Leaf (plant)
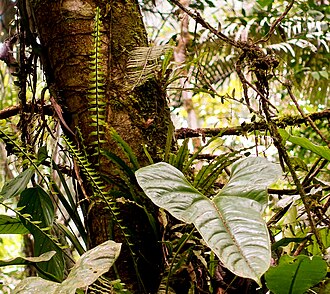
The leaf is one of the three basic organs of the higher plants , along with the stem axis and the root, and is called the phyllomic organ type. Leaves are lateral outgrowths at the nodes (nodes) of the stem axis. The original functions of the leaves are photosynthesis (the build-up of organic matter with the help of light) and transpiration (water evaporation, is important for nutrient absorption and transport).
Leaves only occur in shoot plants , that is, in fern-like plants (Pteridophyta) and seed plants (Spermatophyta). On the other hand, they are absent in mosses and algae , on the thallus of which leaf-like structures can appear, which, however, are only to be regarded as analogies of leaves.
The variety of leaf shapes is enormous. In some cases, leaf organs emerged in the course of evolution that no longer have anything to do with the original function of the leaf (mostly leaf or needle leaf ), namely photosynthesis and transpiration: for example, petals , leaf thorns and leaf tendrils, as well as bud scales (see Metamorphoses of the sheet ).


etymology
The altgerm. Word mhd. , Ahd. Blat belongs to Germanic blatha- ("bloomed, leaf") to idg. Bhel- "swell, bud, bloom" (→ ball "swollen, inflated") and is related to bloom .
anatomy
The anatomical structure described here applies to a bifacial leaf, the most common type of leaf. The elements epidermis, mesophyll and vascular bundles are characteristic of all leaves.
epidermis
The leaf closes on the outside with a closing tissue, the epidermis, which consists of only one layer of cells. The epidermis has a waterproof cuticula layer of wax that prevents unregulated evaporation. The cells of the epidermis generally do not have any chloroplasts (the cell components in which photosynthesis takes place). Exceptions to this are the epidermis of hygro-, helo- and hydrophytes and partly shadow leaves, but especially the guard cells of the stomata ( stomata ), which always contain chloroplasts. The stomata are used to regulate gas exchange, primarily the release of water vapor. According to the distribution of the stomata, a distinction is made between hypostomatic (stomata on the underside of the leaf, most common form), amphistomatic (stomata on both leaf sides) and epistomatic leaves (stomata on the upper side of the leaf, e.g. with floating leaves).
The appendages formed by the epidermis are called hairs ( trichomes ). If subepidermal cell layers are also involved in the formation, one speaks of emergences: Examples are spines or glandular villi.
Mesophyll (leaf parenchyma)
The assimilation tissue is called mesophyll . It is usually divided into the palisade parenchyma located below the upper epidermis and the sponge parenchyma located below . The palisade parenchyma consists of one to three layers of elongated, chloroplast-rich cells perpendicular to the leaf surface. Around 80 percent of all chloroplasts are in the palisade parenchyma, whose main task is photosynthesis. The sponge parenchyma consists of irregularly shaped cells that, due to their shape, form large intercellular spaces. The main task of the sponge parenchyma is to ensure the ventilation of the parenchymal tissue. The cells are relatively poor in chloroplasts.
Vascular bundle
The vascular bundles are often located on the border between palisade and sponge parenchyma in the upper sponge parenchyma. The structure resembles that of the vascular bundles in the stem axis and is mostly collateral . The vascular bundles branch off from the stem axis and pass through the petiole without turning into the blade. This means that the xylem faces the top of the leaf and the phloem faces the underside of the leaf.
Large vascular bundles are often surrounded by an endodermis , here called the bundle sheath . The bundle sheath controls the exchange of substances between the vascular bundle and mesophyll. The vascular bundles end blindly in the mesophyll. The vascular bundle is reduced more and more, i.e. initially the sieve tubes become fewer and fail , then only screws tracheids remain in the xylem part , which ultimately end blind. As a rule, the entire leaf is so dense with vascular bundles that no leaf cell is more than seven cells away from a vascular bundle. The resulting small fields between the vascular bundles are called areoles or intercostal fields.

Thick line: underside of leaf
dotted: palisade parenchyma
black: wooden parts of vascular bundles
A normal bifacial leaf
B inverse bifacial leaf ( wild garlic )
C, D derivative of the unifacial round leaf ( garlic , flutter rush )
E unifacial sword leaf ( irises )
F equifacial flat leaf
G equifacial needle leaf
H equifacial round leaf ( stonecrop )
The function of the vascular bundles is the transport of water and minerals into the leaf (via the xylem) and the removal of photosynthetic products from the leaf (via the phloem).
Strengthening fabric
In the vicinity of the vascular bundles or at the leaf margins there are often strands of sclerenchyme that serve to strengthen the leaf tissue. In some species, subepidermal collenchyme layers serve the same purpose .
Classification according to anatomical aspects
Different leaf types are distinguished according to the position of the palisade parenchyma in the leaf.
