following years, among others, William J. Sanders and colleagues carried out an extensive revision of the African proboscis in 2010. In the late 20th century and in the first two decades of the 21st century, Emmanuel Gheerbrant, among others, devoted himself to research into the earliest proboscis .
Proboscis and humans
In the late phase of the proboscid development and with the formation and development of humans, there were numerous interactions between the two groups. Initially, the proboscis and early humans settled common habitats, as has been proven at various sites of the Pleistocene in Africa and Eurasia. At least since the Middle Pleistocene, early humans increasingly used the proboscis as a source of food and raw materials. Clear evidence of the hunt is rather rare, but is evident, among other things, with the lance of Lehringen from the last warm period ( Eem warm period ), the shaft of which was stuck in the skeleton of a European forest elephant . The use of raw materials, on the other hand, can be demonstrated at numerous locations, mostly indicated by dismantled carcasses. This applies not only to the forms formerly native to Eurasia or Africa such as Mammuthus and Palaeoloxodon , numerous references are also documented from America and thus expand the spectrum to include genera such as Notiomastodon or Mammoth . Especially at the end of the last glacial period and with the emergence of Upper Palaeolithic art, trunk animals were included in the artistic work of man. Body parts of the animals were not only used in the production of objects for mobile small art , they themselves also served as models for a wide variety of representations, be it small sculptures or carvings in stone and bones or engravings and cave paintings on rock walls. The numerous mammoth portraits of the Franco-Cantabrian cave art are to be emphasized here .
To date, three species of elephants have survived. At least the African and Asian elephants can be ascribed a great local importance in art and culture, which is expressed in numerous representations on rock walls and in the case of the latter also in temple architecture and in the religious context. The Asian elephant is also the only representative in the permanent service of humans, but it is not a fully domesticated animal. The populations of the three elephant species living today are mainly due to hunting for the tusks and additionally due to the increasing destruction of habitat as a result of the spread of human settlements and Economic areas at risk.
literature
- Juan L. Cantalapiedra, Óscar Sanisidro, Hanwen Zhang, María T. Alberdi, José L. Prado, Fernando Blanco and Juha Saarinen: The rise and fall of proboscidean ecological diversity. Nature Ecology & Evolution, 2021, doi: 10.1038 / s41559-021-01498-w
- Ursula B. Göhlich: Order Proboscidea. In: Gertrud E. Rössner and Kurt Heissig: The Miocene land mammals of Europe. Munich, 1999, pp. 157-168.
- Jan van der Made: The evolution of the elephants and their relatives in the context of a changing climate and geography. In: Harald Meller (ed.): Elefantenreich. A fossil world in Europe. Halle / Saale, 2010, pp. 340-360.
- William J. Sanders, Emmanuel Gheerbrant, John M. Harris, Haruo Saegusa, and Cyrille Delmer: Proboscidea. In: Lars Werdelin and William Joseph Sanders (eds.): Cenozoic Mammals of Africa. University of California Press, Berkeley, London, New York, 2010, pp. 161-251.
Individual evidence
- ↑ a b c d e Jeheskel Shoshani: Skeletal and basic anatomical features of elephants. In: Jeheskel Shoshani and Pascal Tassy (eds.): The Proboscidea. Evolution and palaeoecology of the Elephants and their relatives. Oxford, New York, Tokyo, 1996, pp. 9-20.
- ↑ a b c d e f g G. Wittemyer: Family Elephantidae (Elephants). In: Don E. Wilson and Russell A. Mittermeier (Eds.): Handbook of the Mammals of the World. Volume 2: Hooved Mammals. Lynx Edicions, Barcelona 2011, ISBN 978-84-96553-77-4 , pp. 50-79.
- ↑ a b c d e f g h i Jeheskel Shoshani and Pascal Tassy (plus other authors): Order Proboscidea - Elephants. In: Jonathan Kingdon, David Happold, Michael Hoffmann, Thomas Butynski, Meredith Happold and Jan Kalina (eds.): Mammals of Africa Volume I. Introductory Chapters and Afrotheria. Bloomsbury, London 2013, pp. 173-200.
- ↑ Per Christiansen: Body size in proboscideans, with notes on elephant metabolism. Zoological Journal of the Linnean Society 140, 2004, pp. 523-549.
- ↑ a b c d e f g Emmanuel Gheerbrant: Paleocene emergence of elephant relatives and the rapid radiation of African ungulates. PNAS 106 (26), 2009, pp. 10717-10721.
- ↑ a b Asier Larramendi: Shoulder height, body mass, and shape of proboscideans. Acta Palaeontologia Polonica 61 (3), 2016, pp. 537-574
- ↑ Kathlyn M. Smith and Daniel C. Fisher: Sexual dimorphism of structures showing indeterminate growth: tusks of American mastodons (Mammut americanum). Paleobiology 37 (2), 2011, pp. 175-194.
- ↑ a b c d e f g h Jeheskel Shoshani and Pascal Tassy: Summary, conclusions, and a glimpse into the future. In: Jeheskel Shoshani and Pascal Tassy (eds.): The Proboscidea. Evolution and palaeoecology of the Elephants and their relatives. Oxford, New York, Tokyo, 1996, pp. 335-348.
- ↑ a b c d e f g h i j k l m Jeheskel Shoshani: Understanding proboscidean evolution: a formidable task. Tree 13, 1998, pp. 480-487.
- ^ Wighart von Koenigswald: Lebendige Eiszeit. Changing climate and wildlife. Theiss-Verlag, Stuttgart 2002, pp. 1-190 (pp. 42-53) ISBN 3-8062-1734-3
- ↑ a b c d e Ursula B. Göhlich: Order Proboscidea. In: Gertrud E. Rössner and Kurt Heissig: The Miocene land mammals of Europe. Munich, 1999, pp. 157-168.
- ^ A b William J. Sanders: Horizontal tooth displacement and premolar occurrence in elephants and other elephantiform proboscideans. Historical Biology 30 (1–2), 2018, pp. 137–156, doi: 10.1080 / 08912963.2017.1297436 .
- ↑ Ekke W. Günther: The teeth of the forest elephants of Bilzingsleben. In: Dietrich Mania (ed.): Bilzingsleben IV. Homo erectus - Its culture and its environment. Publications of the State Museum for Prehistory in Halle 44, Berlin 1991, pp. 149–174.
- ↑ a b c Shi-Qi Wang, Tao Deng, Jie Ye, Wen He and Shan-Qin Chen: Morphological and ecological diversity of Amebelodontidae (Proboscidea, Mammalia) revealed by a Miocene fossil accumulation of an upper-tuskless proboscidean. Journal of Systematic Palaeontology 15 (8), 2017, pp. 601-615 doi: 10.1080 / 14772019.2016.1208687 .
- ^ A b c Adrian M. Lister and Paul Bahn: Mammuts - The Giants of the Ice Age. Sigmaringen, 1997, pp. 1-168.
- ^ A b Dick Mol and Wilrie van Logchem: The Mastodon of Milia - the longest tusks in the world. Deposits 19, 2009, pp. 26-32.
- ↑ a b Cyrille Delmer: Reassessment of the generic attribution of Numidotherium savagei and the homologies of lower incisors in proboscideans. Acta Palaeontologica Polonica 54 (4), 2009, pp. 561-580.
- ↑ a b Jehezekel Shoshani, Robert M. West, Nicholas Court, Robert JG Savage and John M. Harris: The earliest proboscideans: general plan, taxonomy, and Palaeoecology. In: Jeheskel Shoshani and Pascal Tassy (eds.): The Proboscidea. Evolution and palaeoecology of the Elephants and their relatives. Oxford, New York, Tokyo, 1996, pp. 57-75.
- ^ W. David Lambert: The microstructure of proboscidean ivory and its application to the subordinal identification of isolated ivory specimens. Bulletin of the Florida Museum of Natural History 45 (4), 2005, pp. 521-530.
- ↑ Arun Banerjee: The mammoth ivory. In: Ulrich Joger and Ute Koch (eds.): Mammuts from Siberia. Darmstadt, 1994, pp. 38-42.
- ^ A b Sebastian J. Pfeifer, Wolfram L. Hartramph, Ralf-Dietrich Kahlke and Frank A. Müller: Mammoth ivory was the most suitable osseous raw material for the production of Late Pleistocene big game projectile points. Scientific Reports 9, 2019, p. 2303 doi: 10.1038 / s41598-019-38779-1 .