- Most of the leaves are built bifacially , i.e. that is, a top and bottom are formed.
- With normal bifacial (= dorsiventral) leaves (A) the palisade parenchyma is on top (= dorsal ), the spongy tissue is below (= ventral ).
- In the case of inverse bifacial leaves (B), the palisade parenchyma is at the bottom (e.g. with wild garlic ).
- With equifacial leaves (F, G), the top and bottom are equally provided with palisade parenchyma, with the sponge parenchyma in between. A typical example is the pine needle leaf (G).
- With unifacial leaves (C, D), the upper and lower sides emerge only from the underside of the leaf system (leaf primordium). They are formally derived from inverse bifacial leaves, in which the upper side of the leaf is reduced. With unifacial leaves, the vascular bundles are arranged in a circle or arc in the leaf cross-section, the phloem points outwards. Petioles are often unifacial, but so are the leaves of many monocots , such as rushes , whose leaves are often stem-like. A special case are the leaves of the irises (E), the unifacial leaf of which became secondarily flat again, but by flattening in the axial direction, so that riding leaves, also called sword leaves, were created.
Morphological structure
A leaf is subdivided into the lower leaf (hypophyll), consisting of the leaf base and the stipules (stipulae), and the upper leaf (epiphyll), which is divided into leaf blade (lamina) and petiolus. Not all parts are formed in all leaves, all parts are subject to manifold variations.
- For a description of the leaf shape in the botanical literature, see
Sub-sheet
Leaf base
The leaf base or the leaf base is the lowest part with which the leaf attaches to the stem axis. The leaf axil is the angle between the stem axis and the leaf branching off from it. It is usually only slightly thickened, but sometimes takes up the entire circumference of the stem axis. In the latter case, one speaks of a leaf surrounding the stem . When the leaves are opposite to each other, the bases of the two leaves are sometimes united (as in the case of the honeysuckle ). Sometimes the leaf base pulls down on both sides as a wing-like strip far down the stem; such stalks are called winged .
In some plant families, such as sweet and sour grasses and umbellifers , the leaf base forms a so-called leaf sheath . It is a more or less wide part, mostly to be found above the base of the leaf, like a sheath surrounding the stem axis. Most of the time the vagina is split, i.e. That is, the edges are free, just superimposed. In contrast, the leaves of the sour grass have closed sheaths or those with no free edges. With many leaves, however, the part of the scabbard is only hinted at or is missing entirely.
Stipules
The stipules (stipules or stipules) are lateral, lobed or leaf-like outgrowths on the leaf base. They are usually small, in many plant species they are missing or are thrown off as the leaves shoot. Depending on the structure of the petiole, there are two types. With a bifacial petiole, lateral stipples appear, which are always paired on the side of the leaf base. This shape is characteristic of dicotyledons. With a unifacial petiole, median (axillary) stipples occur, which only occur in singular and lie in the median in the axilla of the leaf. They are often hood-shaped and occur mainly in monocots.
In some families the stipules are strongly developed, such as in the butterflies (such as the pea ), the rose family and the violet family . They can either be free (e.g. vetch ) or seemingly attached to the petiole ( roses ).
In many trees, such as linden , hornbeam or poplar , the stipules are formed as membranous, non-green scales that fall off while the leaves are unfolding. In the knotweed family, the stipules are transformed into a stipple sheath ( ochrea ), a membranous sheath that encloses the stem in a tubular shape. The ligule of the sweet and sour grasses , which sits at the transition from the leaf sheath to the leaf blade, is also a stipule.
Top sheet
Petiole
The petiolus is the part of the leaf that follows the leaf base and is more or less sharply demarcated from the following part of the leaf by its narrow, stalk-shaped shape. According to the anatomical structure, a distinction is made between bifacial and unifacial petioles. Most monocot and many conifers have no petiole. Leaves without a stem are called sitting. There are also leaves that only consist of the stem, which is then flat and wide and on which the actual leaf surface is completely absent. It is a so-called leaf stalk ( Phyllodium ), z. B. with some acacias . The petiole is usually only formed on leaves. If the petiole is thickened below the leaf blade, this thickening is called the geniculum .
Leaf blade
In most cases, the leaf blade (lamina) forms the main part of the leaf, which is often referred to as the actual leaf. The leaf blade is normally the carrier of the leaf functions photosynthesis and transpiration . The so-called nerve , the course of the vascular bundles, is noticeable on most leaf blades . Large vascular bundles are also called ribs, many leaves have a central rib (1) as an apparent extension of the petiole, from which the side ribs (2) branch off. The vascular bundles are popularly referred to as nerves or veins, both of which are misleading terms, since the vascular bundles have neither an excitation conduction nor a circulatory function.
A distinction is made between three forms of nerve, which also have a systematic meaning. In the monocots mainly parallel nerve occurs. Here the main veins run lengthways and parallel to each other. This results in the mostly smooth leaf margin of the monocot. This is particularly evident with the grasses. The main arteries and the many smaller parallel vascular bundles are, however, connected to one another by small vascular bundles that are mostly visible to the naked eye (transversal anastomoses ). The parallel arrangement of the vascular bundles also leads to a parallel arrangement of the stomata .
Most dicotyledons have a more complex network nerve. This also results in the almost arbitrary shape of the blade.
In ferns and ginkgo , the fork or fan nerve occurs. Here the vascular bundles are dichotomous (fork-shaped) and end blindly at the front end of the leaf.
Especially with the dicotyledons, the leaves appear in a large variety of shapes. The shape and texture of the leaves are therefore important determinants for recognizing the plant species. The texture can e.g. B. be skinny, leathery or succulent (= fleshy). Hair ( trichomes ) are also often important for the surface . The following are important for the shape:
- The structure of the leaf blade: If the blade represents a single, contiguous area of tissue, one speaks of a "simple" leaf. In contrast to this, there are also so-called “compound” sheets. With them, the division of the leaf area has progressed so far that the individual sections appear as completely separate parts. These are called leaflets , regardless of their size . They imitate the shape of simple leaves and are often even provided with a petiole.
- The arrangement of the sections: According to their mutual arrangement, roughly three types can be distinguished:
- pinnate leaves,
- palmate leaves and
- foot-shaped leaves.
In the former, the midrib is called. H. the common stalk on which the individual leaflets usually sit in pairs, leaf spindle (rachis). If the latter closes with a terminal leaflet (terminal leaflet), you have an imparipinnate leaf in front of you. The terminal leaflets can also be shaped like a tendril such. B. in the peas. On the other hand, one speaks of a pair of pinnate leaves if there is no such terminal leaflet. The hand-shaped leaves are differentiated according to the number of leaves as three-fold, five-fold, etc. There are also leaves that are composed several times; this is particularly the case with pinnate leaves. The sections are called plumage here . One speaks here of "double-pinnate" leaves.
Simple, undivided leaf of the quaking aspen
Pinnate leaf of the rose
Palm-shaped leaf of the horse chestnut
Foot-shaped leaf of the snow rose
- The leaf margin (3): The very diverse forms of the leaf margin are described in botany by numerous terms, some of which are listed below: whole-margined, toothed, serrated, curved, notched, etc.
- The shape of the blade or leaflet: Here it is indicated whether the leaf z. B. round, elliptical, linear, kidney-shaped, etc. is.
- The Spreitengrund (4), also called Spreitengrund, describes how the leaf blade merges into the petiole: z. B. heart-shaped, arrow-shaped.
- The spreading apex (5, the tip) can be edged, rounded, pointed, blunt, etc.
- The cross-section of the spread (rolled over, folded, grooved) is also important.
- The three-dimensional shape can also differ from the typical leaf (spherical, tubular, etc.)
A detailed description of the leaf shapes is given in the article Leaf Shape.
evolution
A general distinction is made between two types of leaves, which according to the telom theory were created independently of one another:
- Microphylls are small, often needle-shaped leaves with only one vascular bundle. The mesophyll is usually not very differentiated. Their emergence in evolution is interpreted as a reduction in telomas. The oldest vascular plants , which from the top silur known Urfarngewächse as cooksonia and Rhynia had no leaves. The first microphylls are known from the Protolepidodendrales from the Lower Devonian . Today the microphylls occur in the bear moss plants , the horsetail and the fork-leaf plants . Microphylls are usually small, but the scale trees ( lepidodendrons ) reached a length of around one meter.
- The formation of the macro- or megaphyll is explained by the leveling (planation) and subsequent fusion of the originally three-dimensional telome. Megaphylls appear for the first time in ferns (Polypodiophyta) and are usually called fronds here. The basic type of megaphyll is the pinnate foliage. The other leaf shapes can be derived from it - largely also fossil evidence. In the fossil primofilices (Middle Devon to Lower Perm ), the pinnate sections were still spatially arranged (space fronds), as is still the case with the adder tongue plants (Ophioglossaceae) today .
Growth and lifespan
Leaves arise from a few cells from the outer cell layers (tunica) of the shoot meristem , i.e. exogenous. Lateral outgrowths form in the tunica below the apical meristem . From an initially weak elevation, a small, usually bluntly conical cell tissue hump, called the leaf primordium or the leaf primordium, emerges.
The dorso-ventral organization of the leaf occurs through a signal from the shoot meristem. If this signal is not received - for example when the leaf primordium is separated from the shoot meristem - a radially symmetrical structure with ventral differentiations is formed. Dorsal development is promoted by a group of genes that includes the genes PHABULOSA (PHB), PHAVOLUTA (PHV) and REVOLUTA (REV), which code for transcription factors. These genes are already formed in the peripheral zone of the shoot meristem, i.e. before the leaf primordium is formed. As soon as the primordium is recognizable, the expression of the genes is restricted to the dorsal side. On the ventral side of the leaf primordium, genes of the YABBY (YAB) gene family (transcription factors with zinc finger domain ) and genes of the KANADI (KAN) gene family (GARP transcription factors) are expressed. These genes are also initially expressed uniformly throughout the leaf primordium. Leaf primordia therefore initially express dorsalizing (PHB) as well as ventralizing (YAB, KAN) genes. A signal from the meristem activates PHB transcription factors, depending on the location, these repress the YAB and KAN genes and maintain their own expression. This creates the dorso-ventral structure. The proximo-distal leaf development also seems to be promoted by this.