- ↑ Arun Banerjee: Comparative investigation of the "Schreger structure" on the tusks of elephants. Mainz Natural Science Archive 42, 2004, pp. 77–88.
- ^ Marie Albéric, Mason N. Dean, Aurélien Gourrier, Wolfgang Wagermaier, John WC Dunlop, Andreas Staude, Peter Fratzl and Ina Reiche: Relation between the Macroscopic Pattern of Elephant Ivory and Its Three-Dimensional Micro-Tubular Network. PLoS ONE 12 (1), 2017, p .e0166671, doi: 10.1371 / journal.pone.0166671 .
- ^ Marie Albéric, Aurélien Gourrier, Wolfgang Wagermaier, Peter Fratzl and Ina Reiche: The three-dimensional arrangement of the mineralized collagen fibers in elephant ivory and its relation to mechanical and optical properties. Acta Biomaterialia 72, 2018, pp. 342-351, doi: 10.1016 / j.actbio.2018.02.016 .
- ↑ Attila Virág: Histogenesis of the Unique Morphology of Proboscidean Ivory. Journal of Morphology 273, 2012, pp. 1406-1423.
- ^ David L. Fox: Growth increments in Gomphotherium tusks and implications for late Miocene climate change in North America. Palaeogeography, Palaeoclimatology, Palaeoecology 156, 2000, pp. 327-348.
- ^ Daniel C. Fisher, Michael D. Cherney, Cody Newton, Adam N. Rountrey, Zachary T. Calamari, Richard K. Stucky, Carol Lucking and Lesley Petrie: Taxonomic overview and tusk growth analyzes of Ziegler Reservoir proboscideans. Quaternary Research 82, 2014, pp. 518-532.
- ↑ a b c d Heinz Tobien: The structure of the mastodont molar (Proboscidea, Mammalia). Part 1: The bunodont pattern. Mainzer Geoswissenschaftliche Mitteilungen 2, 1973, pp. 115–147.
- ↑ a b c d Heinz Tobien: The structure of the mastodont molar (Proboscidea, Mammalia). Part 2: The zygodont and zygobundodont patterns. Mainzer Geoswissenschaftliche Mitteilungen 4, 1975, pp. 195–233.
- ↑ a b c d e Heinz Tobien: The structure of the mastodont molar (Proboscidea, Mammalia). Part 3: The Oligocene mastodont genera Palaeomastodon, Phiomia and the Eo / Oligocene paenungulate Moeritherium. Mainzer Geoscientific Mitteilungen 6, 1978, pp. 177–208.
- ↑ a b c D. Tab Rasmussen and Mercedes Gutiérrez: A Mammalian fauna from the Late Oligocene of Northwestern Kenya. Palaeontographica Department A 288 (1-3), 2009, pp. 1-52.
- ^ Pascal Tassy: Dental homologies and nomenclature in Proboscidea. In: Jeheskel Shoshani and Pascal Tassy (eds.): The Proboscidea. Evolution and palaeoecology of the Elephants and their relatives. Oxford, New York, Tokyo, 1996, pp. 21-25.
- ↑ a b c d Vincent J. Maglio: Origin and evolution of the Elephantidae. Transactions of the American Philosophical Society 63, 1973, pp. 1-149.
- ↑ Ralf-Dietrich Kahlke: The origin, development and distribution history of the Upper Pleistocene Mammuthus-Coelodonta Faunal Complex in Eurasia (large mammals). Treatises of the Senckenbergische Naturforschenden Gesellschaft 546, 1994, pp. 1-64.
- ↑ a b c d e f g h i j k William J. Sanders, Emmanuel Gheerbrant, John M. Harris, Haruo Saegusa and Cyrille Delmer: Proboscidea. In: Lars Werdelin and William Joseph Sanders (eds.): Cenozoic Mammals of Africa. University of California Press, Berkeley, London, New York, 2010, pp. 161-251.
- ^ HU Pfretzschner: Enamel microstructure and hyopsodonty in large mammals. In: Patricia Smith and Eitan Tchernov (Eds.): Structure, Function and Evolution of Teeth. London, 1992, pp. 147-162.
- ↑ Rodolphe Tabuce, Cyrille Delmer and Emanuel Gheerbrant: Evolution of the enamel microstructure in the earliest proboscideans. Zoological Journal of the Linnean Society 149, 2007, pp. 611-628.
- ↑ a b c d Jeheskel Shoshani, Robert C. Walter, Michael Abraha, Seife Berhe, Pascal Tassy, William J. Sanders, Gary H. Marchant, Yosief Libsekal, Tesfalidet Ghirmai and Dietmar Zinner: A proboscidean from the late Oligocene of Eritrea, a `` missing link '' between early Elephantiformes and Elephantimorpha, and biogeographic implications. PNAS 103 (46), 2006, pp. 17296-17301.
- ^ N. Court: Limb posture and gait in Numidotherium koholense, a primitive proboscidean from the Eocene of Algeria. Zoological Journal of the Linnean Society 111, 1994, pp. 297-338.
- ^ GE Weissengruber, FK Fuss, G. Egger, G. Stanek, KM Hittmair and G. Forstenpointner: The elephant knee joint: morphological and biomechanical considerations. Journal of Anatomy 208 (1), 2006, pp. 59-72.
- ↑ John R. Hutchinson, Cyrille Delmer, Charlotte E. Miller, Thomas Hildebrandt, Andrew A. Pitsillides and Alan Boyde: From Flat Foot to Fat Foot: Structure, Ontogeny, Function, and Evolution of Elephant “Sixth Toes”. Science 334 (6063), 2011, pp. 1699-1703, doi: 10.1126 / science.1211437 .
- ↑ Martin S. Fischer: The upper lip of the elephant. Zeitschrift für Mammaliankunde 52, 1987, pp. 262-263 ( [1] ).
- ↑ Martin S. Fischer and Uschi Trautmann: Fetuses of African elephants (Loxodonta africana) in photographs. Elephant 2, 1987, pp. 40-45.
- ↑ William P. Wall: Cranial evidence for a proboscis in Cadurcodon and a review of snout structure in the family Amynodontidae (Perissodactyla, Rhinocerotoidea). Journal of Paleontology 54 (5), 1980, pp. 968-977.
- ^ A b G. N. Markov, N. Spassov and V. Simeonovski: A reconstruction of the facial morphology and feeding behavior of the deinotheres. In: G. Cavarretta et al. (Eds.): The World of Elephants - International Congress. Consiglio Nazionale delle Ricerche. Rome, 2001, pp. 652-655.
- ↑ a b Ursula B. Göhlich: Tertiary primeval elephants from Germany. In: Harald Meller (ed.): Elefantenreich. A fossil world in Europe. Halle / Saale, 2010, pp. 362–372.
- ^ A b George A. Lyras: Brain Changes during Phyletic Dwarfing in Elephants and Hippos. Brain, Behavior and Evolution, 2019, doi: 10.1159 / 000497268 .
- ↑ Suzana Herculano-Huzel, Kamilla Avelino-de-Souza, Kleber Neves, Jairo Porfírio, Débora Messeder, Larissa Mattos Feijó, José Maldonado and Paul R. Manger: The elephant brain in numbers. Frontiers in Neuroanatomy 8, 2014, p. 46, doi: 10.3389 / fnana.2014.00046 .
- ↑ Anastasia S. Kharlamova, Sergei V. Saveliev, Albert V. Protopopov, Busisiwe C. Maseko, Adhil Bhagwandin, and Paul R. Manger: The Mummified Brain of a Pleistocene Woolly Mammoth (Mammuthus primigenius) Compared With the Brain of the Extant African Elephant (Loxodonta africana). The Journal of Comparative Neurology 523, 2015, pp. 2326-2343.
- ↑ Asier Larramendi and Maria Rita Palombo: Body Size, Biology and Encephalization Quotient of Palaeoloxodon ex gr. P. falconeri from Spinagallo Cave (Hyblean plateau, Sicily). Hystrix, the Italian Journal of Mammalogy 26 (2), 2015, pp. 102-109.
- ↑ Julien Benoit: A new method of estimating brain mass through cranial capacity in extinct proboscideans to account for the non-neural tissues surrounding their brain. Journal of Vertebrate Paleontology 35, 2015, p. E991021, doi: 10.1080 / 02724634.2014.991021 .