The leaf hump develops from the leaf anlage, which is differentiated by a constriction into a broad, proximal section, the lower leaf, and a narrow, distal section, the upper leaf.
The growth takes place only for a short time with the tip (akroplast). The tip stops growing very early, growth takes place through basal or intercalar meristems (basiplastes or intercalar growth). The leaf blade (lamina) mostly arises from basiplastic growth, the leaf stalk (petiolus) and the spreading of the grasses from intercalary growth. The ferns are an exception, their growth acroplastically by means of a vertex cell or a vertex edge (made up of several cells).
In the further course of growth, cell division and cell elongation processes do not occur evenly in the entire leaf body, but only within meristematic (or division) active zones. Whether, at what point in time and how intensively these zones are active is genetically determined and leads to a characteristic leaf shape.
Leaves usually only have a limited lifespan; only in a few perennial species do the leaves remain for the entire lifespan of the plant (e.g. Welwitschia ). According to the lifespan, a distinction is made between evergreen leaves (live at least two growing seasons ), evergreen (hibernating green), deciduous (only one growing season long) and decrepit leaves (fall off very soon, e.g. sepals of the poppy ).
The leaf fall occurs through the formation of a separate separation zone (abscission zone) at the transition from the stem axis to the leaf (see abscission ).
Color and color change
The absorption spectra of chlorophylls dissolved in solvents always have two distinct absorption maxima, one between 600 and 800 nm and one around 400 nm, called the Soret band . Figure 1 shows these absorption maxima for chlorophyll a and b . The green gap is the reason why leaves - these contain chlorophyll a and b - are green: Together, chlorophyll a and b absorb mainly in the blue spectral range (400–500 nm) and in the red spectral range (600–700 nm). In the green area, on the other hand, there is no absorption, so this portion of sunlight is scattered, which makes leaves appear green.
The discoloration of the leaves before the autumn leaves fall is particularly noticeable. This comes about because the green nitrogen- rich photosynthesis pigment chlorophyll is broken down in the cells and the nitrogen is shifted to the axis of the shoot. The yellow carotenes , which were previously covered by green, remain in the leaf and, in some species, the red anthocyanins , which are responsible for the colorful autumn colors. In some plants the anthocyanins generally dominate over the green chlorophyll, e.g. B. in the copper beech . Other leaves are mottled green-white, variegated . These forms are very popular in the ornamental plant sector.
Leaf sequence
The sequence of differently shaped leaves on a plant is called a leaf sequence or heteroblastic row . A typical leaf sequence is cotyledons - primary leaves - deciduous leaves - petals. In between, bracts and lower leaves can be interposed. With the ferns , the shape of the leaves changes only slightly on the entire shoot and on all branches, one of the few exceptions is the antler ferns with their sterile coat leaves. In the course of the leaf sequence, differently shaped leaves appear in some species, this phenomenon is referred to in the literature as leaf dimorphism or heterophylly ; a well-known example of this is found in ivy .
Cotyledons
The cotyledons of the seed plants are the first leaves created in the embryo and are already recognizable in the seed . They are usually much simpler than the following sheets. The number of cotyledons also serves as an important systematic characteristic. The class of the monocot (Liliopsida) was named after its single cotyledon (monocotyledon). Until a few years ago, they were compared to the class of the dicotyledonous (Magnoliopsida) (dicotyledon), which is now divided into two classes. The Nacktsamer usually have several cotyledons and are therefore called polykotyl. Depending on whether the cotyledons break through the surface of the earth during germination, one speaks of epigeic (above the surface of the earth, most of our cultivated plants) or hypogean (below the surface, e.g. of the peanut ) germination .
Primary leaves
In many plants, the cotyledons are followed by foliage leaves, which are also designed even more simply than those formed later. These are the so-called primary leaves.
Foliage leaves
These are the leaves that make up the majority of the leaf mass in most plants and whose main job is photosynthesis and transpiration. The structure described above in the sections Anatomy and Morphological Structure applies to them in particular .
Cover sheets
The first leaves, often differentiated from the following leaves by their shape or position, on the side axes, e.g. B. on flower stalks. In monocots usually 1 in dicots usually 2. See also: floral diagram .
Petals
Considered morphologically, a is blossom , a short shoot, the seated at this short shoot sheets are formed into the petals: The bloom are either differently configured as sepals (sepals) and petals (petals) or uniformly as tepals (Tepalen); inwardly follow the dust and the carpels .
Lower leaves
Lower leaves (cataphylls) are usually small and simply designed, often scale-shaped. In many cases, only the lower sheet is formed. Mostly they are not green. On the stem axis they are below the leaves, hence the name. They are either at the beginning of the basal shoot or the side shoot, in woody plants, lower leaves are often found as bud scales at the lower end of the annual shoot (but not in all woody plants the bud scales are lower leaves). Here, the deciduous and lower leaf regions alternate periodically. Lower leaves are also found on rhizomes, underground runners. The onion scales of onions are also mostly lower leaves.
Bracts
As bracts is known in plants bracts , which carry a single flower, an inflorescence or part of inflorescence in its axil. A bract of a single flower is called a wrapper. Bracts are called bracts, which usually surround an inflorescence in several groups. Their totality is called the envelope (involucum). The leaves following the bract on the branch are called bracteoles.
Often the bracts differ from the normal leaves, e.g. B. by a conspicuous color. They are only distinguished from the lower leaves by their position in the stem. Often there are transitional forms (transitional leaves) between the deciduous and bracts.
Cover sheets
A bract, also called bractea, is a bract that often has a partial inflorescence or individual flowers in its armpit. See also: support sheet .
Leaf position
Leaves are arranged on the stem axis in a regular, species-specific manner. One or more leaves can be found at each node of the stem axis, there are four basic types of leaf position:
- With the two-line or distal leaf position there is only one leaf at each node, leaves of consecutive nodes are shifted by 180 °, so that two longitudinal rows of leaves result on the stem axis. Representatives are many monocotyledonous plants and butterflies .
- If the leaf position is alternate, there is also only one leaf at each node, but the angle between two leaves is different from 180 °, the leaves stand along a spiral line. This arrangement is characteristic of dicotyledonous plants.
- When the leaves are opposite to each other, there are two leaves at each node. In the decussed or cross-opposed leaf position, successive leaf pairs are each rotated by 90 degrees, i.e. they are at right angles to one another. There are four longitudinal lines. Representatives are mint family , carnation family and olive family .
- If the leaves are lively, there are three or more leaves at each node, with the leaves of the next younger node standing on gaps. Representatives are e.g. B. the red plants ( woodruff ).
Metamorphoses of the leaves
As with the root and the stem axis , the leaves are also often modified by metamorphoses in order to either fulfill their original function adapted to certain environmental conditions or to take on other functions at all.
Sun and shadow leaves
Sun leaves
Sun leaves, d. H. Leaves that are exposed to full sunlight often form a multi-layered, small-cell palisade parenchyma. The intercellular areas in the sponge parenchyma are weak.
Shadow leaves
Shadow leaves often have a reduced palisade parenchyma, the leaves consist of a few cell layers, the cells are large and have few chloroplasts. The intercellular system is spacious, the palisade cells are conical. The water lines are often reduced.
Sun and shade leaves appear on a plant especially in trees (e.g. European beech ). Sun leaves also lead to the xeromorphic leaves, shadow leaves to the hygromorphic leaves.
Xeromorphic leaves

E = convex, papilla-like epidermal cells
H = living, epidermal leaf hairs
I = large intercellular ones
S = raised stomata
Many plants in arid locations reduce their leaves completely or turn them into thorns, such as B. the cactus plants . This significantly reduces the surface of the plant and thus also the transpiration.
However, numerous xerophytes keep their leaves, but their structure is strongly modified in the direction of reducing perspiration. Xeromorphic leaves are usually rough leathery ( hard deciduous trees such as laurel , myrtle and olive tree ). The stomata are sunk deep into the leaf surface, the resulting depressions (crypts) are provided with hairs that further hinder the air convection . The substomatic intercellular space can be sealed with wax. In many cases, the leaves are rolled up when it is dry, thus further enclosing the stomata (e.g. Stipa capillata ).
The epidermis has a thickened cuticle with heavy wax deposits. Often the leaves are densely covered with dead hair. This leads to less air exchange and a significantly more humid microclimate directly on the leaf surface.
Xeromorphic leaves are often constructed equifacially. The coniferous leaf also has a typically xeromorphic structure, as the conifers are often exposed to severe frost drought in winter .
However, since a decrease in transpiration can lead to overheating, some plants place their leaves perpendicular to the sun, such as some Australian eucalyptus , which form "shadowless forests".
Hygromorphic leaves
Hygromorphic leaves are an adaptation to always moist locations. In addition to the characteristics of the shadow leaves, they have large, thin-walled epidermal cells that often carry chloroplasts and have only a thin cuticle. The stomata are often raised above the epidermis to facilitate perspiration. Hygrophytes (hygromorphic leaves), which mostly live in tropical areas, have the difficulty of releasing water due to the high humidity in order to absorb new (and thus also minerals). In contrast to xerophytes, who have their stoma turned inwards in order to transpire as little water as possible, hygrophytes have their stomata turned outwards. Sometimes there is also active water excretion ( guttation ) via the stomata, then water droplets hang on the protruding stoma, blown away by the wind or falling to the ground by animals. Guttation is the excretion of water that is no longer in gaseous form as in transpiration.