- ↑ Julien Benoit, Lucas J. Legendre, Rodolphe Tabuce, Theodor Obada, Vladislav Mararescul and Paul Manger: Brain evolution in Proboscidea (Mammalia, Afrotheria) across the Cenozoic. Scientific Reports 9, 2019, p. 9323, doi: 10.1038 / s41598-019-45888-4 .
- ↑ Polly K. Phillips and James Edward Heath: Heat exchange by the pinna of the African elephant (Loxodonta africana). Comparative Biochemy and Physiology 101 (4), 1992, pp. 693-699.
- ↑ Robin C. Dunkin, Dinah Wilson, Nicolas Way, Kari Johnson and Terrie M. Williams: Climate influences thermal balance and water use in African and Asian elephants: physiology can predict drivers of elephant distribution. The Journal of Experimental Biology 216, 2013, pp. 2939-2952.
- ↑ Moise Koffi, Yiannis Andreopoulos and Latif M. Jiji: The Role of pinnae flapping motion on Elephant Metabolic heat dissipation. Journal of Heat Transfer 136, 2014, p. 101101, doi: 10.1115 / 1.4027864 .
- ↑ António F. Martins, Nigel C. Bennett, Sylvie Clavel, Herman Groenewald, Sean Hensman, Stefan Hoby, Antoine Joris, Paul R. Manger and Michel C. Milinkovitch: Locally-curved geometry generates bending bending cracks in the African elephant skin. Nature Communications 9, 2018, p. 3865, doi: 10.1038 / s41467-018-06257-3 .
- ^ A. Valente: Hair structure of the Woolly mammoth, Mammuthus primigenius and the modern elephants, Elephas maximus and Loxodonta africana. Journal of Zoology 199 (2), 1983, pp. 271-274, doi: 10.1111 / j.1469-7998.1983.tb02095.x .
- ↑ OV Chernova, IV Kirillova, GG Boeskorov, FK Shidlovskiy and MR Kabilov: Architecture of the hairs of the woolly mammoth and woolly rhino. Proceedings of the Zoological Institute RAS 319 (3), 2015, pp. 441-460.
- ↑ Holger Römpler, Nadin Rohland, Carles Lalueza-Fox, Eske Willerslev, Tatyana Kuznetsova, Gernot Rabeder, Jaume Bertranpetit, Torsten Schöneberg and Michael Hofreiter: Nuclear gene indicates coat-color polymorphism in mammoths. Science 313 (5783), July 2006, p. 62, doi: 10.1126 / science.1128994 .
- ↑ Kimberley Sawtelle: Mastodont hair gives clues to habitat. Mammoth Trumpet 6 (4), 1991, p. 8.
- ↑ a b c Juan L. Cantalapiedra, Óscar Sanisidro, Hanwen Zhang, María T. Alberdi, José L. Prado, Fernando Blanco and Juha Saarinen: The rise and fall of proboscidean ecological diversity. Nature Ecology & Evolution, 2021, doi: 10.1038 / s41559-021-01498-w
- ↑ a b c d e f g h Jan van der Made: The evolution of the elephants and their relatives in the context of a changing climate and geography. In: Harald Meller (ed.): Elefantenreich. A fossil world in Europe. Halle / Saale, 2010, pp. 340-360.
- ↑ a b c d e f g María Teresa Alberdi, José Luis Prado, Edgardo Ortiz-Jaureguizar, Paula Posadas and Mariano Donato: Paleobiogeography of trilophodont gomphotheres (Mammalia: Proboscidea). A reconstruction applying DIVA (Dispersion-Vicariance Analysis). Revista Mexicana de Ciencias Geológicas 28 (2), 2011, pp. 235-244.
- ↑ a b c d Maria Rita Palombo: Elephants in miniature. In: Harald Meller (Ed.): Elefantenreich - Eine Fossilwelt in Europa. Halle / Saale 2010, pp. 275–295.
- ↑ Sevket Sen: A review of the Pleistocene dwarfed elephants from the Aegean islands, and their paleogeographic context. Fossil Imprint 73 (1/2), 2017; Pp. 76-92.
- ^ A b Athanassios Athanassiou, Alexandra AE van der Geer and George A. Lyras: Pleistocene insular Proboscidea of the Eastern Mediterranean: A review and update. Quaternary Science Reviews 218, 2019, pp. 306-321.
- ↑ Shermin de Silva and George Wittemyer: A Comparison of Social Organization in Asian Elephants and African Savannah Elephants. International Journal of Primatology 33 (5), 2012, pp. 1125-1141.
- ^ Faysal Bibi, Brian Kraatz, Nathan Craig, Mark Beech, Mathieu Schuster and Andrew Hill: Early evidence for complex social structure in Proboscidea from a late Miocene trackway site in the United Arab Emirates. Biology Letters 8 (4), 2012, pp. 670-673.
- ↑ Angela S. Stoeger, Gunnar Heilmann, Matthias Zeppelzauer, Andre Ganswindt, Sean Hensman and Benjamin D. Charlton: Visualizing sound emission of elephant vocalizations: evidence for two rumble production types. PLoS ONE 7 (11), 2012, p. E48907, doi: 10.1371 / journal.pone.0048907 .
- ↑ Angela S. Stoeger and Shermin de Silva: African and Asian Elephant Vocal Communication: A Cross-Species Comparison. In: G. Witzany (Ed.): Biocommunication of Animals. Springer Science & Media Press, 2014, pp. 21-39.
- ↑ Moti Nissani: Elephant Cognition: A Review of Recent Experiments. Gajah 28, 2008, pp. 44-52.
- ↑ Kaori Mizuno, Naoko Irie, Mariko Hiraiwa-Hasegawa and Nobuyuki Kutsukake: Asian elephants acquire inaccessible food by blowing. Animal Cognition 19 (1), 2016, pp. 215-222; doi: 10.1007 / s10071-015-0929-2.
- ↑ Nicholas Court: Cochlea anatomy of Numidotherium koholense: auditory acuity in the oldest known proboscidean. Lethaia 25 (2), 1992, pp. 211-215, doi: 10.1111 / j.1502-3931.1992.tb01385.x .
- ↑ a b Arnaud Schmitt and Emmanuel Gheerbrant: The ear region of earliest known elephant relatives: new light on the ancestral morphotype of proboscideans and afrotherians. Journal of Anatomy 228, 2016, pp. 137-152.
- ↑ Eric G. Ekdale: Morphological variation in the ear region of Pleistocene Elephantimorpha (Mammalia, Proboscidea) from Central Texas. Journal of Morphology 272, 2011, pp. 452-464.
- ↑ Julien Benoit, Samuel Merigeaud, Rodolphe Tabuce: Homoplasy in the ear region of Tethytheria and the systematic position of Embrithopoda (Mammalia, Afrotheria). Geobios 46, 2013, pp. 357-370.
- ↑ LEL Rasmussen and BA Schulte: Chemical signals in the reproduction of Asian (Elephas maximus) and African (Loxodonta africana) elephants. Animal Reproduction Science 53, 1998, pp. 19-34.
- ^ Daniel C. Fisher and David Fox: life history and unilateral loss of molar function in the Cohoes mastodon: A case study in nutritional stress? Journal of Vertebrate Paleontology 27 (3 suppl.), 2007, pp. 74A-75A.
- ^ Daniel C. Fisher: New ideas about old bones. American Paleontologist 16 (3), 2008, pp. 18-22.
- ^ Joseph J. El Adli, Daniel C. Fisher, Michael D. Cherney, Rafael Labarca and Frédéric Lacombat: First analysis of life history and season of death of a South American gomphothere. Quaternary International 443, 2017, pp. 180-188.
- ^ Daniel C. Fisher and David Fox: Calving histories of female mastodons (Mammut americanum). Journal of Vertebrate Paleontology 25 (3 suppl.), 2005, p. 57A.
- ↑ Bas van Geel, André Aptroot, Claudia Baittinger, Hilary H. Birks, Ian D. Bull, Hugh B. Cross, Richard P. Evershed, Barbara Gravendeel, Erwin JO Kompanje, Peter Kuperus, Dick Mol, Klaas GJ Nierop, Jan Peter Pals, Alexei N. Tikhonov, Guido van Reenen and Peter H. van Tienderen: The ecological implications of a Yakutian mammoth's last meal. Quaternary Research 69, 2008, pp. 361-376.