Needle leaf
The coniferous leaves of most coniferous plants (Pinophyta) are largely an adaptation to drought (xeromorphism). The mostly evergreen trees are exposed to frost drought in winter, i. In other words, the plant cannot absorb water through the frozen ground and must therefore counteract water loss through the leaves: the needles have a small surface, a thick cuticle and the stomata are sunk into the epidermis.
Needle leaves have other characteristic features - most leaves are constructed equifacially, sponge and palisade parenchyma are not clearly separated. In addition, there are some bifacial needle leaves, e.g. B. that of the silver fir ( Abies alba ), which have a different differentiation according to the top (palisade parenchyma) and underside (sponge parenchyma and white wax layer with stomata) similar to a leaf. The surface of the mesophyll cells of some Pinus species is enlarged by ridge-shaped wall indentations (arm palisade parenchyma). Between this parenchyma and the epidermis lies a sclerotic (dead) strengthening tissue, the so-called hypodermis, made of extremely thick cell walls. The epidermal cells are usually almost completely filled with secondary and tertiary wall thickenings and only have narrow connecting channels to the neighboring cell. Resin canals usually run lengthways in the mesophyll . The one or two unbranched vascular bundles are surrounded by a common vascular bundle sheath , the endodermis . The transport of substances between the vascular bundle and the mesophyll takes place through a special transfusion tissue ( Strasburger cells) and through short dead tracheids . The vascular bundle consists of xylem and phloem, as in the stem axis and the root. In between there is a thin layer of cambium, which is used to form new sieve cells in perennial needle leaves (sieve cells are very short-lived, see also bast ). Xylem is hardly ever formed again.
Further metamorphoses
Thorns
Thorns are used by plants to ward off animals. Leaf spines are single or multi-pointed transformations of leaves or leaf parts made of sklerenchymatic tissue. The thorns of the barberry are transformations of the entire leaf, they occur on the long shoots. Sub-leaf thorns (stipular thorns) always occur in pairs and are z. B. to be found in the Robinia .
Tendrils
Tendrils are used to hold the plant on supports. They can be derived from all basic organs of the leaf. In the pea , for example, the terminal leaflets of the pinnate leaves are transformed, while in the flat pea the tendril is formed by the leaf blade, while the stipules take over photosynthesis. With some plants, the leaf stalk is used for tendrils - these wind around the support (e.g. pitcher plants or bindweed ).
Storage organs
In arid locations, leaves are often transformed into organs that store water. Such leaves are often built equifacially. Either the epidermis and subepidermal tissue can be involved in water storage, or it takes place in the mesophyll .
Water-storing cells always have very large sap vacuoles . Succulent leaves have a thick, fleshy, juicy appearance. Plants with such leaves are called leaf succulents. As with shoot succulence, leaf succulence is often associated with the CAM mechanism . Typical leaf succulents are the agaves or the houseleek species.
Also onions are made of leaves and are used to store. An onion is an extremely compressed, subterranean sprout axis, on which thick-fleshed scale leaves lie on top of each other. These scale leaves are lower leaves or emerge from the base of dead leaves and serve to store reserve materials . In unfavorable seasons, the plant survives as an onion. At the beginning of a new vegetation period, axillary buds sprout between the scale leaves to form new vegetation bodies and use up the stored reserve materials. In addition to the kitchen onion , tulips , lilies and daffodils are other examples of onion plants. Onions are only found in monocots .
Phyllodes
When the petiole is broadened and takes over the function of the leaf blade, one speaks of phyllodes. In this case, the leaf blade is usually greatly reduced. Examples can be found in the acacia , where there are often several transition stages from the typical pinnate leaves to spiderless phyllodes on a plant.
Branch of barberry from below. The leafy short shoots sit in the armpits of the thorns.
Succulent leaves of the roof houseleek
The onion is made up of leaves
Leaves of carnivorous plants

In many carnivorous plants , the leaves have been transformed into organs with which prey is captured and absorbed; depending on the species, they are referred to as either sticky, hinged or pitfall traps. In some species of plants, the leaves are also capable of, sometimes very rapid, movements ( sundew plants , water hoses ). All carnivorous plants are able to absorb the dissolved nutrients of the prey with the surface of their traps, the carnivorous plants in the strict sense are additionally provided with glands on the surface of the traps, through which they secrete enzymes that dissolve the prey.
Leaves of the epiphytes
Epiphytes grow on trees or other plants and are therefore dependent solely on precipitation and humidity (fog) for their water and nutrient supply. Many epiphytes form funnel-shaped rosettes with their leaves, in which rainwater collects. In the funnels of the nest ferns , e.g. B. ( Asplenium nidus ), even humus accumulates over time, as well as in the coat leaves of the antler ferns ( Platycerium ). The genus Dischidia ( Asclepiadaceae ) forms tubular leaves in which ant colonies settle and drag in the earth. Advent roots grow into these "flower pots" . The same applies to many lithophytes .