- ↑ Irina V. Kirillova, J. Argant, EG Lapteva, OM Korona, J. van derpflicht, EV Zinovyev, AA Kotov, OF Chernova, EO Fadeeva, OA Baturina, MR Kabilov, FA Shidlovskiy and OE Zanina: The diet and environment of mammoths in North-East Russia reconstructed from their content of the feces. Quaternary International 406, 2016, pp. 147-161.
- ↑ Lee A. Newsom and Mathew C. Mihlbachler: Mastodons (Mammut americanum) Diet Foraging Patterns Based on Analysis of Dung Deposits. In: S. David Webb (Ed.): First Floridians and Last Mastodons: The Page-Ladson Site in the Aucilla River. Springer, 2006, pp. 263-331.
- ↑ Hilary H. Birks, Bas van Geel, Daniel C. Fisher, Eric C. Grimm, Wim J. Kuijper, Jan van Arkel and Guido BA van Reenen: Evidence for the diet and habitat of two late Pleistocene mastodons from the Midwest, USA . Quaternary Research, 2018, pp. 1–21, doi: 10.1017 / qua.2018.100 .
- ^ Victor M. Bravo-Cuevas, Nuria M. Morales-García and Miguel A. Cabral-Perdomo: Description of mastodons (Mammut americanum) from the late Pleistocene of southeastern Hidalgo, central Mexico. Boletín de la Sociedad Geológica Mexicana 67 (2), 2015, pp. 337–347.
- ↑ Alexander GSC Liu, Erik R. Seiffert and Elwyn L. Simons: Stable isotope evidence for an amphibious phase in early proboscidean evolution. PNAS 105, 2008, pp. 5786-5791.
- ↑ Mark T. Clementz, Patricia A. Holroyd, Paul L. Koch: Identifying Aquatic Habits Of Herbivorous Mammals Through Stable Isotope Analysis. Palaios 23 (9), 2008, pp. 574-585.
- ↑ Ivan Calandra, Ursula B. Göhlich and Gildas Merceron: Feeding preferences of Gomphotherium subtapiroideum (Proboscidea, Mammalia) from the Miocene of Sandelzhausen (Northern Alpine Foreland Basin, southern Germany) through life and geological time: evidence from dental microwear analysis. Paläontologische Zeitschrift 84, 2010, pp. 205–215.
- ↑ Yan Wu, Tao Deng, Yaowu Hu, Jiao Ma, Xinying Zhou, Limi Mao, Hanweng Zhang, Jie Ye and Shi-Qi Wang: A grazing gomphothere in the Middle Miocene Central Asia, 10 million years prior to the origin of Elephantidae. Scientific Reports 8, 2018, p. 7640, doi: 10.1038 / s41598-018-25909 .
- ↑ Patricia A. Groenewald, Judith Sealy, Deano Stynder and Kathlyn M. Smith: Dietary resource partitioning among three coeval proboscidean taxa (Anancus capensis, Mammuthus subplanifrons, Loxodonta cookei) from the South African Early Pliocene locality of Langebaanweg E Quarry. Palaeogeography, Palaeoclimatology, Palaeoecology 543, 2020, p. 109606, doi: 10.1016 / j.palaeo.2020.109606 .
- ↑ Jiao Ma, Yuan Wang, Changzhu Jin, Yaowu Hu and Hervé Bocherens: Ecological flexibility and differential survival of Pleistocene Stegodon orientalis and Elephas maximus in mainland southeast Asia revealed by stable isotope (C, O) analysis. Quaternary Science Reviews 212, 2019, pp. 33-44.
- ↑ Ulrich Joger: Lifestyle and ecology of modern elephants: Are conclusions about the forest elephant and its environment possible? In: Harald Meller (Ed.): Elefantenreich - Eine Fossilwelt in Europa. Halle / Saale, 2010, pp. 314–321.
- ^ Margret Bunzel-Drüke, Joachim Drüke and Henning Vierhaus: The influence of large herbivores on the natural landscape of Central Europe. Working Group Biological Environmental Protection District Soest eV 2001 ( [2] ).
- ^ Friedrich Bachmayer and Helmuth Zapfe: An important find of Dinotherium from the Pannon of Lower Austria. Annals of the Natural History Museum in Vienna 80, 1976, pp. 145–162.
- ^ A b Matjaž Kuntner, Laura J. May-Collado and Ingi Agnarsson: Phylogeny and conservation priorities of afrotherian mammals (Afrotheria, Mammalia). Zoologica Scripta 40 (1), 2011, pp. 1-15.
- ↑ Rodolphe Tabuce, Laurent Marivaux, Mohammed Adaci, Mustapha Bensalah, Jean-Louis Hartenberger, Mohammed Mahboubi, Fateh Mebrouk, Paul Tafforeau and Jean-Jacques Jaeger: Early Tertiary mammals from North Africa reinforce the molecular Afrotheria clade. Proceedings of the Royal Society Series B 274, 2007, pp. 1159-1166.
- ^ A b Robert W. Meredith, Jan E. Janečka, John Gatesy, Oliver A. Ryder, Colleen A. Fisher, Emma C. Teeling, Alisha Goodbla, Eduardo Eizirik, Taiz LL Simão, Tanja Stadler, Daniel L. Rabosky, Rodney L. Honeycutt, John J. Flynn, Colleen M. Ingram, Cynthia Steiner, Tiffani L. Williams, Terence J. Robinson, Angela Burk-Herrick, Michael Westerman, Nadia A. Ayoub, Mark S. Springer and William J. Murphy: Impacts of the Cretaceous Terrestrial Revolution and KPg Extinction on Mammal Diversification. Science 334, 2011, pp. 521-524.
- ↑ a b c Mark S. Springer, William J. Murphy, Eduardo Eizirik and Stephen J. O'Brian: Placental mammal diversification and the Cretaceous-Tertiary boundary. PNAS 100 (3), 2003, pp. 1056-1061.
- ↑ Erik R. Seiffert: A new estimate for African phylogeny based on simultaneous analysis of genomic, morphological, and fossil evidence. BMC Evolutionary Biology 7, 2007, p. 224.
- ↑ Maureen A. O'Leary, Jonathan I. Bloch, John J. Flynn, Timothy J. Gaudin, Andres Giallombardo, Norberto P. Giannini, Suzann L. Goldberg, Brian P. Kraatz, Zhe-Xi Luo, Jin Meng, Xijun Ni, Michael J. Novacek, Fernando A. Perini, Zachary S. Randall, Guillermo W. Rougier, Eric J. Sargis, Mary T. Silcox, Nancy B. Simmons, Michelle Spaulding, Paúl M. Velazco, Marcelo Weksler, John R Wible and Andrea L. Cirranello: The Placental Mammal Ancestor and the Post-K-Pg Radiation of Placentals. Science 339, 2013, pp. 662-667.
- ^ A b Mario A. Cozzuol, Dimila Mothé and Leonardo S. Avilla: A critical appraisal of the phylogenetic proposals for the South American Gomphotheriidae (Proboscidea: Mammalia). Quaternary International 255, 2012, pp. 36-41.
- ↑ a b c Jeheskel Shoshani, WJ Sanders and Pascal Tassy: Elephants and other Proboscideans: a summary of recent findings and new taxonomic suggestions. In: G. Cavarretta et al. (Ed.): The World of Elephants - International Congress. Consiglio Nazionale delle Ricerche. Rome, 2001, pp. 676-679.
- ↑ a b c d e f g Jeheskel Shoshani and Pascal Tassy: Advances in proboscidean taxonomy & classification, anatomy & physiology, and ecology & behavior Quaternary International 126–128, 2005, pp. 5–20.
- ↑ Cyrille Delmer: Phylogenetic relationships between Paleogene proboscideans: New data and impact on tethytherian systematics. Journal of Vertebrate Paleontology 25 (3, suppl.), 2005, p. 50A.
- ↑ a b c Emmanuel Gheerbrant, Jean Sudre, Henri Cappetta, Mohamed Iarochène, Mbarek Amaghzaz and Baâdi Bouya: A new large mammal from the Ypresian of Morocco: Evidence of surprising diversity of early proboscideans. Acta Palaeontologica Polonica47 (3), 2002, pp. 493-506.
- ↑ a b c d Emmanuel Gheerbrant, Jean Sudre, Pascal Tassy, Mbarek Amaghzaz, Baâdi Bouya and Mohamed Iarochène: Nouvelles données sur Phosphatherium escuilliei (Mammalia, Proboscidea) de l'Éocène inférieur du Maroc, apports à la probosgulésie et des Probosgulésie des lophodontes. Geodiversitas 27 (2), 2005, pp. 239-333.
- ↑ a b Erik R. Seiffert, Sobhi Nasir, Abdulrahman Al-Harthy, Joseph R. Groenke, Brian P. Kraatz, Nancy J. Stevens and Abdul Razak Al-Sayigh: Diversity in the later Paleogene proboscidean radiation: a small barytheriid from the Oligocene of Dhofar Governorate, Sultanate of Oman. Natural Sciences 99, 2012, pp. 133-141, doi: 10.1007 / s00114-011-0878-9 .
- ↑ a b Jeheskel Shoshani, Edward M. Golenberg and Hong Yang: Elephantidae phylogeny: morphological versus molecular results. Acta Theriologica Suppl. 5, 1998, pp. 89-122.
- ↑ a b c Malcolm C. McKenna and Susan K. Bell: Classification of mammals above the species level. Columbia University Press, New York, 1997, pp. 1-631 (pp. 497-504).
- ↑ a b Michael Buckley, Omar P. Recabarren, Craig Lawless, Nuria García and Mario Pino: A molecular phylogeny of the extinct South American gomphothere through collagen sequence analysis. Quaternary Science Reviews 224, 2019, p. 105882, doi: 10.1016 / j.quascirev.2019.105882 .
- ↑ Erika Hagelberg, Mark G. Thomas, Charles E. Cook Jr., Andrei V. Sher, Gennady F. Baryshnikov and Adrian M. Lister: DNA from ancient mammoth bone. Nature 370, 1994, pp. 333-334.
- ↑ M. Noro, R. Masuda, IA Dubrovo, MC Yoshida and M. Kato: Molecular phylogenetic inference of the woolly mammoth Mammuthus primigenius, based on complete sequences of mitochondrial cytochrome b and 12S ribosomal RNA genes. Journal of Molecular Evolution 46 (3), 1998, pp. 314-326.
- ↑ Cristian Capelli, Ross DE MacPhee, Alfred L. Roca, Francesca Brisighelli, Nicholas Georgiadis, Stephen J. O'Brien and Alex D. Greenwood: A nuclear DNA phylogeny of the woolly mammoth (Mammuthus primigenius). Molecular Phylogenetics and Evolution 40, 2006, pp. 620-627, doi: 10.1016 / j.ympev.2006.03.015 .
- ↑ Evgeny I Rogaev, Yuri K Moliaka, Boris A Malyarchuk, Fyodor A Kondrashov, Miroslava V Derenko, Ilya Chumakov and Anastasia P Grigorenko: Complete mitochondrial genome and phylogeny of Pleistocene mammoth Mammuthus primigenius. PLoS Biology 4 (3), 2006, p. E73, doi: 10.1371 / journal.pbio.0040073 .
- ↑ a b c d Nadin Rohland, Anna-Sapfo Malaspinas, Joshua L. Pollack, Montgomery Slatkin, Paul Matheus and Michael Hofreiter: Proboscidean Mitogenomics: Chronology and Mode of Elephant Evolution Using Mastodon as Outgroup. PLoS Biology 5 (8), 2007, pp. 1663-1671, doi: 10.1371 / journal.pbio.0050207 .
- ↑ Nadin Rohland, David Reich, Swapan Mallick, Matthias Meyer, Richard E. Green, Nicholas J. Georgiadis, Alfred L. Roca and Michael Hofreiter: Genomic DNA Sequences from Mastodon and Woolly Mammoth Reveal Deep Speciation of Forest and Savanna Elephants. PLoS Biology 8 (12), 2010, p. E1000564, doi: 10.1371 / journal.pbio.1000564 .
- ↑ a b M. Buckley, N. Larkin and M. Collins: Mammoth and Mastodon collagen sequences; survival and utility. Geochimica et Cosmochimica Acta 75, 2011, pp. 2007-2016.
- ↑ Matthias Meyer, Eleftheria Palkopoulou, Sina Baleka, Mathias Stiller, Kirsty EH Penkman, Kurt W. Alt, Yasuko Ishida, Dietrich Mania, Swapan Mallick, Tom Meijer, Harald Meller, Sarah Nagel, Birgit Nickel, Sven Ostritz, Nadin Rohland, Karol Schauer, Tim Schüler, Alfred L Roca, David Reich, Beth Shapiro, Michael Hofreiter: Palaeogenomes of Eurasian straight-tusked elephants challenge the current view of elephant evolution. eLife Sciences 6, 2017, p. e25413, doi: 10.7554 / eLife.25413 .
- ↑ Eleftheria Palkopoulou, Mark Lipson, Swapan Mallick, Svend Nielsen, Nadin Rohland, Sina Baleka, Emil Karpinski, Atma M. Ivancevic, Thu-Hien To, R. Daniel Kortschak, Joy M. Raison, Zhipeng Qu, Tat-Jun Chin, Kurt W. Alt, Stefan Claesson, Love Dalén, Ross DE MacPhee, Harald Meller, Alfred L. Roca, Oliver A. Ryder, David Heiman, Sarah Young, Matthew Breen, Christina Williams, Bronwen L. Aken, Magali Ruffier, Elinor Karlsson , Jeremy Johnson, Federica Di Palma, Jessica Alfoldi, David L. Adelson, Thomas Mailund, Kasper Munch, Kerstin Lindblad-Toh, Michael Hofreiter, Hendrik Poinar and David Reich: A comprehensive genomic history of extinct and living elephants. PNAS 115 (11), 2018, pp. E2566 – E2574, doi: 10.1073 / pnas.1720554115 .
- ↑ Jacob Enk, Alison Devault, Regis Debruyne, Christine E. King, Todd Treangen, Dennis O'Rourke, Steven L. Salzberg, Daniel Fisher, Ross DE MacPhee and Hendrik Poinar: Complete Columbian mammoth mitogenome Suggests interbreeding with woolly mammoths. Genome Biology 12, 2011, p. R51, doi: 10.1186 / gb-2011-12-5-r51 .
- ↑ Jacob Enk, Alison Devault, Christopher Widga, Jeffrey Saunders, Paul Szpak, John Southon, Jean-Marie Rouillard, Beth Shapiro, G. Brian Golding, Grant Zazula, Duane Froese, Daniel C. Fisher, Ross DE MacPhee and Hendrik Poinar: Mammuthus population dynamics in Late Pleistocene North America: Divergence, Phylogeography and Introgression. Frontiers in Ecology and Evolution 4, 2016, p. 42, doi: 10.3389 / fevo.2016.00042 .
- ↑ Hong Yang, Edward M. Golenberg and Jeheskel Shoshani: Phylogenetic resolution within the Elephantidae using fossil DNA sequence from the American mastodon (Mammut americanum) as an outgroup. PNAS 93, 1996, pp. 1190-1194.
- ^ Ludovic Orlando, Catherine Hänni and Christophe J. Douady: Mammoth and elephant phylogenetic relationships: Mammut americanum, the missing outgroup. Evolutionary Bioinformatics 2, 2007, pp. 45-51.
- ^ A b W. David Lambert: New tetralophodont gomphothere material from American Tetralophodon. Journal of Vertebrate Paleontology 27 (3), 2007, pp. 676-682.
- ↑ Dimilla Mothé, Leonardo S. Avilla, Desi Zhao, Guangpu Xie and Boyang Sun: A new Mammutidae (Proboscidea, Mammalia) from the Late Miocene of Gansu Province, China. Anais da Academia Brasileira de Ciências 88 (1), 2016, pp. 65-74.
- ↑ W. David Lambert: Eurybelodon shoshanii to unusual new shovel-tusked gomphothere (Mammalia, Proboscidea) from the late Miocene of Oregon. Journal of Vertebrate Paleontology 36 (3), 2016, p. E1091352, doi: 10.1080 / 02724634.2016.1091352 .
- ↑ Steven R. May: The Lapara Creek Fauna: Early Clarendonian of south Texas, USA. Palaeontologia Electronica 22 (1), 2019, p. 22.1.15A (p. 1–129, here 42–62) doi: 10.26879 / 929 .