Most (especially epiphytic) bromeliads , for example Tillandsia species, form special absorption hairs (suction scales) with the help of which they can absorb water through the leaf.
Exchange of substances via the surface
The most important tasks of the leaves are photosynthesis , which is accompanied by the exchange of oxygen and carbon dioxide with the ambient air, and transpiration , i.e. the release of water into the atmosphere. These processes are described in more detail in the respective articles. There are also a number of other substances that the leaves can absorb or release into the air.
Exchange via the stomata
Above all, gaseous and very volatile substances are absorbed through the stomata. The most important are sulfur dioxide, ammonia and nitrogen dioxide. Ammonia can provide 10 to 20 percent of plant nitrogen in areas with intensive animal husbandry. The uptake of ammonia through the stomata increases linearly with the external concentration. The same is true for nitrogen dioxide . High concentrations of sulfur dioxide damage photosynthesis, while low concentrations can lead to better growth, especially if there is a lack of sulfur in the soil.
However, plants can also lose nutrients through their leaves. For example, the loss of nitrogen through the stomatal release of ammonia was calculated to be 15 kg nitrogen per hectare for rice and seven kg nitrogen per hectare for wheat , which in the latter case corresponded to 20 percent of the fertilizer application. When exposed to high levels of sulfur dioxide, leaves give off hydrogen sulfide . This is interpreted as a detoxification mechanism. But even plants without sulfur dioxide pollution emit volatile sulfur compounds; values of two to three kilograms of sulfur per hectare and year have been calculated for oats and rape . Plants with a high selenium content also give off volatile selenium compounds such as dimethyl selenium .
Absorption of dissolved substances, foliar fertilization
In terrestrial plants, the uptake of dissolved substances via the leaves is severely restricted by the cuticle of the epidermis. Low-molecular compounds such as sugar as well as minerals and water can pass through the cuticle through hydrophilic pores. These pores have a diameter of one nanometer . B. Urea (diameter 0.44 nanometers) easily pass. The pores are negatively charged so that cations can pass more easily than anions . This z. B. Ammonium absorbed faster than nitrate . Pores occur particularly frequently in the cell wall of the guard cells, which explains the frequently observed positive correlation between the number of stomata and the uptake of nutrients from liquid fertilizer applied.
The further uptake into the cell proceeds in the same way as with the nutrient uptake of the roots via the apoplast. With the same external nutrient concentration, however, the uptake rate is significantly lower for leaves than for the roots due to the additional bottleneck in the cuticle. In contrast to roots, the uptake of ions by leaves is promoted by light. The rate of absorption is also dependent on the internal nutrient concentration, i.e. that is, absorption is faster if there is a lack of nutrients.
In natural ecosystems, the uptake of nutrients is only important for nitrogen and sulfur.
Foliar fertilization generally supplies the plants with nutrients more quickly than conventional soil fertilization. Therefore, despite some disadvantages, it is used in many areas.
The disadvantages include:
- Rolling off the hydrophobic leaf surface
- Wash off by rain
- Certain nutrients such as calcium can no longer be transported from the leaves to other parts of the plant.
- With foliar fertilization, only a limited amount of nutrients can be applied (the exception is urea).
- It can cause damage to the leaf: necrosis and burns .
However, under certain conditions, foliar fertilization is of great practical importance:
- Nutrient deficiency in the soil: On limestone soils, which immobilize iron , foliar fertilization with iron can protect against chlorosis . The same goes for manganese deficiency. In the case of fruit trees, foliar fertilization with boron can protect against a boron deficiency in autumn.
- Dry topsoil : In semi-arid areas, the availability of nutrients is often drastically reduced due to the desiccation of the topsoil. In such cases, foliar fertilization is more effective than soil fertilization.
- Many plants have reduced root activity during seed filling. Here, too, foliar fertilization can lead to higher nutrient levels and also to crop yields.
Irrigation with salty water can lead to a greatly increased absorption of chloride and sodium . This effect is stronger with this type of irrigation than with drip irrigation.
Leaching
The loss of organic and inorganic substances through liquids, especially rain and irrigation, is usually referred to with the English term leaching (licking, washing out). There are four types:
- Active excretion of solutions, e.g. B. the excretion of salt by salt glands in halophytes .
- Excretion of inorganic solutions at leaf tips and margins by pressure from the roots: Guttation .
- Leaching from injured leaf areas.
- Leaching from the apoplast of intact leaves.
The last two species are of major ecological importance. The loss is higher in old leaves and under stress (drought, high temperature, ozone ). A low rain pH ( acid rain ) also increases leaching. The cations of the leaf are replaced by protons like in an ion exchanger .
With the exception of nitrogen and sulfur, leaching predominates in natural ecosystems. The loss is particularly high in areas with heavy rainfall. The following annual values were calculated for tropical rainforests (in kilograms per hectare): potassium 100–200, nitrogen 12–60, magnesium 18–45, calcium 25–29, and phosphorus 4–10. In temperate latitudes, the high leaching rate of calcium and manganese is noticeable compared to the internal leaf content . These elements are not phloem-mobile , i. i.e., they accumulate in the leaves. The strong leaching is interpreted as a strategy of the plants to avoid too high concentrations.