- ↑ a b Rodolphe Tabuce, Raphaël Sarr, Sylvain Adnet, Renaud Lebrun, Fabrice Lihoreau, Jeremy E. Martin, Bernard Sambou, Mustapha Thiam and Lionel Hautier: Filling a gap in the proboscidean fossil record: a new genus from the Lutetian of Senegal. Journal of Paleontology 94 (3), 2020, pp. 580-588, doi: 10.1017 / jpa.2019.98 .
- ↑ a b Wang Yuan, Jin ChangZhu, Deng ChengLong, Wei GuangBiao and Yan YaLing: The first Sinomastodon (Gomphotheriidae, Proboscidea) skull from the Quaternary in China. Chinese Science Bulletin 57 (36), 2012, pp. 4726-4734.
- ^ Mohammed Al-Kindi, Martin Pickford, Yusouf Al-Sinani, Ibrahim Al-Ismaili, Axel Hartman and Alan Heward: Large mammals from the Rupelian of Oman - Recent finds. Fossil Imprint 73 (3–4), 2017, pp. 300–321, doi: 10.2478 / if-2017-0017 .
- ↑ George E. Konidaris, ocrates J. Roussiakis, George E. Theodorou and George D. Koufus: The Eurasian Occurrence of the Shovel-Tusker Konobelodon (Mammalia, Proboscidea) as Illuminated by its Presence in the Late Miocene of Pikermi (Greece). Journal of Vertebrate Paleontology 34 (6), 2014, pp. 1437-1453.
- ↑ Shi-Qi Wang, Xiao-Xiao Zhang and Chun-Xiao Li: Reappraisal of Serridentinus gobiensis Osborn & Granger and Miomastodon tongxinensis Chen: the validity of Miomastodon. Vertebrata PalAsiatica 58 (2), 2020, pp. 134–158, doi: 10.19615 / j.cnki.1000-3118.200310 .
- ↑ Dimila Mothé, Leonardo S. Avilla, Mário Cozzuol and Gisele R. Winck: Taxonomic revision of the Quaternary gomphotheres (Mammalia: Proboscidea: Gomphotheriidae) from the South American lowlands. Quaternary International 276, 2012, pp. 2-7.
- ↑ Dimila Mothé, Leonardo S. Avilla and Mario A. Cozzuol: The South American Gomphotheres (Mammalia, Proboscidea, Gomphotheriidae): Taxonomy, Phylogeny, and Biogeography. Journal of Mammalian Evolution 20, 2013, pp. 23-32.
- ↑ Dimila Mothé and Leonardo Avilla: Mythbusting evolutionary issues on South American Gomphotheriidae (Mammalia: Proboscidea). Quaternary Science Reviews 110, 2015, pp. 23-35.
- ↑ Dimila Mothé, Marco P. Ferretti and Leonardo S. Avilla: Running Over the Same Old Ground: Stegomastodon Never Roamed South America. Journal of Mammalian Evolution 26 (2), 2019, pp. 165-177, doi: 10.1007 / s10914-017-9392-y .
- ^ Jean Sudre, Jean-Jacques Jaeger, Bernard Sigé and Monique Vianey-Liaud: Nouvelles données sur les Condylarthres du Thanétien et de l'Yprésien du bassin d'Ouarzazate (Maroc). Geobios 26 (5), 1993, pp. 609-615.
- ↑ a b c Emmanuel Gheerbrant: The oldest known proboscidean and the role of Africa in the radiation of modern orders of placentals. Bulletin of the Geological Society of Denmark 44, 1998, pp. 181-185.
- ↑ Emmanuel Gheerbrant, Mbarek Amaghzaz, Baadi Bouya, Florent Goussard and Charlène Letenneur: Ocepeia (Middle Paleocene of Morocco): The Oldest Skull of an Afrotherian Mammal. PLoS ONE 9 (1), 2014, p. E89739, doi: 10.1371 / journal.pone.0089739 .
- ↑ Emmanuel Gheerbrant, Andrea Filippo and Arnaud Schmitt: Convergence of Afrotherian and Laurasiatherian Ungulate-Like Mammals: First Morphological Evidence from the Paleocene of Morocco. PLoS ONE 11 (7), 2016, p. E0157556, doi: 10.1371 / journal.pone.0157556 .
- ↑ a b c Emmanuel Gheerbrant, Baadi Bouya and Mbarek Amaghzaz: Dental and cranial anatomy of Eritherium azzouzorum from the Paleocene of Marocco, earliest known proboscidean material. Palaeontographica, Department A 297 (5/6), 2012, pp. 151-183.
- ^ Neil A. Wells and Philip D. Gingerich: Review of Eocene Anthracobunidae (Mammalia, Proboscidea) with an new genus and species, Jozaria palustris, from the Kuldana Formation of Kohat (Pakistan). Contributions from the Museum of Paleontology, The University of Michigan 26 (2), 1983, pp. 117-139.
- ↑ Philip D. Gingerich, Donald E. Russell and Neil A. Wells: Astragalus of Anthracobune (Mammalia, Proboscidea) from the Early-Middle Eocene of Kashmir. Contributions from the Museum of Paleontology, The University of Michigan 28 (3), 1990, pp. 71-77.
- ↑ Léonard Ginsburg, Khadim Hussain Durrani, Akhtar Mohammad Kassi and Jean-Loup Welcomme: Discovery of a new Anthracobunidae (Tethytheria, Mammaiia) from the lower Eocene lignite of the Kach-Harnai Area in Baluchistan (Pakistan). Comptes rendus de l'Académie des sciences. Sciences de la Terre et des planètes 328, 1999, pp. 209-213.
- ↑ Stéphane Ducrocq, Aung Naing Soe, Bo Bo, Mouloud Benammi, Yaowalak Chaimanee, Than Tun, Tin Thein and Jean-Jacques Jaeger: First record of an Anthracobunidae (Mammalia,? Tethytheria) from the Eocene of the Pondaung Formation, Myanmar. Comptes rendus de l'Académie des sciences. Sciences de la Terre et des planètes 330, 2000, pp. 725-730.
- ↑ Kenneth D. Rose, Luke T. Holbrook, Kishor Kumar, Rajendra S. Rana, Heather E. Ahrens, Rachel H. Dunn, Annelise Folie, Katrina E. Jones and Thierry Smith: Anatomy, Relationships, and Paleobiology of Cambaytherium (Mammalia , Perissodactylamorpha, Anthracobunia) from the lower Eocene of western India. Journal of Vertebrate Paleontology 39 (suppl.), 2020, pp. 1-147, doi: 10.1080 / 02724634.2020.1761370 .
- ^ A b Emmanuel Gheerbrant, Jean Sudre and Henry Cappetta: A Palaeocene proboscidean from Morocco. Nature 383, 1996, pp. 68-70.
- ↑ Mohammad Mahboubi, R. Ameur, Jean-Yves Crochet and Jean-Jacques Jaeger: Earliest known proboscidean from early Eocene of north-west Africa. Nature 308, 1984, pp. 543-544.
- ^ William Sanders, John Kappelmann and D. Tab Rassmussen: New large-bodied mammals from the late Oligocene site of Chilga, Ethiopia. Acta Palaeontologica Polonica 49 (3), 2004, pp. 365-392.
- ↑ Kati Huttunen: Systematics and Taxonomy of the European Deinotheriidae (Proboscidea, Mammalia). Annalen des. Naturhistorisches Museum zu Wien 103 A, 2002, pp. 237–250.
- ^ HJ Gregor, R. Kuhn and DH Storch: Deinotherium? a proboscidian? Documenta Naturae 130, 2000, pp. 1-141.
- ↑ Kati Huttunen and Ursula Bettina Göhlich: A partial skeleton of Prodeinotherium bavaricum (Proboscidea, Mammalia) from the Middle Miocene of Unterzolling (Upper Freshwater Molasse, Germany). Geobios 35, 2002, pp. 489-514.
- ↑ Pascal Tassy and Martin Pickford: Un nouveau mastodonte zygolophodonte (Proboscidea, Mammalia) dans le Miocène inférieur d'Afrique orientale: Systématique et paleoenvironnement. Geobios 16 (1), 1983, pp. 53-77.