In addition to minerals, larger amounts of organic compounds can also be lost through leaching. For forests of the temperate latitudes, values of 25 to 60 kilograms of carbon per hectare and year have been calculated, for tropical forests the amount is estimated at several hundred kilograms.
The leaf as a living space
As physiologically very active plant parts (photosynthesis), leaves usually contain a lot of nutrients and are therefore a very important source of food for a large number of animal species. However, several animal groups also use the leaves as a habitat. These include leaf miners such as B. the horse chestnut leaf miner . These are insects whose larvae eat burrows inside the leaves. Further examples are leaf rollers (family Attelabidae), whose females roll up leaves and lay their eggs in them, so that the larvae are protected, and gall wasps , which, when they lay eggs , trigger the formation of so-called galls , growths of the plant tissue, on which the larvae feed.
Leaves are also attacked by a wide variety of fungi, such as powdery mildew , smut fungi and rust fungi , which can cause great damage in agricultural and horticultural crops. Endophytic fungi often live in leaves and do not cause any noticeable damage to the plant.
In turn, other plants can live on leaves; this life form is called epiphyllium . Epiphyllus mosses and lichens are particularly common in tropical rainforests and cloud forests .
The habitat that the immediate leaf surface provides for other organisms is also known as the phyllosphere .
literature
- Wolfram Braune, Alfred Leman, Hans Taubert: Plant anatomical internship. 1. As an introduction to the anatomy of the vegetation organs of the seed plants . 6th edition. Gustav Fischer, Jena 1991, ISBN 3-334-60352-0 , p. 176-220 .
- Manfred A. Fischer, Wolfgang Adler, Karl Oswald: Excursion flora for Austria, Liechtenstein and South Tyrol . 2nd, improved and enlarged edition. Province of Upper Austria, Biology Center of the Upper Austrian State Museums, Linz 2005, ISBN 3-85474-140-5 , p. 72-84 .
- Stefan Klotz, Dieter Uhl , Christopher Traiser, Volker Mosbrugger: Physiognomic adaptations of leaves to environmental conditions . in: Naturwissenschaftliche Rundschau. Stuttgart 58.2005,11, pp. 581-586, ISSN 0028-1050
- Ulrich Lüttge, Manfred Kluge, Gabriela Bauer: Botany. A basic textbook . VCH, Weinheim u. a. 1988, ISBN 3-527-26119-2 .
- Klaus Napp-Zinn: Anatomy of the sheet. T II. Leaf anatomy of angiosperms. B: Experimental and ecological anatomy of the angiosperm leaf. in: Handbook of Plant Anatomy. Vol. 8 Part 2 B. Borntraeger, Stuttgart 1988 (2nd delivery), ISBN 3-443-14015-7 .
- Schmeil, Fitschen : Flora of Germany and neighboring countries . Quelle & Meyer, Heidelberg / Wiesbaden 89 1993, ISBN 3-494-01210-5
- Peter Sitte , Elmar Weiler , Joachim W. Kadereit , Andreas Bresinsky , Christian Körner : Textbook of botany for universities . Founded by Eduard Strasburger . 35th edition. Spektrum Akademischer Verlag, Heidelberg 2002, ISBN 3-8274-1010-X .
Web links
- Structure of a typical leaf
- Blade cross section (overview)
- Sheet pictures from the picture archive of the University of Basel
- Leaf nerve images from the image archive of the University of Basel
- Leaf shapes and leaf positions
- Examples of fossil, pliocene leaves
Individual evidence
- ^ The dictionary of origin (= Der Duden in twelve volumes . Volume 7 ). Reprint of the 2nd edition. Dudenverlag, Mannheim 1997 ( p. 85 ). See also Friedrich Kluge : Etymological dictionary of the German language . 7th edition. Trübner, Strasbourg 1910 ( p. 58 ).
- ↑ cf. Lexicon of Biology. Vol. 3. Spectrum Akademischer Verlag, Heidelberg 2000, p. 1. ISBN 3-8274-0328-6 ; Custom and a., 2002, pp. 717-750.
- ^ Wilhelm Seyffert (Ed.): Textbook of Genetics . Spektrum Akademischer Verlag, Heidelberg 2003, ISBN 3-8274-1022-3 , pp. 712 f .
- ^ W. Rothmaler: Exkursionsflora von Deutschland; Berlin 1966
- ^ Gerhard Wagenitz : Dictionary of Botany. The terms in their historical context. 2nd, expanded edition. Spektrum Akademischer Verlag, Heidelberg / Berlin 2003, ISBN 3-8274-1398-2 , p. 331.
- ↑ The section follows Horst Marschner : Mineral nutrition of higher plants . 2nd Edition. Academic Press, London 1995, ISBN 0-12-473543-6 , pp. 116-130 .