- ↑ Xiaoxiao Zhang and Shiqi Wang: First record of Eozygodon (Mammutidae, Proboscidea) in Eurasia. Historical Biology, 2020, doi: 10.1080 / 08912963.2020.1723579 .
- ↑ Shiqi Wang, Yu Li, Jaroon Duangkrayom, Shao-Kun Chen, Wen He and Shan-Qin Chen: Early Mammut from the Upper Miocene of northern China, and ist implications for the evolution and differentiation of Mammutidae. Vertebrata Palasiatica 55 (3), 2017, pp. 233-256.
- ↑ Heinz Tobien: Evolution of the zygodons with emphasis of dentition. In: Jeheskel Shoshani and Pascal Tassy (eds.): The Proboscidea. Evolution and palaeoecology of the Elephants and their relatives. Oxford, New York, Tokyo, 1996, pp. 76-84.
- ^ A b Henry Fairfield Osborn: The Evolution, Phylogeny, and Classification of the Mastodontoidea. Bulletin of the Geological Society of America 32 (3), 1921, pp. 327-332.
- ↑ Pascal Tassy: Who is who among the Proboscidea? In: Jeheskel Shoshani and Pascal Tassy (eds.): The Proboscidea. Evolution and palaeoecology of the Elephants and their relatives. Oxford, New York, Tokyo, 1996, pp. 40-48.
- ↑ María Teresa Alberdi, José Luis Prado, Edgardo Ortiz-Jaureguizar, Paula Posadas and Mariano Donato: Historical biogeography of trilophodont Gomphotheres (Mammalia, Proboscidea) reconstructed applying dispersion vicariance analysis. In: E. Díaz-Martínez and I. Rábano (Eds.): 4th European Meeting on the Palaeontology and Stratigraphy of Latin America. Cuadernos del Museo Geominero, nº 8. Instituto Geológico y Minero de España, (Madrid) 2007, pp. 9-14.
- ↑ Ursula B. Göhlich: Elephantoidea (Proboscidea, Mammalia) from the Middle and Upper Miocene of the Upper Freshwater Molasse of Southern Germany: Odontology and Osteology. Munich Geoscientific Treatises Series A 36, Munich, 1998.
- ↑ Pascal Tassy, Pairote Anupandhanant, Léonard Ginsburg, Pierre Meine, Benjavun Ratanasthien and Varaviudth Sutteethorn: A new Stegolophodon (Proboscidea, Mammalia) from the Early Miocene of Northern Thailand. Geobios 25 (4), 1992, pp. 511-523.
- ↑ Jon E. Kalb, David J. Froehlich and Gordon L. Bell: Palaeobiogeography of late Neogene African and Eurasian Elephantoidea. In: Jeheskel Shoshani and Pascal Tassy (eds.): The Proboscidea. Evolution and palaeoecology of the Elephants and their relatives. Oxford, New York, Tokyo, 1996, pp. 117-123.
- ^ William J. Sanders: Oldest Record of Stegodon (Mammalia: Proboscidea). Journal of Vertebrate Paleontology 19 (4), 1999, pp. 793-797.
- ↑ Haruo Saegusa: Stegodontidae: evolutionary relationships. In: Jeheskel Shoshani and Pascal Tassy (eds.): The Proboscidea. Evolution and palaeoecology of the Elephants and their relatives. Oxford, New York, Tokyo, 1996, pp. 178-190.
- ↑ Vincent J. Maglio: Four new species of Elephantidae from the Plio-Pleistocene of northwestern Kenya. Breviora 341, 1970, pp. 1-43
- ^ Adrian M. Lister and Hans van Essen: The earliest Mammoth in Europe. Abstracts of the 18th International Senckenberg Conference April 25-30, 2004 in Weimar.
- ↑ AE Basilyan, MA Anisimov, PA Nikolskiy and VV Pitulko: Wooly mammoth mass accumulation next to the Paleolithic Yana RHS site, Arctic Siberia: ist geology, age, and relation to past human activity. Journal of Archaeological Science 38, 2011, pp. 2461-2474.
- ↑ Federica Marano and Maria Rita Palombo: Population structure in straight-tusked elephants: a case study from Neumark Nord 1 (late Middle Pleistocene ?, Sachsen-Anhalt, Germany). Bollettino della Società Paleontologica Italiana 52 (3), 2013, pp. 207-218.
- ^ A b José Luis Prado, Joaquín Arroyo-Cabrales, Eileen Johnson, María Teresa Alberdi and Oscar J. Polaco: New World proboscidean extinctions: comparisons between North and South America. Archaeological and Anthropological Sciences 7, 2015, pp. 277-288, doi: 10.1007 / s12520-012-0094-3 .
- ↑ Grant D. Zazula, Ross DE MacPhee, Jessica Z. Metcalfe, Alberto V. Reyes, Fiona Brock, Patrick S. Druckermiller, Pamela Groves, C. Richard Harington, Gregory WL Hodgins, Michael L. Kunz, Fred J. Longstaffe, Daniel H. Mann, H. Gregory McDonald, Shweta Nalawade-Chavan and John R. Southon: American mastodon extirpation in the Arctic and Subarctic predates human colonization and terminal Pleistocene climate change. PNAS 111 (52), 2014, pp. 18460-18465, doi: 10.1073 / pnas.1416072111 .
- ^ Anthony J. Stuart, Leopold D. Sulerzhitsky, Lyubov A. Orlova, Yaroslav V. Kuzmin and Adrian M. Lister: The latest woolly mammoths (Mammuthus primigenius Blumenbach) in Europe and Asia: a review of the current evidence. Quaternary Science Reviews 21, 2002, pp. 1559-1569.
- ^ Yaroslav V. Kuzmin: Extinction of the woolly mammoth (Mammuthus primigenius) and woolly rhinoceros (Coelodonta antiquitatis) in Eurasia: Review of chronological and environmental issues. Boreas 39, 2010, pp. 247-261.
- ^ Adrian M. Lister: Mammoths in miniature. Nature 362, 1993, pp. 288-289.
- ↑ Gregory James Smith and Larisa RG DeSantis: Extinction of North American Cuvieronius (Mammalia: Proboscidea: Gomphotheriidae) driven by dietary resource competition with sympatric mammoths and mastodons. Paleobiology 46 (1), 2020, pp. 41-57, doi: 10.1017 / pab.2020.7 .
- ^ A b Carl von Linné: Systema naturae. 10th edition, 1758, volume 1, p. 33 ( [3] ).
- ^ Frédéric Cuvier: Eléphant d'Afrique. In: Étienne Geoffroy Saint-Hilaire, Fréderic Cuvier (ed.): Histoire naturelle des mammifères: avec des figures originales, coloriées, dessinées d'après des animaux vivans. Tome sixième. Paris 1825 ( [4] ).
- ↑ Anonymous: Histoire naturelle des mammifères: avec des figures originales, coloriées, dessinées d'après des animaux vivans; & c. by MM. Geoffroy Saint-Hilaire and F. Cuvier. Livraisons 52de et 53eme. The Zoological Journal 3, 1827, pp. 140-142 ( [5] ).
- ^ A b c John Edward Gray: On the natural arrangement of vertebrose animals. London Medical Repository 15, 1821, pp. 297-310 (305) ( [6] ).
- ^ Johann Karl Wilhelm Illiger: Prodromus systematis mammalium et avium additis terminis zoographicis utriudque classis. Berlin, 1811, pp. 1-301 (p. 96) ( [7] ).
- ^ Antoni V. Milewski and Ellen S. Dierenfeld: Structural and functional comparison of the proboscis between tapirs and other extant and extinct vertebrates. Integrative Zoology 8, 2013, pp. 84-94.
- ↑ Merlin Peris: Aristotle's Notices on the Elephant. Gajah 22, 2003, pp. 71-75.
- ^ A b Johann Friedrich Blumenbach: Handbook of Natural History. Göttingen, 1779, pp. 1–448 (pp. 130–133) ( [8] ), Handbuch der Naturgeschichte. Göttingen, 1797, pp. 1–714 (p. 125) ( [9] ) and Handbuch der Naturgeschichte. Göttingen, 1799, pp. 1-708 (pp. 697-698) ( [10] ).
- ↑ Étienne Geoffroy Saint-Hilaire and Georges Cuvier: Mémoire sur une nouvelle division des Mammifères, et sur les principes qui doivent servir de base dans cette sorte de travail. Magasin Encyclopedique 2, 1795, pp. 164-190 (pp. 178, 189) ( [11] ).
- ^ A b Georges Cuvier: Le regne animal distribue d'apres son organization pour servir de base a l'histoire naturelle des animaux et d'introduction a l'anatomie comparee. (Volume 1) Paris, 1817, pp. 1-540 (pp. 227-242) ( [12] ).
- ^ Henri Marie Ducrotay de Blainville: Prodrome d'une nouvelle distribution systèmatique du règne animal. Bulletin des Sciences 3 (3), 1816, pp. 105-124 ( [13] ).
- ↑ Richard Owen: Description of teeth and portions of jaws of two extinct anthrocotherioid quadrupeds (Hyopotamus vectianus and Hyop. Bovinus) discovered by the Marchioness of Hastings in the Eocene deposits on the NW coast of the Isle of Wight: with an attempt to develope Cuvier's idea of the classification of pachyderms by the number of their toes. The Quarterly journal of the Geological Society of London 4, 1848, pp. 103-141 ( [14] ).
- ↑ a b c d George Gaylord Simpson: The Principles of Classification and a Classification of Mammals. Bulletin of the American Museum of Natural History 85, 1945, pp. 1-350 (pp. 243-250).
- ^ Theodore Gill: On the relations of the orders of mammals. Proceedings of the American Association for the Advancement of Science, 19th meeting, 1870, pp. 267-270 ( [15] ).
- ↑ Wilfried W. de Jong, Anneke Zweers and Morris Goodman: Relationship of aardvark to elephants, hyraxes and sea cows from α-crystallin sequences. Nature 292, 1981, pp. 538-540.
- ↑ Malcolm C. McKenna: The alpha crystallin A chain of the eye lens and mammalian phylogeny. Annales Zoologice Fennici 28, 1992, pp. 349-360.
- ↑ Anne Lavergne, Emmanuel Douzery, Todd Stichler, François M. Catzeflis and Mark S. Springer: Interordinal Mammalian Relationships: Evidence for Paenungulate Monophyly Is Provided by Complete Mitochondrial 12S rRNA Sequences. Molecular Phylogenetics and Evolution 6 (2), 1996, pp. 245-258.
- ↑ Ole Madsen, Peter MT Deen, Graziano Pesole, Cecilia Saccone and Wilfried W. de Jong: Molecular Evolution of Mammalian Aquaporin-2: Further Evidence that Elephant Shrew and Aardvark Join the Paenungulate Clade. Molecular Biology and Evolution 14 (4), 1997, pp. 363-371.
- ↑ Mark S. Springer, Gregory C. Cleven, Ole Madsen, Wilfried W. de Jong, Victor G. Waddell, Heather M. Amrine, and Michael J. Stanhope: Endemic African mammals shake the phylogenetic tree. Nature 388, 1997, pp. 61-64.
- ↑ Michael J. Stanhope, Victor G. Waddell, Ole Madsen, Wilfried de Jong, S. Blair Hedges, Gregory C. Cleven, Diana Kao and Mark S. Springer: Molecular evidence for multiple origins of Insectivora and for a new order of endemic African insectivore mammals. PNAS 95, 1998, pp. 9967-9972.
- ↑ Michael J. Stanhope, Ole Madsen, Victor G. Waddell, Gregory C. Cleven, Wilfried W. de Jong and Mark S. Springer: Highly Congruent Molecular Support for a Diverse Superordinal Clade of Endemic African Mammals. Molecular Phylogenetics and Evolution 9 (3), 1998, pp. 501-508.
- ↑ Masato Nikaido, Hidenori Nishihara, Yukio Hukumoto and Norihiro Okada: Ancient SINEs from African Endemic Mammals. Molecular Biology and Evolution 20 (4), 2003, pp. 522-527.
- ↑ Hidenori Nishihara, Yoko Satta, Masato Nikaido, JGM Thewissen, Michael J. Stanhope and Norihiro Okada: A Retroposon Analysis of Afrotherian Phylogeny. Molecular Biology and Evolution 22 (9), 2005, pp. 1823-1833.
- ↑ Georges Cuvier: Mémoire sur les espèces l'éléphans vivantes et fossiles. Mémoires de l'Institut des Sciences et Arts 2, 1799, pp. 1-23 ( [16] ).
- ^ Georges Cuvier: Sur le grand mastodonte. Annales du Muséum d'histoire Naturelle, 1806, pp. 270-285 ( [17] ).
- ^ Charles Girard: On the classification of Mammalia. Proceedings of the American Association for the Advancement of Science 6, 1852, pp. 319-335 ( [18] ).
- ^ Henry Fairfield Osborn: The Fayum Expedition of the American Museum. Science 25 (639), 1907, pp. 513-516.
- ^ Henry Fairfield Osborn: Hunting the ancestral elephant in the Fayum desert. Century Magazine 74, 1907, pp. 815-835 ( [19] ).
- ^ Henry Fairfield Osborn: Adaptive radiation and classification of the Proboscidea. PNAS 7, 1921, pp. 231-234.
- ^ Henry Fairfield Osborn: Evolution and Geographic Distribution of the Proboscidea: Moeritheres, Deinotheres and Mastodonts. Journal of Mammalogy 15 (3), 1934, pp. 177-184.
- ^ Henry Fairfield Osborn: The Proboscidea. New York, Part 1. 1936, pp. 1-802 ( [20] ) and Part 2. 1942, pp. 805-1675 ( [21] ).
- ^ Yves Coppens, Vincent J. Maglio, Cary T. Madden and Michel Beden: Proboscidea. In: Vincent J. Maglio and HBS Cooke (Eds.): Evolution of African Mammals. Harward University Press, 1978, pp. 336-367.
- ↑ John M. Harris: Deinotherioidea and Barytherioidea. In: Vincent J. Maglio and HBS Cooke (Eds.): Evolution of African Mammals. Harward University Press, 1978, pp. 315-332.
- ↑ John M. Harris: Moeritherioidea. In: Vincent J. Maglio and HBS Cooke (Eds.): Evolution of African Mammals. Harward University Press, 1978, pp. 333-335.
- ↑ Hartmut Thieme and Stefan Veil: New investigations into the Eemzeitlichen elephant hunting ground Lehringen, Ldkr. Verden. Die Kunde 36, 1985, pp. 11-58.
- ^ Alan J. Bryan, Rodolfo M. Casamiquela, José M. Cruxent, Ruth Gruhn and Claudio Ochsenius: An El Jobo Mastodon Kill at Taima-Taima, Venezuela. Science 200, 1978, pp. 1275-1277.
- ↑ Donald K. Grayson and David J. Meltzer: Clovis hunting and large mammal extinction: A critical review of the evidence. Journal of World Prehistory 16 (4), 2002, pp. 313-359.
- ↑ Gerhard Bosinski: The great time of the ice age hunters. Europe between 40,000 and 10,000 BC Chr. Yearbook of the Roman-Germanic Central Museum Mainz 34, 1987, pp 3-139.
- ↑ Michel Lorblanchet: cave painting. A manual. Sigmaringen, 1997.
- ↑ Raman Sukumar: The Human-Elephant Relationship through the Ages: A Brief Macro-Scale History. In: Piers Locke and Jane Buckingham (Eds.): Conflict, Negotiation, and Coexistence: Rethinking Human-Elephant Relations in South Asia. Oxford, 2016, doi: 10.1093 / acprof: oso / 9780199467228.001.0001 .
- ↑ KS Gobush, CTT Edwards, F. Maisel, G. Wittemyer, D. Balfour and RD Taylor: Loxodonta cyclotis. The IUCN Red List of Threatened Species 2021. e.T181007989A181019888 ( [22] ); last accessed on March 26, 2021.
- ↑ KS Gobush, CTT Edwards, D. Balfour, G. Wittemyer, F. Maisel and RD Taylor: Loxodonta africana. The IUCN Red List of Threatened Species 2021. e.T181008073A181022663 ( [23] ); last accessed on March 26, 2021.
- ^ C. Williams, SK Tiwari, VR Goswami, S. de Silva, A. Kumar, N. Baskaran, K. Yoganand and V. Menon: Elephas maximus. The IUCN Red List of Threatened Species 2008. e.T7140A12828813 ( [24] ); last accessed on March 26, 2021.
Web links
- The Paleobiology Database: Proboscidea